Abstract
Chronic consumption of a high-fat, high-sucrose (HFHS) diet increases insulin resistance and results in type 2 diabetes mellitus in C57BL/6J mice. Hyperglycemia in diabetics increases oxidative stress, which is associated with a high risk of diabetic complications. The purpose of this study was to examine the hypoglycemic and antioxidant effects of chamnamul [Pimpinella brachycarpa (Kom.) Nakai] in an animal model of type 2 diabetes. The α-glucosidase inhibitory activity of a 70% ethanol extract of chamnamul was measured in vitro. Five-week-old male C57BL/6J mice were fed a basal or HFHS diet with or without a 70% ethanol extract of chamnamul at a 0.5% level of the diet for 12 weeks after 1 week of adaptation. After sacrifice, serum glucose, insulin, adiponectin, and lipid profiles, and lipid peroxidation of the liver were determined. Homeostasis model assessment for insulin resistance (HOMA-IR) was determined. Chamnamul extract inhibited α-glucosidase by 26.7%, which was 78.3% the strength of inhibition by acarbose at a concentration of 0.5 mg/mL. Serum glucose, insulin, and cholesterol levels, as well as HOMA-IR values, were significantly lower in the chamnamul group than in the HFHS group. Chamnamul extract significantly decreased the level of thiobarbituric acid reactive substances and increased the activities of superoxide dismutase, catalase, and glutathione peroxidase in the liver compared with the HFHS group. These findings suggest that chamnamul may be useful in prevention of hyperglycemia and reduction of oxidative stress in mice fed a HFHS diet.
The prevalence of diabetes mellitus has been increasing at an epidemic rate [1]. The number of people with diabetes worldwide increased from 153 million in 1980 to 347 million in 2008. Most of this increase has been due to a rise in the prevalence of type 2 diabetes, which is characterized by hyperglycemia, primarily due to increased insulin resistance and impaired insulin secretion [2].
The primary goals of diabetes treatment are to control hyperglycemia and prevent diabetic complications, which threaten the lives of diabetic patients, reduce the quality of life, and account for much of the social and financial burdens imposed by the disease [3,4]. Strict control of blood glucose levels is also the most important factor in reducing the risk of diabetic complications [3]. Among oral hypoglycemic agents commonly used by patients with type 2 diabetes, α-glucosidase inhibitors, such as acarbose, are used to inhibit the function of α-glucosidase in the small intestine, resulting in delayed digestion and absorption of dietary complex carbohydrates [5]. Although acarbose has the potential advantage of posing a low risk of hypoglycemia, it may induce side effects including flatulence, abdominal cramping, vomiting, and diarrhea [6]. Therefore, many studies have been undertaken to identify α-glucosidase inhibiting activity in plants, which have minimal side effects [7-9]. Among the plants in which this activity has been found, guava leaf extract has been accepted as a health/functional food item in Korea [10].
Hyperglycemia in a diabetic state induces the overproduction of reactive oxygen species (ROS) and free radicals [11]. The resulting oxidative stress has been proposed to be the root cause of the progression of diabetes and an important mediator of diabetic complications [12]. Accumulating evidence has suggested that antioxidant nutrients and phytochemicals are beneficial in alleviating diabetic complications [13,14]. Thus, agents with hypoglycemic and antioxidant activities could be very promising for the management of diabetes.
