Abstract
The purpose of this study was to determine the antioxidant effect of fucoxanthin. After rats were fed a normal fat diet (NF), high fat diet (HF), and high fat with 0.2% fucoxanthin diet (HF + Fxn) for 4 weeks, the markers of oxidative stress and antioxidant capacity like lipid peroxidation, plasma total antioxidant capacity (TAC), and activities of antioxidant enzymes (catalase, superoxide dismutase (SOD), and gluthathione peroxidase (GSH-Px)) were determined. mRNA expression of transcription factor, nuclear erythroid factor like 2 (Nrf2), and its target genes such as NAD(P)H quinone oxidoreductase1 (NQO1) and heme oxygenase-1 (HO-1) were also determined. Mean weight gain in the HF + Fxn group was lower, without statistical significance, and the total food intake in the HF + Fxn group was lower than that in the HF group (P < 0.05). The activity of GSH-Px (P < 0.05) in plasma was significantly higher in the HF + Fxn group than those in the HF group (P < 0.05). In the liver, the activities of catalase (P < 0.05) and GSH-Px (P < 0.05) in the HF + Fxn group were significantly higher than those in the HF group. Plasma TAC level was significantly higher in the HF + Fxn group than that in the HF group (P < 0.05). Lipid peroxidation in plasma tended to be lower without statistical significance. Fucoxanthin supplements were shown to have higher mRNA expression of Nrf2 and NQO1 than those in the high fat diet only group (P < 0.05). In conclusion, supplementation of fucoxanthin improved the antioxidant capacity, depleted by high fat diet, by activating the Nrf2 pathway and its downstream target gene NQO1. Therefore, supplementation of fucoxanthin, especially for those who consume high fat in their diet, may benefit from reduced risk of oxidative stress.
A high fat diet has been associated with obesity, and obesity is believed to cause over production of reactive oxygen species (ROS) [1]. These ROS are known to cause cellular damage, which is implicated in the pathogenesis of various diseases such as diabetes, heart diseases, cancer, and infectious illnesses [1]. In animal study, increased lipid peroxidation in rats fed high fat diet was reported by Milagro et al. [2], while a human study also showed that oxidative stress was correlated with BMI in obese subjects [3].
Nuclear erythroid factor like 2 (Nrf2) is a master regulator of antioxidative defense responses and controls the expression of important antioxidant and detoxification genes (Phase II genes) [4]. Phase II genes, including heme oxygenase-1 (HO-1), glutathione S-transferases (GSTs), and NAD(P)H quinone oxidoreductase (NQO1), scavenge reactive oxygen/nitrogen species (ROS/RNS), detoxify electrophiles and xenobiotics, and maintain intracellular reducing potential [5].
Natural plants that are effective for preventing obesity and obesity related chronic diseases without producing side effects have gained much attention [6]. In addition, efforts to utilize these natural ingredients into commercially available products have been accelerated.
Fucoxanthin is a kind of xanthophylls that has a unique structure which include an allenic bond, epoxide group, and hydroxyl group which differs from that of β-carotene [7]. Due to its unique chemical structure, fucoxanthin is considered a potential antioxidant [8]. In fact, numerous studies have investigated the correlation between anti-inflammatory and anti-cancer effects with the antioxidant function of fucoxanthin [9-13]. The latest in vitro study reported that fucoxanthin markedly decreased the levels of thiobarbituric acid-reactive substances (TBARS) and protein carbonyl contents while significantly increasing the glutathione levels in a concentration-dependent manner [14]. Fucoxanthin also enhanced the expression of transcription factor like Nrf2, thus increasing mRNA and protein expression of HO-1 and NQO1 after incubation with fucoxanthin [15]. However, few in vivo studies regarding the molecular mechanisms of fucoxanthin's antioxidant function has been conducted. Therefore, this study investigated the effect of adding fucoxanthin into a high fat diet on oxidative stress, antioxidant capacity, and mRNA expression of proteins related with antioxidant action.
