Abstract
BACKGROUND/OBJECTIVES
This study was performed to investigate the in vitro antioxidant and cytoprotective effects of fermented sesame sauce (FSeS) against hydrogen peroxide (H2O2)-induced oxidative damage in renal proximal tubule LLC-PK1 cells.
MATERIALS/METHODS
1,1-diphenyl-2-picrylhydrazyl (DPPH), hydroxyl radical (•OH), and H2O2 scavenging assay was used to evaluate the in vitro antioxidant activity of FSeS. To investigate the cytoprotective effect of FSeS against H2O2-induced oxidative damage in LLC-PK1 cells, the cellular levels of reactive oxygen species (ROS), lipid peroxidation, and endogenous antioxidant enzymes including catalase (CAT), superoxide dismutase (SOD), and glutathione peroxidase (GSH-px) were measured.
RESULTS
The ability of FSeS to scavenge DPPH, •OH and H2O2 was greater than that of FSS and AHSS. FSeS also significantly inhibited H2O2-induced (500 µM) oxidative damage in the LLC-PK1 cells compared to FSS and AHSS (P < 0.05). Following treatment with 100 µg/mL of FSeS and FSS to prevent H2O2-induced oxidation, cell viability increased from 56.7% (control) to 83.7% and 75.6%, respectively. However, AHSS was not able to reduce H2O2-induced cell damage (viability of the AHSS-treated cells was 54.6%). FSeS more effectively suppressed H2O2-induced ROS generation and lipid peroxidation compared to FSS and AHSS (P < 0.05). Compared to the other sauces, FSeS also significantly increased cellular CAT, SOD, and GSH-px activities and mRNA expression (P < 0.05).
Soy sauce is a traditional fermented soy product with a salty taste that is widely consumed in Asia. It is rich in free amino acids, reducing sugars, and organic acids, and contains low levels of isoflavones. Fermented soy sauce (FSS) was found to possess high levels of antioxidant activity along with the ability to scavenge free radicals in vitro and in vivo [1,2,3]. Other studies reported that soy sauce has anticarcinogenic [4,5,6,7] and antimutagenic [8,9] properties. Fermented sesame sauce (FSeS), a new type of liquid condiment, is prepared in a manner similar to that of FSS and is produced in South Korea. Sesame, the raw material used to manufacturer FSeS, is rich in high levels of lignans (such as sesamin, sesamolin, sesaminol, and sesame) that have antioxidant, anticarcinogen, hypolipidemic, antihypertensive, and immunoregulatory activities in humans and experimental animals [10]. In contrast to other fermented sauces, acid-hydrolyzed soy sauce (AHSS) is prepared by hydrolyzing defatted soybeans with hydrochloric acid. A couple studies reported that AHSS contains 1, 3-dichloropropan-2-ol (1, 3-DCP) and 3-chloropropane-1, 2-diol (3-MCPD), which exert potential mutagenic effects in humans and rodents [11,12].
Reactive oxygen species(ROS) are produced in mammalian cells during energy production in mitochondria by reducing oxygen during aerobic respiration, which including superoxide (O2-), peroxide (O2-2), hydroxyl radical (·OH), hydrogen peroxide (H2O2), and peroxynitrite (ONOO-) play a key role in the oxidative stress induced damage in renal epithelial cells is thought to be important for the development of renal disease [13,14]. Accumulation of the ROS was reduced the activity of endogenous antioxidant enzymes including catalase (CAT), superoxide dismutase (SOD), glutathione peroxidase (GSH-px), and depletion of GSH, as well as caused the oxidative damage in renal cells [14]. In particular, hydrogen peroxide (H2O2), a reactive oxygen metabolite formed by either enzyme-catalyzed or spontaneous dismutation of superoxide anions, has been implicated in the pathogenesis of renal injury [13,14,15]. H2O2- induced renal cell damage and tissue necrosis is associated with lipid peroxidation in renal cell membranes [13,14,15,16,17].
Dietary intake of foods rich in antioxidants, such as phenolic compounds and vitamin E, is able to increase the levels of protective antioxidants in the body and improve the human antioxidant defense system to reduce the risk of renal dysfunction induced by ROS [18,19]. Many studies have reported that FSS has various beneficial properties [4,5,6,7,8,9]. However, the beneficial activities of FSeS have not been studied. This study was designed to investigate the ability of FSeS to provide protection against H2O2-induced oxidative damage compared to FSS and AHSS in LLC-PK1 cells, an epithelial cell line derived from pig kidney that has retained characteristics of the proximal tubular epithelium and is wildly used as a cell model for studying ROS-induced renal damage [17]. Additionally, the mechanisms underlying these effects were elucidated.
FSeS, FSS, and AHSS were provided by Daesang Food Co., Ltd. (Echeon, South Korea). Sauce samples (200 mL) were freeze-dried at -80℃, and underwent extraction three times with a 20-fold volume of methanol at room temperature in the dark for 24 h. The methanol extracts were combined, filtered through filter paper (Whatman International, Maidstone, Kent, UK), and concentrated under a vacuum at 50℃ in a rotary evaporator (Büchi RE 111 rotavapor, Flawil, Switzerland). The sauce extracts were finally dissolved in dimethyl sulfoxide (DMSO) and stored at -4℃ until further analysis.
3-(4, 5-dimethylthiazol-2-yl)-2, 5-diphenyl tetrazolium bromide (MTT), 2', 7'-dichlorodihydrofluorescin diacetate (DCFH-DA), 1,1-diphenyl-2-picrylhydrazyl (DPPH), and deoxyribose were purchased from Sigma-Aldrich Chemical Co. (St. Louis, MO, USA). Dulbecco's modified Eagle's medium (DMEM), fetal bovine serum (FBS), penicillin-streptomycin solution, and 0.05% trypsin-0.53 mM EDTA were purchased from Grand Island Biological Company (Grand Island, NY, USA). TRIzol reagent, oligodT18 primer, murine maloney leukemia virus (MMLV) reverse transcriptase, RNase inhibitor, reverse transcriptase buffer, dNTPs, ethidium bromide (EtBr), and agarose were purchased from Invitrogen Life Technologies (Carlsbad, CA, USA). Chemicals used were of standard analytical grade.