Chamnamul [Pimpinella brachycarpa (Kom.) Nakai] is an edible green, leafy vegetable commonly consumed in northeastern Asian regions [15] that belongs to the Pimpinella L. genus, Umbelliferae family [16]. It is used to prepare namul, a seasoned vegetable dish consumed raw or parboiled in Korea. Chamnamul has been reported to have antioxidant activity in vitro [17,18]. It displays 2,2-diphenyl-1-picrylhydrazyl (DPPH) radical-scavenging activity [17] and has been shown to inhibit the production of intracellular ROS induced by hydrogen peroxide [18]. Thus, chamnamul could be helpful in reducing the oxidative stress associated with diabetes. However, the antioxidant effects of chamnamul have not been investigated in vivo. Thus, the purpose of the present study was to investigate the antioxidant effects of chamnamul in an animal model of type 2 diabetes mellitus. In addition, the hypoglycemic activity of chamnamul in vivo and α-glucosidase inhibitory activity in vitro were measured. We investigated the hypoglycemic and antioxidant effects of chamnamul in C57BL/6J mice fed a high-fat, high-sucrose (HFHS) diet. These mice have been reported to become obese and develop insulin resistance and type 2 diabetes when fed such a diet [19-21]. The results will provide the basis for determining the potential benefits of chamnamul in diabetes mellitus.
Casein, D,L-methionine, a mineral mixture, and a vitamin mixture were purchased from ICN Pharmaceuticals Inc. (Costa Mesa, CA, USA) and tert-butyl hydroquinone was purchased from Fluka Co. (Milwaukee, WI, USA). Cornstarch was obtained from Daesang Co. (Seoul, Korea) and sucrose and soybean oil were purchased from Cheiljedang Co. (Seoul, Korea). Lard was obtained from the Lotte Samgang Co. (Seoul, Korea). Acarbose was purchased from Bayer Korea Ltd. (Seoul, Korea). Assay kits for glucose, triglycerides, and cholesterol were acquired from the Asan Co. (Seoul, Korea) and an insulin assay kit was obtained from the Linco Co. (St. Charles, MO, USA). An enzyme-linked immunosorbent assay (ELISA) kit for adiponectin supplied by BioVendor Research and Diagnostic Products (Modrice, Czech Republic) was used. Yeast α-glucosidase, p-nitropheny-α-D-glucopyranoside, Alphacel, choline bitartrate, and all other reagent-grade chemicals were obtained from the Sigma Chemical Co. (St. Louis, MO, USA).
Chamnamul was obtained from a local market in Busan, Korea, washed several times with water, drained, freeze dried, and powdered. The chamnamul powder was extracted twice with 20 volumes of 70% ethanol for 24 h at room temperature, and the solvent was removed by rotary evaporation under vacuum [17]. The extraction yield was 13.4%.
Yeast α-glucosidase inhibitory activity was measured using a method described by Watanabe et al. [22]. Yeast α-glucosidase (0.7 U) was dissolved in 100 mM phosphate buffer (pH 7.0) containing 2 g/L bovine serum albumin and 0.2 g/L NaN3 to prepare the enzyme solution. p-Nitrophenyl-α-D-glucopyranoside (5 mM) in the same buffer was used as a substrate solution. The inhibitory activities of 70% ethanol extracts of chamnamul and acarbose, a positive control, against α-glucosidase were measured at concentrations of 0.5 mg/mL using a microplate reader (model 550; Bio-Rad, Hercules, CA, USA). All measurements were performed in triplicate.
Five-week-old male C57BL/6J mice (n = 21) obtained from Bio Genomics, Inc. (Seoul, Korea) were housed individually in plastic cages and maintained under standard laboratory conditions at a temperature of 24 ± 5℃ and a relative humidity of 55 ± 5% with a regular light cycle (06:00-18:00 light, 18:00-06:00 dark). The mice were divided randomly into three groups after 1 week of adaptation, during which time they had free access to commercial chow. Control, HFHS, and chamnamul groups were fed a basal diet, HFHS diet, and HFHS diet containing a 70% ethanol extract of chamnamul at 0.5% of the diet, respectively, ad libitum for 12 weeks (Table 1). The compositions of the basal and HFHS diets were determined based on previous studies [19-21], in which C57BL/6J mice developed type 2 diabetes when fed a diet high in fat and simple sugar. The basal diet contained 5% corn oil and 65% cornstarch, and the HFHS diet contained 3% corn oil, 33% lard, 11% cornstarch and 27.1% sucrose. The fat-calorie percentages of the basal and HFHS diets were 11.7% and 58.3%, respectively. Body weight and food intake were measured once and three times a week, respectively. All study procedures were approved by the Animal Resource Center at Inje University (2012-51).