Four-week-old male Sprague-Dawley (Central Lab. Animal Inc., Korea) rats were fed either a normal fat diet (NF) or a high fat diet (HF) for 1 week. Afterwards, high fat diet groups (HF) were divided into two groups: HF diet only and HF with fucoxanthin diet (HF + Fxn). Rats were fed the experimental diet for 4 more weeks. Both control and experimental group were fed the same AIN-93G diet (ICN, Irvine, CA, USA), except for the fat and carbohydrate contents. The NF group was fed with standard AIN-93G diet including 7% fat (soybean oil), 20% from protein (casein), and 73% from carbohydrate. The vitamin and mineral contents of the diets were identical containing 0.035% mineral mixture and 0.01% vitamin mixture. The HF group had the same diet except that it consisted of 20% fat. HF + Fxn diet included 20% fat, 20% protein, 72.8% carbohydrates, and 0.2% fucoxanthin (40 g/kg diet). Fucoxanthin, a powder form mainly consisting of glycolipids containing 5% fucoxanthin , extracted from seaweed, was purchased from Amicogen, Inc (Jinju, Korea). Rats were maintained individually in stainless steel cages in conditioned rooms (24 ± 1℃, 12 h light/dark cycle). The amount of food eaten and weight were measured twice weekly. The amount of food eaten was measured on a consistent time frame to calculate the food efficiency ratio. All animal studies were treated in accordance with the Dankook University ethics committee's guidelines for the care and use of laboratory animals (approval code: 12-004).
After 4 weeks of diet treatments, rats were fasted for 12 h and then sacrificed. Blood samples were collected directly from the heart by cardiac puncture. Plasma from blood was collected by centrifugation at 4,000 × g for 30 min and then stored at -70℃ for further analysis. The liver, epididymal fat pad, intestinal fat pad, kidney, thymus, and spleen were removed and rinsed with 0.9% NaCl solution. The organs were then dried with filter paper, and their weights were measured. Liver homogenates were prepared on ice in a ratio of 1 g wet tissue for 9 ml phosphate buffer (50 mM, pH 7). Homogenates were then centrifuged at 1000 g for 10 min at 4℃, and supernatant was collected and stored at -70℃ until the analysis of enzyme activities and protein content [16].
Lipid peroxide level in plasma was determined using thiobarbituric acid (TBA) assay as described previously [17]. Briefly, 100 µL of 8.1% (w/v) sodium dodecylsulphate, 750 µL of 20% (v/v) acetic acid (pH 3.5), and 750 µL of 0.8% TBA were added in 100 µL of plasma to a total volume of 2 mL using water. The mixture was boiled for 30 min in water bath and then cooled at room temperature. Five hundred µL of cold water and 2.5 mL of butanol and pyridine were added into the mixture in order at 15:1 (v/v), and then centrifuged. After centrifugation, the supernatants were measured for absorbance at 532 nm using a spectrophotometer. Standard curve using various concentrations of 1,1,3,3-tetra ethoxy propane (TEP) were used for calculation and the results was expressed as TBA-reactive substances (TBARS) concentration (nmol/mL).
The total antioxidant capacity (TAC) was measured using an antioxidant assay kit (Sigma, St Louise, Mo, USA). TAC was measured using the ferrylmyoglobin/ABTS spectrophotometric assay by generating the chromogenic ABTS + radical cation from the interaction between ABTS, metmyoglobin, and hydrogen peroxide as previously described [18,19]. Trolox was used as an antioxidant standard to calculate Trolox equivalent antioxidant capacity; absorbance readings were taken at 520 nm.
Activity of catalase in plasma and liver homogenates was determined by measuring the amount of decrease in absorption at 240 nm in a reaction mixture containing phosphate buffer (0.1 mmol/L, pH 7.0) and H2O2 (8.8 mmol/L) according to Aebi [20]. One catalase unit was defined as the amount of enzyme required to decompose 1 mmol/L of H2O2/min. SOD was measured with a SOD determination kit (Sigma, MO, USA) using Dojindo's highly water-soluble tetrazolium salt, WST-1 (2-(4-Iodophenyl)-3-(4-nitrophenyl)-5-(2,4-disuLfophenyl)-2H-tetrazolium, monosodium salt) [21]. SOD activity was quantified by measuring the decrease in color development at 440 nm. GSH-Px activity with H2O2 as a substrate was measured according to Paglia and Valentine [22]. The enzyme reaction in a tube containing NADPH, reduced glutathione (GSH), sodium azide, and glutathione reductase was initiated by the addition of H2O2, and the change in absorbance was monitored at 340 nm. Antioxidant activity was expressed in units per milliliter in plasma and in units per milligram tissue protein in liver. Liver protein level was measured by the method of Lowry et al. [23].
The total RNA was isolated from liver samples using TRI-reagent (Sigma, MO, USA) according to the manufacturer's protocol [24]. Briefly, after liver samples (0.2 g) were homogenized in TRI Reagent and mixed with 200 µL chloroform (Sigma, MO, USA), the mixture was centrifuged and then added isopropanol into the supernatant. After removing the supernatant, the RNA pellet was washed with 75% ethanol, dried and then dissolved with RNA in 50 µL RNase-free dH2O/0.1 mmol/L EDTA. The purity of each RNA preparation was estimated from the optical density at 260 and 280 nm, respectively.