DPPH radical scavenging activity was estimated as previously described by Hatano et al. [20]. Sauce extracts (10, 50, and 100 µg/mL) were added to a methanolic solution (0.1 mL) of DPPH radicals (final concentration, 150 µM). The mixture was shaken vigorously and allowed to stand at 37℃ for 30 min. Absorbance of the reaction mixture was then measured at 517 nm using a UV-2401PC spectrophotometer (Shimadzu, Kyoto, Japan).
•OH scavenging activity was evaluated as previously described by Halliwell et al. [21]. A reaction mixture (1.4 mL) containing 0.2 mL of the sauce extracts (10, 50, and 100 µg/mL), deoxyribose (6 mM), H2O2 (3 mM), KH2PO4-K2HPO4 buffer (20 mM, pH 7.4), FeCl3 (400 µM), EDTA (400 µM), and ascorbic acid (400 µM) was incubated at 37℃ for 1 h. The extent of deoxyribose degradation was measured using a thiobarbituric acid (TBA) assay. One mL of 1% TBA and 1 mL of 2.8% trichloroacetic acid (TCA) were added to the mixture and heated at 90℃ for 30 min. Absorbance of the reaction mixture was measured at 532 nm using a UV-2401PC spectrophotometer.
H2O2 scavenging activity was estimated according to the method of Ruch et al. [22]. Sauce extract (20 µL) was dissolved in 200 µL of phosphate buffer (0.1 M, pH7.4) and mixed with 600 µL of H2O2 solution (40 mM). The absorbance of the reaction mixture was measured at 230 nm using a UV-2401PC spectrophotometer.
LLC-PK1 porcine renal tubules cells were obtained from the American Type Culture Collection (ATCC; Manassas, VA, USA). The cells were routinely maintained in DMEM medium supplemented with 10% (v/v) FBS, and 1% penicillin-streptomycin in a humidified CO2 incubator (model 3154; Forma Scientific, Inc., Marietta, OH, USA) with 5% CO2 at 37℃.
Cell viability was measured using an MTT assay. LLC-PK1 cells were seeded in 96well plates (Nunc, Rochester, NY, USA) at a density of 1 × 104 cells/well. Following a 24h incubation, the cells were first treated with the sauce samples(10 and 100 µ g/mL) for 24 h, and then exposed to H2O2 (500 µM) for an additional 4 h. Next, 100 µL MTT reagent (0.5 mg/mL) was added to each well and the cells were incubated in a humidified incubator at 37℃ to allow the MTT to be metabolized. After 4 h, 100 µL DMSO was added to each well to dissolve the formazan crystals. Absorbance of the samples was measured at a wavelength of 540 nm using a microplate reader (model 680; Bio-Rad, Hercules, CA, USA).
Lipid peroxidation was quantitated using a TBA reactive substance (TBARS) assay as previously described [23]. Briefly, the cultured cells were washed with cooled phosphate-buffered saline (PBS), scraped into TCA (2.8%, w/v), and sonicated 3 times for 10 second intervals at 40 V setting over ice. Concentration of the total cell proteins was determined using a bicinchoninic acid (BCA) assay. The suspension(200 µL) was mixed with 1 mL TBA (0.67%, w/v) and 1 mL TCA (25%, w/v), heated (30 min at 95℃), and centrifuged (3,000 × g for 10 min at 4℃). The TBA reacted with the products of lipid oxidative degradation, yielding red complexes. Absorbance was measured at 532 nm using a UV-2401PC spectrophotometer (Shimadzu, Kyoto, Japan).
Intracellular ROS levels were measured using the fluorescent probe DCFH-DA. The treated LLC-PK1 cells were washed with calcium- and magnesium-free PBS, and incubated in DCFH-DA (20 µM) containing serum- and phenol red-free DMEM at 37℃ for 30 min. The medium was then removed and the cells were washed twice with PBS. Fluorescence was measured using a FLUOstar OPTIMA fluorescence plate reader (BMG Labtech, Ortenberg, Germany); excitation was read at 485 nm and emission was detected at 535 nm. Relative ROS production was expressed as the percentage of fluorescence for the treated samples over fluorescence for the appropriate controls: (fluorescencetreatment / fluorescencecontrol) × 100.
LLC-PK1 cells grown in a 10cm cell culture dish (Nunc, Rochester, NY, USA) were incubated with FSeS, FSS, and AHSS for 24 h, and then treated with H2O2 (500 µM) for 4 h at 37℃. The cells were washed with PBS, removed by scraping, and centrifuged (3,000 × g for 10 min at 4℃). The resulting cell pellet was stored at -80℃. The pellets were then thawed, resuspended in 300 µL cold lysis buffer (PBS and 1 mM EDTA), homogenized, and centrifuged (12,000 × g for 10 min at 4℃). Protein contents were determined using a Bio-Rad protein assay kit according to the manufacturer's instructions. Catalase(CAT) activity (U/mg protein) in the supernatant was assessed according to the method described by Nelson and Kiesow [24] in which disappearance of the H2O2 substrate was measured using a UV-2401PC spectrophotometer at 260 nm. Superoxide dismutase (SOD) activity (U/mg protein) was measured using a modified auto-oxidation pyrogallol method [25]. One unit of SOD activity was defined as the amount of enzyme that inhibited the auto-oxidation rate of pyrogallol by 50%. Glutathione peroxidase (GSH-px) activity (U/mg protein) was evaluated as previously described by Hafemen et al. [26].