At the end of the feeding trials, the mice were sacrificed by heart puncture following an overnight fast. Blood samples were collected immediately and serum was obtained by centrifugation (1,500 g, 15 min). After collecting the blood, liver samples were taken. Serum and liver samples were stored at -70℃ for further analysis.
Serum glucose [23], triglyceride [24], and cholesterol levels were measured by enzymatic methods [25] using commercial kits. Serum insulin levels were determined by radioimmunoassay using commercial kits. Homeostasis model assessment for insulin resistance (HOMA-IR) values were estimated by dividing the product of fasting insulin (µU/mL) and glucose (mmol/L) levels by 22.5 [26]. Serum adiponectin levels were measured using a commercial ELISA kit.
Hepatic lipid peroxidation was evaluated as a thiobarbituric acid reactive substance (TBARS) using the method of Ohkawa et al. [27]. Liver tissue was homogenized in five volumes of 10 mM sodium phosphate buffer (pH 7.4). The homogenate (0.5 mL) was mixed with a solution comprising 15% trichloroacetic acid, 0.4% TBA, and 2.5% HCl (1 mL) and then incubated at 100℃ for 45 min. After cooling, the reaction mixture was centrifuged at 1,500 × g for 10 min and absorbance was then measured at 534 nm. The protein content was measured using the Bradford method [28] with bovine serum albumin as a standard. The level of lipid peroxides was expressed as nmol malondialdehyde (MDA)/mg protein.
To measure the activities of antioxidant enzymes, liver samples were homogenized in 10 volumes of 50 mM phosphate buffer (pH 7.4). The homogenates were centrifuged at 700 g for 10 min, and the supernatant was further centrifuged at 10,000 g for 10 min to collect mitochondrial pellets for the measurement of catalase (CAT) activity. The remaining supernatant was further centrifuged at 100,000 g for 60 min, and the final supernatant was used to measure superoxide dismutase (SOD) and glutathione peroxidase (GSH-Px) activities. CAT activity was determined according to the Aebi method [29]. One unit of CAT activity was defined as µmoles of hydrogen peroxide reduced per minute. Activities of SOD and GSH-Px were measured by the Marklund and Marklund method [30] and a method developed by Paglia and Valentine [31], respectively. One unit of SOD activity was defined as the amount of enzyme that reduced the rate of pyrogallol autoxidation by 50%. One unit of GSH-Px activity was defined as nmoles of NADPH substrate converted to NADP+ per minute. All assays were carried out in triplicate and the enzyme activity was expressed as specific activity (U/mg protein).
All data are expressed as means ± standard error of the mean (SEM). One-way analysis of variance with a post hoc Tukey's test was used to evaluate significant differences among groups (P < 0.05). Statistical analysis was performed with SAS version 9.2 (SAS Institute Inc., Cary, NC, USA).
The inhibitory activities of the 70% ethanol extract of chamnamul and acarbose against yeast α-glucosidase in vitro are shown in Fig. 1. The chamnamul extract and acarbose inhibited α-glucosidase by 26.7% and 34.1%, respectively, at 0.5 mg/mL.
The body weights, weight gains, food intakes, and feed efficiency ratios (FERs) of the animals are listed in Table 2. The final body weight and weight gain were significantly higher in the HFHS group than in the control group (P < 0.01). The consumption of chamnamul did not significantly influence the final body weight and weight gain in mice fed a HFHS diet. The FER was significantly higher in the HFHS group than in the control group (P < 0.01). The FER tended to be lower in the chamnamul group than in the HFHS group, although this difference was not significant.