Synthesis of cDNA and determination of mRNA expression of transcription factor and enzymes such as Nrf2, HO-1, NQO-1, and β-actin were performed by real-time detection PCR as recently described in detail [25,26]. cDNA was synthesized using SuperScript II reverse transcriptase (Invitrogen, CA, USA). Forward and reverse primers were 5'-gaagaagattgcgcagaagg-3' and 5'-gaaggcggtcttagcctctt-3' for HO-1, 5'-cgcagagaggacatcattca-3 and 5'-cgccagagatgactcaacag-3' for NQO-1, 5'-gagacggccatgactgattt-3' and 5'-cagtgaggggatcgatgagt-3' for Nfe-2, and 5'-aggtcatcactactatcggcaat-3' and 5'-actcatcgtactcctgcttg-3 for β-actin. Ct-values of target genes and the reference gene were obtained using an Applied Biosystems StepOne Plus RT-PCR system (Applied biosystems, CA, USA). Relative mRNA abundance of the investigated genes is expressed as fold change in the target genes compared to the control (β-actin).
Statistical analysis was performed using the Statistical Analysis System (SAS Institute, Cary, NC, USA). Data are expressed as mean with standard error, and statistically significant differences among groups were evaluated using one way ANOVA (analysis of variance). Statistically significant differences among the means of groups were tested at α = 0.05 using Duncan's multiple range tests.
Although the final body weight of the HF + Fxn group (325.9 ± 6.9 g) was lower than that of the HF group (332.9 ± 8.6 g), no significant difference in final body weight was observed between the two group (Table 1). The total food intake of the HF + Fxn group was significantly lower than that of the HF group. The liver weight of the HF (15.1 ± 0.7 g) or HF + Fxn group (15.7 ± 0.3 g) was significantly heavier than that of the NF group (10.4 ± 0.2 g, P < 0.05, Table 2). No significant differences in the weights of the kidney, thymus, spleen, and total adipose tissue among the groups were observed.
The plasma GSH-Px concentration in the HF + Fxn group (2.48 ± 0.07 U/mL) was significantly higher than that in the HF group (1.80 ± 0.07 U/mL) (Table 3). In liver antioxidant enzymes, the activities of catalase and GSH-Px were significantly increased when fucoxanthin was added to the high fat diet, compared with the high fat only diet (P < 0.05). No significant difference in SOD activity in the liver was observed among groups. Plasma TAC concentration was significantly higher in the HF + Fxn group (0.87 ± 0.01 mmol/L) than that in the HF group (0.80 ± 0.03 mmol/L) (P < 0.05) (Fig. 1). Although plasma TBARS concentration in the HF + Fxn group was decreased, compared with the HF group, no statistical significance was shown (Fig. 2).
When rats were fed a high fat diet containing fucoxanthin (HF + Fxn), the mRNA expression of hepatic Nrf2 and NQO1 were significantly increased compared to that in the HF group (P < 0.05) (Fig. 3). The mRNA expression of HO-1 in that HF + Fxn group was higher than that in the HF group, but statistical significance was not shown.
This study showed that 0.2% of fucoxanthin rich power supplementation with high fat diet in rats was not very effective in reducing weight gain (Table 1) or weight of adipose tissue (Table 2). Although the final body weight of the HF + Fxn group (325.9 ± 6.9 g) was lower than that of the HF group (332.9 ± 8.6 g), no significant difference was observed (Table 1). In contrast with our study, significant weight loss and anti-obesity effects were observed in Park's et al.'s study [27] and the reduced weight size of adipose tissue was found in Maeda et al.'s study [28]. Explanations for this discreptancy maybe due to the differences in the fucoxanthin amount supplemented in the diet or the duration of feeding period. In fact, 2% of fucoxanthin was added to the diet in Maeda et al.'s study [28] whereas only 0.2% of fucoxanthin was added in this study.
In this study, high fat diet in rats was associated with decreased overall antioxidant systems, but fucoxanthin supplementation in high fat diet significantly improved antioxidant capacities in plasma and in liver. The plasma and liver GSH-Px activities as well as liver catalase activity in the HF + Fxn group were significantly increased, compared with the high fat only diet (Table 3, P < 0.05). Plasma TAC concentration was also significantly improved in the HF + Fxn group than that in the HF group (P < 0.05). Depleted antioxidant system in the body is related to oxidative stress induced by high fat diet [29-31]. When rats were fed a high fat diet, a significant decrease in antioxidants, along with a significant increase in lipid peroxidation, was shown [29,30].
There has been several in vivo and in vitro studies of antioxidant functions of fucoxanthin. Fucoxantin has potent antioxidant ability of quenching various oxygen radicals such as hydroxyl radicals, superoxide radicals, and singlet oxygen as well as inhibiting oxidative damage induced by H2O2 and ultraviolet B [10,32]. Fucoxanthin supplement was also shown to enhance the activities of antioxidant enzymes such as catalase and glutathione transferase and it provides more effective antioxidant ability than β-carotene [8,33].