mRNA expression of CAT, SOD, GSH-px, and glutathione S-transferase (GST) in the cells was measured with an RT-PCR assay. Total RNA was isolated with TRIzol reagent according to the manufacturer's recommendations. Chloroform (200 µL) was added and the solution was centrifuged at 12,000 × g for 15 min at 25℃. Isopropanol was then added to the supernatant at a 1:1 (v:v) ratio and the RNA was pelleted by centrifugation (12,000 × g for 15 min at 4℃). After washing the pellet with 70% ethanol, the RNA was solubilized in diethyl pyrocarbonate-treated RNase-free water and quantified by measuring the absorbance at 260 nm using a UV-2401PC spectrophotometer. Equal amounts of RNA (1 µg) were reverse transcribed in a AccuPower PCR PreMix(Bioneer, Daejeon, South Korea) containing 1 × reverse transcriptase buffer, 1 mM dNTPs, 500 ng of oligodT18 primers, 140 U of MMLV reverse transcriptase, and 40 U of RNase inhibitor for 45 min at 42℃. PCR was then carried out in an automatic PCR thermocycler (Bioneer, Daejeon, South Korea) for 25 cycles of 94℃ for 30 s, 54℃ for 60 s, and 72℃ for 30 s followed by a 7-min extension at 72℃. The PCR products were separated in 2% agarose gels and visualized by EtBr staining. β-actin was used for normalization.
Data are presented as the mean ± standard deviation (SD). Differences between the mean values for individual groups were assessed with a one-way ANOVA and Duncan's multiple range tests. P-values < 0.05 were considered statistically significant. The SAS v9.1 statistical software package (SAS Institute Inc., Cary, NC, USA) was used to conduct the analysis.
Fig. 1A shows the DPPH radical scavenging activities of FSeS, FSS, and AHSS. FSeS effectively scavenged DPPH radicals in a concentration-dependent manner. DPPH scavenging activity levels of 20.7%, 48.1%, and 76.4% was observed for FSeS concentrations of 10, 50 and 100 µg/mL, respectively. The same concentrations of FSS resulted in DPPH radical scavenging activity levels of 17.1%, 34.1%, and 63.8%, respectively. In contrast, the DPPH scavenging activities of AHSS at concentrations ranging from 10 to 100 µg/mL were relatively weak (9.8-17.2%).
As shown in Fig. 1B, the •OH radical scavenging activity of FSeS was stronger than that of FSS. At concentrations of 50 and 100 µg/mL, the •OH scavenging levels of FSeS were 31.5% and 54.2%, respectively. At the same concentrations, the •OH scavenging levels of FSS were 23.1% and 48.6%, respectively. In contrast, AHSS showed only weak •OH radical scavenging activity (16.0%) at a concentration of 100 µg/mL.
H2O2 scavenging activities of FSeS, FSS, and AHSS are presented in Fig. 2. FSeS showed stronger H2O2 scavenging activity (43.6%) than FSS (37.8%) or AHSS (13.3%) at a concentration of 100 µg/mL. Similar results were also observed for concentrations of 10 and 50 µg/mL. It was noted that AHSS exhibited a weak H2O2 scavenging activity that did not increase in a concentration-dependent manner.
To determine whether FSeS, FSS, or AHSS are cytotoxic, LLC-PK1 cells were first treated with the sauce extracts (10 and 100 µg/mL) for 24 h, and cell viability was measured with an MTT assay. FSeS, FSS, and AHSS did not induce cytotoxicity and the viability of all cells was more than 90% (data not shown). Based on these results, concentrations of 10 and 100 µg/mL were used for further studies. As shown in Fig. 3, H2O2 (500 µM) induced death of the LLC-PK cells (cell viability was 56.7%). Following treatment of the H2O2-treated LLC-PK1 cells with 100 µg/mL of FSeS or FSS, cell viability was 83.7% and 75.6%, respectively. Similar results were observed for cells treated with 10 µg/mL of FSeS and FSS. However, treatment with 10 and 100 µg/mL of AHSS resulted cell viability rates of 54.1% and 54.6%. These results demonstrated that FSeS exerted more potent cytoprotective effects compared to FSS and AHSS.
H2O2 significantly increased the ROS levels (to 178.6%) compared to the concentrations found in normal (untreated) cells (Fig. 4). In the presence of H2O2, FSeS (10 and 100 µg/mL) significantly reduced ROS generation. Intercellular ROS levels in cells treated with at 10 and 100 µg/mL of FSeS were 138.9% and 115.8%, respectively. In contrast, ROS levels in the cells treated with 10 and 100 µg/mL of FSS were 159.4% and 144.9%, respectively. AHSS (10 or 100 µg/mL) did not suppress H2O2-induced ROS generation in the LLC-PK1 cells. Treatment with the same concentrations of FSeS, FSS, or AHSS alone did not significantly increase the intracellular ROS levels (data not shown). Taken together, these results suggest that FSeS and (to a lesser extent) FSS are effective ROS scavengers.
Free radicals and ROS-induced oxidative damage are closely associated with lipid peroxidation in the cell membranes, thereby increasing the generation of malondialdehyde (MDA), a biomarker of cell membrane lipid peroxidation [13]. In the present study (Fig. 5), MDA generation markedly increased by almost four times in cells treated with 500 µM H2O2 (1.07 nmol/mg protein) compared to the untreated cells (0.29 nmol/mg protein). However, treatment with FSeS and FSS (particularly FSeS) reduced MDA generation in a concentration-dependent manner. Cells treated with 100 µg/mL FSeS exhibited significantly decreased MDA levels (0.46 nmol/mg) while ones exposed to the same concentration of FSS also showed decreases MDA production (0.64 nmol/mg protein). Similar results were observed for cells treated with 10 µg/mL of FSeS or FSS. In contrast, both concentrations (10 and 100 µg/mL) of AHSS only weakly reduced MDA generation (1.03 and 0.94 nmol/mg protein, respectively).
Under normal conditions, endogenous antioxidant enzymes such as CAT, SOD, and GSH-px are able to protect cells against ROS-induced oxidative damage. The effects of FSeS, FSS, and AHSS on antioxidant enzyme activities in H2O2-treated LLC-PK1 cells are shown in Table 1. Treatment with 500 µM H2O2 lowered CAT activity (1.76 U/mg protein) in the LLC-PK1 cells compared to untreated cells (3.00 U/mg protein). At a concentration of 100 µg/mL, FSeS and FSS increased CAT activity (2.50 U/mg protein with FSeS and 2.00 U/mg protein with FSS) in H2O2-treated LLC-PK1 cells compared to AHSS (1.88 U/mg protein). Similar results were observed for cells treated with 10 µg/mL of FSeS, FSS, or AHSS.