Serum glucose levels were significantly elevated in the HFHS group (170.5 ± 8.9 mg/dL) compared with the control group (129.2 ± 6.6 mg/dL; P < 0.01; Table 3). However, the consumption of chamnamul extract significantly lowered serum glucose levels (144.5 ± 7.0 mg/dL; P < 0.05) compared with those in the HFHS group. No significant difference in serum glucose level was observed between the control and chamnamul groups. Feeding with a HFHS diet increased serum insulin and HOMA-IR values compared with the control group (P < 0.01). Serum insulin and HOMA-IR values in the chamnamul group were significantly lower than those in the HFHS group (P < 0.01) and higher than those in the control group (P < 0.01 and P < 0.05, respectively). Serum adiponectin levels did not differ significantly among the control, HFHS, and chamnamul groups.
Serum cholesterol levels were significantly higher in the HFHS group (144.8 ± 9.1 mg/dL) than in the control group (95.3 ± 6.3 mg/dL; P < 0.01). Chamnamul extract significantly reduced serum cholesterol levels (116.7 ± 7.4 mg/dL; P < 0.05) compared with the HFHS group. Serum cholesterol levels did not differ significantly between the chamnamul and control groups. Serum triglyceride levels did not differ significantly among the three groups.
The effects of chamnamul on lipid peroxide levels and the activity of antioxidant enzymes in the liver are shown in Fig. 2. Consumption of a HFHS diet increased hepatic TBARS levels (0.924 ± 0.063 nmol MDA/mg protein) compared with the control group (0.659 ± 0.038 nmol MDA/mg protein; P < 0.01). The consumption of chamnamul extract reduced hepatic TBARS levels in mice fed a HFHS diet (0.644 ± 0.044 nmol MDA/mg protein; P < 0.01) to values comparable with those of the control group.
SOD activity in the liver was significantly lower in the HFHS group than in the control group (P < 0.01). However, the consumption of chamnamul extract elevated SOD activity by 24.3% compared with the HFHS group (P < 0.05), to values that did not differ significantly from those of the control group. The activities of hepatic CAT and GSH-Px were significantly reduced in the HFHS group compared with the control group (P < 0.05). The consumption of chamnamul extract increased the activities of CAT and GSH-Px by 17.5% and 22.8%, respectively, compared with the HFHS group (P < 0.05). The activities of CAT and GSH-Px did not differ significantly between the chamnamul and control groups.
We determined the effect of chamnamul on blood glucose status and oxidative stress in vivo and α-glucosidase inhibitory activity in vitro to evaluate its benefit for diabetes mellitus. Consumption of a HFHS diet for 12 weeks produced obesity, insulin resistance, hyperinsulinemia, and hyperglycemia in C57BL/6J mice. This finding is in line with those of previous studies [19-21,32,33], which have demonstrated the inducement of type 2 diabetes following consumption of a HFHS diet in these animals. Yang et al. [32] reported that a HFHS diet induced insulin resistance by the downregulation of genes involved in insulin signaling, resulting in hyperinsulinemia and hyperglycemia.
Consumption of chamnamul extract at 0.5% of the diet did not significantly influence HFHS diet-induced obesity. However, chamnamul effectively alleviated hyperinsulinemia and hyperglycemia (Table 3). Chamnamul extract also decreased the HOMA-IR index, a surrogate index of insulin resistance [34]. In addition, the α-glucosidase inhibitory activity of the chamnamul extract was 78.3% as strong as that of acarbose in vitro (Fig. 1). An α-glucosidase inhibitor, such as acarbose, inhibits the digestion of carbohydrates, resulting in a flattening of postprandial hypoglycemia. Lebovitz [35] reported that acarbose reduced glucose toxicity through the alleviation of postprandial hyperglycemia, resulting in an improvement of overall glycemic control. Chamnamul extract may be able to improve fasting hyperglycemia by reducing glucose toxicity and increasing insulin sensitivity. Determining the effect of chamnamul on the expression of insulin signaling genes would be useful for the investigation of the underlying mechanism of improved insulin resistance. A study aiming to isolate and identify the active compound(s) with α-glucosidase inhibitory activity from chamnamul is currently in progress.