This study also showed that protein expression of transcription factor, Nrf2, and NQO1, its target gene, were depleted in rats fed high fat diet, and the supplementation of fucoxanthin significantly increased those antioxidant related gene expressions. In the present study, the increase in Nrf2 expression was consistent with a higher activity of the antioxidant enzymes such as GSH-px and calalase observed in the liver and the plasma after fucoxanthin supplementation. Thus it can be suggested that fucoxanthin supplementation improves antioxidant system of rats fed a high fat diet, which is resulted from activation of transcription factor, Nrf2 and its target gene, Nqo2. Itoh et al. [34] reported that the gene expression of antioxidant proteins is regulated through activation of the transcription factor Nrf2, and Nrf2 further up-regulates its target gene, NQO1 and finally initiates to activate various antioxidant enzymes [35]. Some natural compounds such as quercetin, curcumin, or blueberry were shown to increase the mRNA expression of antioxidant enzymes such as HO-1, NQO1, and GSTs through activation of Nrf2 [6,25,36]. It has been shown that fucoxnathin treatment (0.5-5 µmol/L) for 12 h, in vitro study, activates the transcription factor Nrf2 as well as the mRNA expressions of HO-1 and NQO1 in a dose-dependent manner [15]. A study showed that high fat diet decreases expression of Nrf2 and its target gene and reduced expression is related to increased oxidative stress in liver of rats [37].
In conclusion, this study suggests that fucoxanthin exerts its antioxidant effect via enhancement of antioxidant enzyme activities and plasma total antioxidant capacity and fucoxanthin increase the protein expression of Nrf2 leading to enhanced expression of antioxidant protein NQO1. Therefore, addition of fucoxanthin to a high fat diet is considered to be beneficial in improving antioxidant systems in the body by antioxidant activities of fucoxanthin through Nrf2 pathways.
Figures and Tables
![]() | Fig. 1
Effect of fucoxanthin rich powder on plasma total antioxidant capacity (TAC) in rats fed high fat diet. Rats were divided into three groups; normal fat diet group (NF group, 7% fat), high fat diet group (HF group, 20% fat), and high fat diet with 0.2% fucoxanthin (HF+Fxn group). Each bar represents the mean ± S.D. (n = 8). Different letters above each bar indicate significant differences among the groups at α = 0.05 as determined by Duncan's multiple range tests. |
![]() | Fig. 2
Effect of fucoxanthin rich powder on plasma lipid peroxidation in rats fed with high fat diet. Rats were divided into three groups; normal fat diet group (NF group, 7% fat), high fat diet group (HF group, 20% fat), and high fat diet with 0.2% fucoxanthin (HF+Fxn group). Each bar represents the mean ± S.D. (n = 8). NS stands for not statistically significant among the groups as determined by Duncan's multiple range tests. |
![]() | Fig. 3
Effect of fucoxanthin rich powder on mRNA expression of transcription factor and enzymes related to antioxidant system in the liver of rats. Total RNA was isolated using TRI-reagenet, and cDNA was synthesized using 3 ug of total RNA with SuperScript II reverse transcriptase. Realtime PCR was performed using SYBR green and standard procedures to assess the mRNA expression of the primer in liver samples obtained from each group. An Applied Biosystem StepOne softwere v2.1 was used. Each bar represents the mean ± S.E of three independent experiments. Different letters above each bar indicate significant differences among the groups at α = 0.05 as determined by Duncan's multiple range tests. HO-1: heme oxygenase (decycling) 1, Nrf2: nuclear factor, erythroid derived 2, like 2, NQO-1: NAD(P)H quinone oxidoreductase 1. |
Table 1
Body weight and food intakes of experimental groups
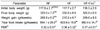
1)NF: normal fat (n = 8), HF: high fat (n = 8), HF+Fxn: high fat with 0.2% fucoxanthin (n = 8)
2)Mean ± SE
3)NS: not significant
4)The significant was determined by Duncan's multiple range test at α = 0.05
5)FER (food efficiency ratio) = Body weight gain for experimental period / Food intake for experimental period
References
1. Dandona P, Aljada A, Chaudhuri A, Mohanty P, Garg R. Metabolic syndrome: a comprehensive perspective based on interactions between obesity, diabetes, and inflammation. Circulation. 2005; 111:1448–1454.
2. Milagro FI, Campión J, Martínez JA. Weight gain induced by high-fat feeding involves increased liver oxidative stress. Obesity (Silver Spring). 2006; 14:1118–1123.