SOD activity in the LLC-PK1 cells treated with 500 µM H2O2 was also significantly decreased (1.75 U/mg protein) compared to the untreated cells (4.27 U/mg protein). At a concentration of 100 µg/mL, FSeS helped restore SOD activity (3.08 U/mg protein); FSS had a similar effect (2.45 U/mg protein). However, treatment with the same concentration of AHSS did not improve SOD activity in the H2O2-treated LLC-PK1 cells.
GSH-px activity was significantly reduced by 500 µM H2O2 (1.91 U/mg protein) compared to the untreated LLC-PK1 cells (4.72 U/mg protein). Treatment with 100 µg/mL of FSeS or FSS resulted in elevated GSH-px activity in the H2O2-treated LLC-PK1 cells (3.11 U/mg protein with FSeS and 2.82 U/mg protein with FSS). However, GSH-px activity was relatively low (2.00 U/mg protein) in cells treated with AHSS up to a concentration of 100 µg/mL.
Effects of the FSeS, FSS, and AHSS extracts on CAT, SOD, GSH-px, and GST mRNA expression in H2O2-treated LLC-PK1 cells was evaluated by RT-PCR. As shown in Fig. 6, H2O2 (500 µM) significantly decreased the mRNA expression of CAT (71.4%), SOD (72.1%), GSH-px (83.4%), and GST (82.2%) compared to that found in the untreated cells. Extracts from all the sauces, particularly FSeS, effectively increased the mRNA expression of CAT (1.8-fold), SOD (2.3-fold), GSH (4.9-fold), and GST (4.2-fold) compared to that observed in the H2O2-treated cells. Following treatment with 100 µg/mL of FSeS, the mRNA expression levels of CAT, SOD, GSH-px, and GST were 1.3-, 1.5-, 2.1-, and 2.7-fold higher, respectively, relative to the levels observed in LLC-PK1 cells treated with 100 µg/mL of AHSS.
Fermented soybean products such as soy sauce and soy pastes are known as health foods in Asia [26]. FSeS and FSS are prepared by fermenting sesame seeds or soybeans, respectively, with Aspergillus oryzae and other starters at 30℃ for over 180 d. Several studies have reported that FSS has many beneficial properties [1,2,3,4,5,6,7,8,9]. During soy sauce fermentation, the soy isoflavones daidzin and genistin are transformed into daidzein and genistein, respectively, by Asperillus fungi [28,29]. In addition, sesame seeds used to make sesame sauce contain high levels of sesamin and sesaminol along with their glycosides [30,31]. Sesamin and sesaminol glucosides are transformed by Asperillus fungi into sesaminol monoglucoside, sesaminol triglucosides, sesaminol 6-catechol, and epsisesamin 2, 6-dicatechol during sesame sauce fermentation [32,33]. AHSS, a product prepared from defatted soybeans (or other protein-rich materials) and 18% food-grade hydrochloric acid by heating (107℃) for 15-20 h, only contains glutamic acid, reducing sugars, and other amino acids [34].
In the present study, we found that FSeS has strong in vitro antioxidant activity against DPPH, •OH, and H2O2 because this sauce contains sesaminol triglucoside and sesaminol 6-catechol [33]. Sesaminol triglucosides and sesaminol 6-catechol have been shown to have significant DPPH radical scavenging activities [32]. The in vitro antioxidant activity of FSS was weaker than that of FSeS. In contrast to the fermented sauce products, non-fermented AHSS had low levels of in vitro antioxidant activity or lacked this activity altogether. These results suggested that FSeS is a potent antioxidant and could help protect against oxidative damage caused by free radicals. Our data also indicated that the fermentation process and compounds in the sauces produced by fermentation are important.
Increased ROS levels results in renal cell damage [35,36]. As an important ROS, H2O2 can cross cell membranes and react with Fe2+ to generate highly reactive •OH radicals through Fenton's reaction. These radicals attack several cellular compounds, such DNA, proteins, or membrane lipids, and cause cell damage [37]. In the present investigation, we demonstrated that both FSeS and FSS prevented H2O2-induced oxidative damage in LLC-PK1 cells, resulting in increased cell viability as assessed by an MTT assay. In particular, FSeS offered greater protection against H2O2-induced damage than FSS. Furthermore, AHSS was not able to prevent H2O2-induced damage in the LLC-PK1 cells. The protective effects of FSeS and FSS may be correlated with their strong •OH and H2O2 scavenging activities.
MDA, a major end product of the reaction between free radicals and cellular polyunsaturated fatty acids (PUFAs), is an appropriate factor for evaluating the degree of ROS-induced cell damage. Previous studies have demonstrated that renal cell damage caused by H2O2 is directly associated with increased peroxidation of PUFAs in the cell membrane [15,38,39]. However, treatment with antioxidants such as α-tocopherol, 21-aminosteroid, 2-methyl aminochroman, or hydroxyrosol and its metabolites is able to ameliorate H2O2-induced renal cell damage through the inhibition of MDA generation [38,39,40]. Our results suggested that H2O2 (500 µM) significantly increased the total intercellular ROS levels, resulting in the production of MDA in the LLC-PK1 cells. Treatment with FSeS and FSS (particularly 100 µg/mL of FSeS) significantly reduced ROS and MDA levels in H2O2-treated LLC-PK1 cells. In contrast, AHSS did not affect the H2O2-induced production of ROS and MDA.