Mice fed a HFHS diet displayed increased serum cholesterol (Table 3), which is consistent with the results of previous studies [32,33]. Chamnamul extract effectively alleviated HFHS diet-induced hypercholesterolemia. This extract has been reported to reduce blood cholesterol levels in rats fed a high-cholesterol diet, and chamnamul has been suggested to exert a hypocholesterolemic effect, partly by inhibiting the reabsorption of bile salts [36]. Lowering blood cholesterol levels reduces the risk of cardiovascular complications in patients with diabetes [37].
We investigated the antioxidant effects of chamnamul by determining lipid peroxide levels and enzyme activity involved in the antioxidative system in the liver. Mice fed a HFHS diet had elevated levels of hepatic TBARS and reduced SOD, CAT, and GSH-Px activities compared with animals fed a basal diet (Fig. 2). In diabetics, oxidative stress is increased [11], which can contribute to the development of diabetic complications by the elevation of lipoprotein oxidation [38,39], activation of proinflammatory signals, and inactivation of anti-atherogenic enzymes [40].
Levels of hepatic TBARS were significantly lowered by chamnamul extract in mice fed a HFHS diet. Chamnamul has been reported to be an antioxidant that directly scavenges ROS and free radicals in vitro [17,18]. Therefore, chamnamul could have reduced TBARS levels by behaving as an antioxidant in this study. In addition, chamnamul potentiated the activities of SOD, CAT, and GSH-Px in these animals. SOD catalyzes the dismutation of superoxide radicals to H2O2, which can be converted to water by CAT in the peroxisomes or by GSH-Px in the cytosol [41,42]. Thus, chamnamul could improve antioxidant status, thereby effectively alleviating diabetic complications, in this animal model.
In conclusion, chamnamul extract effectively alleviated hyperinsulinemia, hyperglycemia, and hypercholesterolemia in C57BL/6J mice fed a HFHS diet. It also decreased levels of hepatic lipid peroxides and increased the activities of antioxidant enzymes. These findings suggest that chamnamul may be useful in prevention of hyperglycemia and reduction of oxidative stress in mice fed a HFHS diet.
Figures and Tables
Fig. 1
Inhibitory activities of chamnamul against yeast α-glucosidase. The inhibitory activities of the 70% ethanol extracts of chamnamul and acarbose were measured at a concentration of 0.5 mg/mL. Values represent means ± SEM of triplicate measurements.
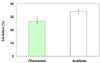
Fig. 2
Lipid peroxide levels and activities of antioixdative enzymes of the liver in the mice fed the experimental diets. (A) TBARS, (B) SOD activity, (C) CAT activity and (D) GSH-Px activity. Groups are the same as in Table 2. Values are presented as mean ± SEM (n = 7). Significantly different at P < 0.05 (*) and P < 0.01 (**).
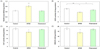
References
1. Danaei G, Finucane MM, Lu Y, Singh GM, Cowan MJ, Paciorek CJ, Lin JK, Farzadfar F, Khang YH, Stevens GA, Rao M, Ali MK, Riley LM, Robinson CA, Ezzati M. Global Burden of Metabolic Risk Factors of Chronic Diseases Collaborating Group (Blood Glucose). National, regional, and global trends in fasting plasma glucose and diabetes prevalence since 1980: systematic analysis of health examination surveys and epidemiological studies with 370 country-years and 2.7 million participants. Lancet. 2011; 378:31–40.