3. Uzun H, Zengin K, Taskin M, Aydin S, Simsek G, Dariyerli N. Changes in leptin, plasminogen activator factor and oxidative stress in morbidly obese patients following open and laparoscopic Swedish adjustable gastric banding. Obes Surg. 2004; 14:659–665.


4. Kwak MK, Itoh K, Yamamoto M, Sutter TR, Kensler TW. Role of transcription factor Nrf2 in the induction of hepatic phase 2 and antioxidative enzymes in vivo by the cancer chemoprotective agent, 3H-1, 2-dimethiole-3-thione. Mol Med. 2001; 7:135–145.


5. Cho HY, Jedlicka AE, Reddy SP, Kensler TW, Yamamoto M, Zhang LY, Kleeberger SR. Role of NRF2 in protection against hyperoxic lung injury in mice. Am J Respir Cell Mol Biol. 2002; 26:175–182.


6. Hsu CL, Yen GC. Phenolic compounds: evidence for inhibitory effects against obesity and their underlying molecular signaling mechanisms. Mol Nutr Food Res. 2008; 52:53–61.


7. Maeda H, Tsukui T, Sashima T, Hosokawa M, Miyashita K. Seaweed carotenoid, fucoxanthin, as a multi-functional nutrient. Asia Pac J Clin Nutr. 2008; 17:Suppl 1. 196–199.
8. Sangeetha RK, Bhaskar N, Baskaran V. Comparative effects of beta-carotene and fucoxanthin on retinol deficiency induced oxidative stress in rats. Mol Cell Biochem. 2009; 331:59–67.


9. Airanthi MK, Hosokawa M, Miyashita K. Comparative antioxidant activity of edible Japanese brown seaweeds. J Food Sci. 2011; 76:C104–C111.


10. Sachindra NM, Sato E, Maeda H, Hosokawa M, Niwano Y, Kohno M, Miyashita K. Radical scavenging and singlet oxygen quenching activity of marine carotenoid fucoxanthin and its metabolites. J Agric Food Chem. 2007; 55:8516–8522.


11. Kumar CS, Ganesan P, Suresh PV, Bhaskar N. Seaweeds as a source of nutritionally beneficial compounds-a review. J Food Sci Technol. 2008; 45:1–13.
12. Khan MN, Choi JS, Lee MC, Kim E, Nam TJ, Fujii H, Hong YK. Anti-inflammatory activities of methanol extracts from various seaweed species. J Environ Biol. 2008; 29:465–469.
13. Kotake-Nara E, Kushiro M, Zhang H, Sugawara T, Miyashita K, Nagao A. Carotenoids affect proliferation of human prostate cancer cells. J Nutr. 2001; 131:3303–3306.


14. Liu CL, Liang AL, Hu ML. Protective effects of fucoxanthin against ferric nitrilotriacetate-induced oxidative stress in murine hepatic BNL CL.2 cells. Toxicol In Vitro. 2011; 25:1314–1319.


15. Liu CL, Chiu YT, Hu ML. Fucoxanthin enhances HO-1 and NQO1 expression in murine hepatic BNL CL.2 cells through activation of the Nrf2/ARE system partially by its pro-oxidant activity. J Agric Food Chem. 2011; 59:11344–11351.