We also found that FSeS was rich in biofunctional compounds including sesamin, sesaminol monoglucoside, sesaminol triglucosides, and sesaminol 6-catechol [33]. Neither daidzein nor genistein have been detected in FSS [33]. Treatment with sesamin was found to significantly decrease ROS production in H2O2-treated rat pheochromocytoma PC12 cells [41]. Sesamin and sesaminol are able to inhibit lipid peroxidation in rat liver and kidney [42,43]. These results indicate that fermented sauces, in particularly FSeS, reduced ROS-induced lipid peroxidation in H2O2-treated LLC-PK1 cells.
ROS and free radicals are scavenged by endogenous antioxidant enzymes including CAT, SOD, GSH-px, and GST. SOD catalyzes the conversion of superoxides (O2·) into H2O2; the H2O2 is further reduced to H2O by CAT. Treatment with CAT and SOD helps prevent ROS-induced damage in LLC-PK1 cells [36,44] and reduced the oxidative stress-induced lipid peroxidation in vivo [45]. GSH-px catalyzed the intercellular reduced GSH which as a hydrogen donor to generate oxidized glutahthine(GSSG), and eliminated H2O2 and catalyzed the reduction of peroxides in living organ [46,47]. In addition, GST is a detoxification enzyme in most mammalian cells, catalyzes the conjugation of electrophilic compounds to GSH [48], and confers protection against H2O2-induced cell death [50]. Our results suggested that H2O2 significantly decreased the mRNA expression and activity of CAT, SOD, and GSH-px in LLC-PK1 cells. Treatment with FSeS and FSS significantly increased the activity and mRNA levels of these antioxidative enzymes. On the other hand, AHSS only exerted a weak effect on the activity and mRNA levels of these enzymes. Similar to our findings, Hou et al. [41] indicated that sesamin and sesamolin significantly increase CAT and SOD activity to prevent H2O2-induced damage in PC12 cells.
In conclusion, we demonstrated that fermented soybean sauce rather than chemically prepared AHSS, and FSeS have potent in vitro antioxidative activities and protect against H2O2-induced oxidative damage in LLC-PK1 cells. This is accomplished through increased activity of endogenous antioxidant enzymes (CAT, SOD, and GSH-px) as well as reduced H2O2-induced ROS and MDA generation. Our findings indicated that the fermentation process and raw materials for fermenting sauces are important for increasing the antioxidative effects. The further studies are needed to determine the detailed protective mechanisms and active compounds of the fermented sauces in oxidative stress-induced damage in vitro and in vivo. The fermented sauces, especially the FSeS can be used as a new health food to prevent oxidative stress-associated diseases.
Figures and Tables
Fig. 1
DPPH (A) and •OH radical scavenging (B) activities of the sauce extracts. Data from three independent experiments are presented as the mean ± SD. a-fDifferent letters over the bars indicate that the mean values are significantly different (P < 0.05) according to Duncan's multiple range test. AHSS, acid-hydrolyzed soy sauce extract; FSS, fermented soy sauce extract; FSeS, fermented sesame sauce extract.
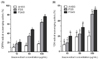
Fig. 2
H2O2 scavenging activities of the sauce extracts. Data from three independent experiments are presented as the mean ± SD. a-dDifferent letters over the bars indicate that the mean values are significantly different (P < 0.05) according to Duncan's multiple range test. AHSS, acid-hydrolyzed soy sauce extract; FSS, fermented soy sauce extract; FSeS, fermented sesame sauce extract.
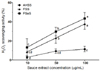
Fig. 3
Protective effect of the sauce extracts against oxidative stress induced by H2O2 in LLC-PK1 cells. Data from three independent experiments are presented as the mean ± SD. a-fDifferent letters over the bars indicate that the mean values are significantly different (P < 0.05) according to Duncan's multiple range test. AHSS, acid-hydrolyzed soy sauce extract; FSS, fermented soy sauce extract; FSeS, fermented sesame sauce extract.
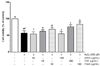
Fig. 4
Effect of sauce extracts on ROS generation in LLC-PK1 cells treated with H2O2. Data from three independent experiments are presented as the mean ± SD. a-eDifferent letters over the bars indicate that the mean values are significantly different (P < 0.05) according to Duncan's multiple range test. AHSS, acid-hydrolyzed soy sauce extract; FSS, fermented soy sauce extract; FSeS, fermented sesame sauce extract.
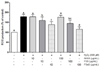
Fig. 5
Effect of sauce extracts on lipid peroxidation levels in LLC-PK1 cells treated with H2O2. Data from three independent experiments are presented as the mean ± SD. a-fDifferent letters over the bars indicate that the mean values are significantly different (P < 0.05) according to Duncan's multiple range test. AHSS, acid-hydrolyzed soy sauce extract; FSS, fermented soy sauce extract; FSeS, fermented sesame sauce extract.
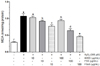
Fig. 6
Effects of the sauce extracts (100 µg/mL) on mRNA expression of CAT, SOD, GSH-px, and GST in LLC-PK1 cells treated with H2O2(500 µM). Band intensity was measured with a densitometer and expressed as fold of the control. Fold ratio = gene expression/β-actin × control numerical value (control fold ratio: 1). a-dDifferent letters over the bars indicate that the mean values are significantly different (P < 0.05) according to Duncan's multiple range test. AHSS, acid-hydrolyzed soy sauce extract; FSS, fermented soy sauce extract; FSeS, fermented sesamin sauce extract.
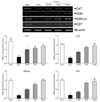
Table 1
Effect of AHSS, FSS, and FSeS on the activity of CAT, SOD, and GSH-px in LLC-PK1 cells exposed to H2O2 (500 µM).