2. King H, Aubert RE, Herman WH. Global burden of diabetes, 1995-2025: prevalence, numerical estimates, and projections. Diabetes Care. 1998; 21:1414–1431.


3. American Diabetes Association (ADA). Summary of revisions for the 2008 clinical practice recommendations. Diabetes Care. 2008; 31:S3–S4.
4. UK Prospective Diabetes Study (UKPDS) Group. Intensive blood-glucose control with sulphonylureas or insulin compared with conventional treatment and risk of complications in patients with type 2 diabetes (UKPDS 33). Lancet. 1998; 352:837–853.
5. Standl E, Baumgartl HJ, Füchtenbusch M, Stemplinger J. Effect of acarbose on additional insulin therapy in type 2 diabetic patients with late failure of sulphonylurea therapy. Diabetes Obes Metab. 1999; 1:215–220.


6. Bressler R, Johnson D. New pharmacological approaches to therapy of NIDDM. Diabetes Care. 1992; 15:792–805.


7. Joo HJ, Kang MJ, Seo TJ, Kim HA, Yoo SJ, Lee SK, Lim HJ, Byun BH, Kim JI. The hypoglycemic effect of Saururus chinensis Baill in animal models of diabetes mellitus. Food Sci Biotechnol. 2006; 15:413–417.
8. Shim YJ, Doo HK, Ahn SY, Kim YS, Seong JK, Park IS, Min BH. Inhibitory effect of aqueous extract from the gall of Rhus Chinensis on alpha-glucosidase activity and postprandial blood glucose. J Ethnopharmacol. 2003; 85:283–287.


9. Wang H, Du YJ, Song HC. α-Glucosidase and α-amylase inhibitory activities of guava leaves. Food Chem. 2010; 123:6–13.


10. Ministry of Food and Drug Safety (KR). Functional Food [Internet]. Cheongwon: Ministry of Food and Drug Safety;2013. cited 2013 Mar 31. Available from: http://www.foodnara.go.kr/hfoodi/.
11. Maritim AC, Sanders RA, Watkins JB 3rd. Diabetes, oxidative stress, and antioxidants: a review. J Biochem Mol Toxicol. 2003; 17:24–38.


12. Kaneto H, Nakatani Y, Kawamori D, Miyatsuka T, Matsuoka TA, Matsuhisa M, Yamasaki Y. Role of oxidative stress, endoplasmic reticulum stress, and c-Jun N-terminal kinase in pancreatic β-cell dysfunction and insulin resistance. Int J Biochem Cell Biol. 2005; 37:1595–1608.


13. Sinclair AJ, Girling AJ, Gray L, Lunec J, Barnett AH. An investigation of the relationship between free radical activity and vitamin C metabolism in elderly diabetic subjects with retinopathy. Gerontology. 1992; 38:268–274.


14. Lean ME, Noroozi M, Kelly I, Burns J, Talwar D, Sattar N, Crozier A. Dietary flavonols protect diabetic human lymphocytes against oxidative damage to DNA. Diabetes. 1999; 48:176–181.


15. Sun GR, Diao SQ, Yao DD. GC-MS analysis of essential oil from Spuriopimpinella brachycarpa. J Northeast For Univ. 2009; 37:102–103.
16. Na H, Kim KW, Kwack Y, Kim SK, Chun C. Comparative anatomy of embryogenic and non-embryogenic calli from Pimpinella brachycarpa. J Plant Biol. 2007; 50:344–350.


17. Kim SJ, Min SC, Shin HJ, Lee YJ, Cho AR, Kim SY, Han J. Evaluation of the antioxidant activities and nutritional properties of ten edible plant extracts and their application to fresh ground beef. Meat Sci. 2013; 93:715–722.


18. Lu J, Qian W, Xu L, Huang G, Cong W, Wang Z, Deng X, Wang D, Guan S. Phytochemical composition and toxicity of an antioxidant extract from Pimpinella brachycarpa (Kom.) Nakai. Environ Toxicol Pharmacol. 2012; 34:409–415.


19. Surwit RS, Kuhn CM, Cochrane C, McCubbin JA, Feinglos MN. Diet-induced type II diabetes in C57BL/6J mice. Diabetes. 1988; 37:1163–1167.


20. Surwit RS, Seldin MF, Kuhn CM, Cochrane C, Feinglos MN. Control of expression of insulin resistance and hyperglycemia by different genetic factors in diabetic C57BL/6J mice. Diabetes. 1991; 40:82–87.


21. Schreyer SA, Vick C, Lystig TC, Mystkowski P, LeBoeuf RC. LDL receptor but not apolipoprotein E deficiency increases diet-induced obesity and diabetes in mice. Am J Physiol Endocrinol Metab. 2002; 282:E207–E214.
22. Watanabe J, Kawabata J, Kurihara H, Niki R. Isolation and identification of α-glucosidase inhibitors from Tochu-cha (Eucommia ulmoides). Biosci Biotechnol Biochem. 1997; 61:177–178.