16. Folch J, Lees M, Sloane Stanley GH. A simple method for the isolation and purification of total lipides from animal tissues. J Biol Chem. 1957; 226:497–509.


17. Santos MT, Valles J, Aznar J, Vilches J. Determination of plasma malondialdehyde-like material and its clinical application in stroke patients. J Clin Pathol. 1980; 33:973–976.


18. Miller NJ, Rice-Evans CA. Factors influencing the antioxidant activity determined by the ABTS.+ radical cation assay. Free Radic Res. 1997; 26:195–199.


19. Rice-Evans CA. Measurement of total antioxidant activity as a marker of antioxidant status in vivo: procedures and limitations. Free Radic Res. 2000; 33:Suppl. S59–S66.
20. Aebi H. Catalase in vitro. Methods Enzymol. 1984; 105:121–126.
21. Fridovich I. Superoxide radical: an endogenous toxicant. Annu Rev Pharmacol Toxicol. 1983; 23:239–257.


22. Paglia DE, Valentine WN. Studies on the quantitative and qualitative characterization of erythrocyte glutathione peroxidase. J Lab Clin Med. 1967; 70:158–169.
23. Lowry OH, Rosebrough NJ, Farr AL, Randall RJ. Protein measurement with the Folin phenol reagent. J Biol Chem. 1951; 193:265–275.


24. Livak KJ, Schmittgen TD. Analysis of relative gene expression data using real-time quantitative PCR and the 2(-Delta Delta C(T)) Method. Methods. 2001; 25:402–408.


25. Wang YP, Cheng ML, Zhang BF, Mu M, Wu J. Effects of blueberry on hepatic fibrosis and transcription factor Nrf2 in rats. World J Gastroenterol. 2010; 16:2657–2663.


26. Ueda K, Ueyama T, Yoshida K, Kimura H, Ito T, Shimizu Y, Oka M, Tsuruo Y, Ichinose M. Adaptive HNE-Nrf2-HO-1 pathway against oxidative stress is associated with acute gastric mucosal lesions. Am J Physiol Gastrointest Liver Physiol. 2008; 295:G460–G469.


27. Park HJ, Lee MK, Park YB, Shin YC, Choi MS. Beneficial effects of undaria pinnatifida ethanol extract on diet-induced-insulin resistance in C57BL/6J mice. Food Chem Toxicol. 2011; 49:727–733.


28. Maeda H, Hosokawa M, Sashima T, Miyashita K. Dietary combination of fucoxanthin and fish oil attenuates the weight gain of white adipose tissue and decreases blood glucose in obese/diabetic KK-Ay mice. J Agric Food Chem. 2007; 55:7701–7706.


29. Rizvi SI, Maurya PK. Markers of oxidative stress in erythrocytes during aging in humans. Ann N Y Acad Sci. 2007; 1100:373–382.


30. Muthulakshmi S, Saravanan R. Protective effects of azelaic acid against high-fat diet-induced oxidative stress in liver, kidney and heart of C57BL/6J mice. Mol Cell Biochem. 2013; 377:23–33.


31. Dai FJ, Hsu WH, Huang JJ, Wu SC. Effect of pigeon pea (Cajanus cajan L.) on high-fat diet-induced hypercholesterolemia in hamsters. Food Chem Toxicol. 2013; 53:384–391.


32. Heo SJ, Jeon YJ. Protective effect of fucoxanthin isolated from Sargassum siliquastrum on UV-B induced cell damage. J Photochem Photobiol B. 2009; 95:101–107.


33. Ravi Kumar S, Narayan B, Vallikannan B. Fucoxanthin restrains oxidative stress induced by retinol deficiency through modulation of Na(+)K(+)-ATPase and antioxidant enzyme activities in rats. Eur J Nutr. 2008; 47:432–441.


34. Itoh K, Chiba T, Takahashi S, Ishii T, Igarashi K, Katoh Y, Oyake T, Hayashi N, Satoh K, Hatayama I, Yamamoto M, Nabeshima Y. An Nrf2/small Maf heterodimer mediates the induction of phase II detoxifying enzyme genes through antioxidant response elements. Biochem Biophys Res Commun. 1997; 236:313–322.


35. Valerio LG Jr, Kepa JK, Pickwell GV, Quattrochi LC. Induction of human NAD(P)H:quinone oxidoreductase (NQO1) gene expression by the flavonol quercetin. Toxicol Lett. 2001; 119:49–57.