Data from three independent experiments are presented as the mean ± SD. aeMean values with different letters are significantly different (P < 0.05) according to Duncan's multiple range test. AHSS, acid-hydrolyzed soy sauce extract; FSS, fermented soy sauce extract; FSeS, fermented sesame sauce extract; CAT, catalase; SOD, superoxide dismutase; GSHpx, glutathione peroxidase.
References
1. Long LH, Kwee DC, Halliwell B. The antioxidant activities of seasonings used in Asian cooking. Powerful antioxidant activity of dark soy sauce revealed using the ABTS assay. Free Radic Res. 2000; 32:181–186.


2. Wang H, Jenner AM, Lee CY, Shui G, Tang SY, Whiteman M, Wenk MR, Halliwell B. The identification of antioxidants in dark soy sauce. Free Radic Res. 2007; 41:479–488.


3. Yang B, Yang H, Li J, Li Z, Jiang Y. Amino acid composition, molecular weight distribution and antioxidant activity of protein hydrolysates of soy sauce lees. Food Chem. 2011; 124:551–555.


4. Benjamin H, Storkson J, Nagahara A, Pariza MW. Inhibition of benzo(a)pyrene-induced mouse forestomach neoplasia by dietary soy sauce. Cancer Res. 1991; 51:2940–2942.
5. Ito A, Watanabe H, Basaran N. Effects of soy products in reducing risk of spontaneous and neutron-induced liver-tumors in mice. Int J Oncol. 1993; 2:773–776.