23. Raabo E, Terkildsen TC. On the enzymatic determination of blood glucose. Scand J Clin Lab Invest. 1960; 12:402–407.


24. Grossman SH, Mollo E, Ertingshausen G. Simplified, totally enzymatic method for determination of serum triglycerides with a centrifugal analyzer. Clin Chem. 1976; 22:1310–1313.


25. Kattermann R, Jaworek D, Möller G, Assmann G, Björkhem I, Svensson L, Borner K, Boerma G, Leijnse B, Desager JP, Harwengt C, Kupke I, Trinder P. Multicentre study of a new enzymatic method of cholesterol determination. J Clin Chem Clin Biochem. 1984; 22:245–251.


26. Haffner SM, Miettinen H, Stern MP. The homeostasis model in the San Antonio Heart Study. Diabetes Care. 1997; 20:1087–1092.


27. Ohkawa H, Ohishi N, Yagi K. Assay for lipid peroxides in animal tissues by thiobarbituric acid reaction. Anal Biochem. 1979; 95:351–358.


28. Bradford MM. A rapid and sensitive method for the quantitation of microgram quantities of protein utilizing the principle of protein-dye binding. Anal Biochem. 1976; 72:248–254.


29. Aebi H. Catalase. In : Bergmeyer HU, Gawehn K, editors. Methods of Enzymatic Analysis. New York (NY): Academic Press;1974. p. 673–684.
30. Marklund S, Marklund G. Involvement of the superoxide anion radical in the autoxidation of pyrogallol and a convenient assay for superoxide dismutase. Eur J Biochem. 1974; 47:469–474.


31. Paglia DE, Valentine WN. Studies on the quantitative and qualitative characterization of erythrocyte glutathione peroxidase. J Lab Clin Med. 1967; 70:158–169.
32. Yang ZH, Miyahara H, Takeo J, Katayama M. Diet high in fat and sucrose induces rapid onset of obesity-related metabolic syndrome partly through rapid response of genes involved in lipogenesis, insulin signalling and inflammation in mice. Diabetol Metab Syndr. 2012; 4:32.


33. Fernandes-Santos C, Carneiro RE, de Souza Mendonca L, Aguila MB, Mandarim-de-Lacerda CA. Pan-PPAR agonist beneficial effects in overweight mice fed a high-fat high-sucrose diet. Nutrition. 2009; 25:818–827.


34. Muniyappa R, Lee S, Chen H, Quon MJ. Current approaches for assessing insulin sensitivity and resistance in vivo: advantages, limitations, and appropriate usage. Am J Physiol Endocrinol Metab. 2008; 294:E15–E26.


35. Lebovitz HE. α-Glucosidase inhibitors as agents in the treatment of diabetes. Diabetes Rev. 1998; 6:132–145.
36. Lee JJ, Choo MH, Lee MY. Effect of Pimpinella brachycarpa extract on lipid metabolism in rats fed high cholesterol diet. J Korean Soc Food Sci Nutr. 2006; 35:1151–1158.


37. Stamler J, Vaccaro O, Neaton JD, Wentworth D. Diabetes, other risk factors, and 12-yr cardiovascular mortality for men screened in the Multiple Risk Factor Intervention Trial. Diabetes Care. 1993; 16:434–444.


38. Rahimi R, Nikfar S, Larijani B, Abdollahi M. A review on the role of antioxidants in the management of diabetes and its complications. Biomed Pharmacother. 2005; 59:365–373.


39. Witztum JL, Steinberg D. The oxidative modification hypothesis of atherosclerosis: does it hold for humans? Trends Cardiovasc Med. 2001; 11:93–102.


40. Du X, Edelstein D, Obici S, Higham N, Zou MH, Brownlee M. Insulin resistance reduces arterial prostacyclin synthase and eNOS activities by increasing endothelial fatty acid oxidation. J Clin Invest. 2006; 116:1071–1080.