6. Nagahara A, Benjamin H, Storkson J, Krewson J, Sheng K, Liu W, Pariza MW. Inhibition of benzo[a]pyrene-induced mouse forestomach neoplasia by a principal flavor component of Japanese-style fermented soy sauce. Cancer Res. 1992; 52:1754–1756.
7. Kataoka S, Liu W, Albright K, Storkson J, Pariza M. Inhibition of benzo[a]pyrene-induced mouse forestomach neoplasia and reduction of H2O2 concentration in human polymorphonuclear leucocytes by flavour components of Japanese-style fermented soy sauce. Food Chem Toxicol. 1997; 35:449–457.


8. Ham SS, Kim SH, Yoo SJ, Oh HT, Choi HJ, Chung MJ. Biological activities of soybean sauce (Kanjang) supplemented with deep sea water and sea tangle. Korean J Food Preserv. 2008; 15:274–279.
9. Yoon KD, Kwon DJ, Hong SS, Kim SI, Chung KS. Inhibitory effect of soybean and fermented soybean products on the chemically induced mutagenesis. Korean J Appl Microbiol Bioeng. 1996; 24:525–528.
10. Namiki M. Nutraceutical functions of sesame: a review. Crit Rev Food Sci Nutr. 2007; 47:651–673.


11. Habermeyer M, Guth S, Eisenbrand G. Identification of gaps in knowledge concerning toxicology of 3-MCPD and glycidol esters. Eur J Lipid Sci Technol. 2011; 113:314–318.


12. Lynch BS, Bryant DW, Hook GJ, Nestmann ER, Munro IC. Carcinogenicity of monochloro-1,2-propanediol (α-chlorohydrin, 3-MCPD). Int J Toxicol. 1998; 17:47–76.


13. Salahudeen AK, Clark EC, Nath KA. Hydrogen peroxide-induced renal injury. A protective role for pyruvate in vitro and in vivo. J Clin Invest. 1991; 88:1886–1893.


14. Small DM, Coombes JS, Bennett N, Johnson DW, Gobe GC. Oxidative stress, anti-oxidant therapies and chronic kidney disease. Nephrology (Carlton). 2012; 17:311–321.


15. Salahudeen AK. Role of lipid peroxidation in H2O2-induced renal epithelial (LLC-PK1) cell injury. Am J Physiol. 1995; 268:F30–F38.


16. Nath KA, Salahudeen AK. Autoxidation of cysteine generates hydrogen peroxide: cytotoxicity and attenuation by pyruvate. Am J Physiol. 1993; 264:F306–F314.


17. Perantoni A, Berman JJ. Properties of Wilms' tumor line (TuWi) and pig kidney line (LLC-PK1) typical of normal kidney tubular epithelium. In Vitro. 1979; 15:446–454.


18. Lobo V, Patil A, Phatak A, Chandra N. Free radicals, antioxidants and functional foods: Impact on human health. Pharmacogn Rev. 2010; 4:118–126.


19. Singh D, Kaur R, Chander V, Chopra K. Antioxidants in the prevention of renal disease. J Med Food. 2006; 9:443–450.


20. Hatano T, Kagawa H, Yasuhara T, Okuda T. Two new flavonoids and other constituents in licorice root: their relative astringency and radical scavenging effects. Chem Pharm Bull (Tokyo). 1988; 36:2090–2097.


21. Halliwell B, Gutteridge JM, Aruoma OI. The deoxyribose method: a simple "test-tube" assay for determination of rate constants for reactions of hydroxyl radicals. Anal Biochem. 1987; 165:215–219.


22. Rosen GM, Rauckman EJ. Spin trapping of superoxide and hydroxyl radicals. Methods Enzymol. 1984; 105:198–209.
23. Fraga CG, Leibovitz BE, Tappel AL. Lipid peroxidation measured as thiobarbituric acid-reactive substances in tissue slices: characterization and comparison with homogenates and microsomes. Free Radic Biol Med. 1988; 4:155–161.


24. Nelson DP, Kiesow LA. Enthalpy of decomposition of hydrogen peroxide by catalase at 25 degrees C (with molar extinction coefficients of H2O2 solutions in the UV). Anal Biochem. 1972; 49:474–478.


25. Marklund S, Marklund G. Involvement of the superoxide anion radical in the autoxidation of pyrogallol and a convenient assay for superoxide dismutase. Eur J Biochem. 1974; 47:469–474.


26. Hafeman DG, Sunde RA, Hoekstra WG. Effect of dietary selenium on erythrocyte and liver glutathione peroxidase in the rat. J Nutr. 1974; 104:580–587.


27. Esaki H, Onozaki H, Osawa T. Antioxidative activity of fermented soybean products. In : Huang MT, Osawa T, Ho CT, Rosen RT, editors. Food Phytochemicals for Cancer Prevention I. ACS Symposium Series. Vol. 546. Washington, D.C.: American Chemical Society;1994. p. 353–360.
28. Chang TS, Ding HY, Tai SS, Wu CY. Metabolism of the soy isoflavones daidzein and genistein by fungi used in the preparation of various fermented soybean foods. Biosci Biotechnol Biochem. 2007; 71:1330–1333.


29. Lee IH, Chou CC. Distribution profiles of isoflavone isomers in black bean kojis prepared with various filamentous fungi. J Agric Food Chem. 2006; 54:1309–1314.


30. Katsuzaki H, Kawakishi S, Osawa T. Sesaminol glucosides in sesame seeds. Phytochemistry. 1994; 35:773–776.


31. Moazzami AA, Andersson RE, Kamal-Eldin A. HPLC analysis of sesaminol glucosides in sesame seeds. J Agric Food Chem. 2006; 54:633–638.


32. Miyake Y, Fukumoto S, Okada M, Sakaida K, Nakamura Y, Osawa T. Antioxidative catechol lignans converted from sesamin and sesaminol triglucoside by culturing with Aspergillus. J Agric Food Chem. 2005; 53:22–27.


33. Song JL. Anticancer effects of fermented sesame sauce [doctor's thesis]. Busan: Pusan National University;2012.
34. Liu K. Soybeans as Functional Foods and Ingredients. Champaign (IL): AOCS Press;2004.
35. Dong J, Ramachandiran S, Tikoo K, Jia Z, Lau SS, Monks TJ. EGFR-independent activation of p38 MAPK and EGFR-dependent activation of ERK1/2 are required for ROS-induced renal cell death. Am J Physiol Renal Physiol. 2004; 287:F1049–F1058.


36. Khan SR. Hyperoxaluria-induced oxidative stress and antioxidants for renal protection. Urol Res. 2005; 33:349–357.


38. Braughler JM, Pregenzer JF. The 21-aminosteroid inhibitors of lipid peroxidation: reactions with lipid peroxyl and phenoxy radicals. Free Radic Biol Med. 1989; 7:125–130.


39. Sheridan AM, Fitzpatrick S, Wang C, Wheeler DC, Lieberthal W. Lipid peroxidation contributes to hydrogen peroxide induced cytotoxicity in renal epithelial cells. Kidney Int. 1996; 49:88–93.


40. Incani A, Deiana M, Corona G, Vafeiadou K, Vauzour D, Dessì MA, Spencer JP. Involvement of ERK, Akt and JNK signalling in H2O2-induced cell injury and protection by hydroxytyrosol and its metabolite homovanillic alcohol. Mol Nutr Food Res. 2010; 54:788–796.


41. Hou RC, Huang HM, Tzen JT, Jeng KC. Protective effects of sesamin and sesamolin on hypoxic neuronal and PC12 cells. J Neurosci Res. 2003; 74:123–133.


42. Kang MH, Naito M, Tsujihara N, Osawa T. Sesamolin inhibits lipid peroxidation in rat liver and kidney. J Nutr. 1998; 128:1018–1022.


43. Sirato-Yasumoto S, Katsuta M, Okuyama Y, Takahashi Y, Ide T. Effect of sesame seeds rich in sesamin and sesamolin on fatty acid oxidation in rat liver. J Agric Food Chem. 2001; 49:2647–2651.


44. Thamilselvan S, Byer KJ, Hackett RL, Khan SR. Free radical scavengers, catalase and superoxide dismutase provide protection from oxalate-associated injury to LLC-PK1 and MDCK cells. J Urol. 2000; 164:224–229.


45. Abdollahi M, Ranjbar A, Shadnia S, Nikfar S, Rezaie A. Pesticides and oxidative stress: a review. Med Sci Monit. 2004; 10:RA141–RA147.
46. Comporti M. Glutathione depleting agents and lipid peroxidation. Chem Phys Lipids. 1987; 45:143–169.


47. Nabavi SM, Nabavi SF, Eslami S, Moghaddam AH. In vivo protective effects of quercetin against sodium fluoride-induced oxidative stress in the hepatic tissue. Food Chem. 2012; 132:931–935.

