Abstract
We evaluated folate status of child-bearing age women diagnosed with abnormal pap smear in the US post-folic acid (FA) fortification era and assessed the determinants of NTD-protective and supra-physiologic (SP) concentrations of folate. The distribution of 843 women according to NTD-protective concentrations of RBC folate, plasma folate and SP concentrations of plasma folate were tested in relation to demographic and life-style factors. Logistic regression models specified NTD-protective concentrations of RBC and plasma folate or SP concentrations of plasma folate as dependent variables and demographic and life-style factors as independent predictors of interest. More than 82% reached NTD-protective concentrations of RBC and plasma folate and ~30% reached SP concentrations of plasma folate. FA supplement use was associated with having SP concentrations of plasma folate rather than NTD-protective concentrations of folate. African American (AA) women and smokers were significantly less likely to achieve NTD-protective concentrations of RBC and plasma folate. A large majority of women reached NTD-protective concentrations of folate with the current level of FA fortification without using supplementary FA. Therefore, the remaining disparities in AA women and in smokers should be addressed by targeted individual improvements in folate intake.
Observational studies and intervention trials have demonstrated that periconceptional supplementation with folic acid (FA) reduces the occurrence of birth defects, especially neural tube defects (NTDs) [1,2]. However, public health campaigns have failed to improve folate status through recommendations of FA supplements as demonstrated by a low proportion of women of child-bearing age consuming FA supplements periconceptionally and nearly half of this population having unplanned pregnancies [3]. Fortification of foods with FA thus offered a plausible solution to these failed health campaigns. In 1998 fortification of cereal grains with FA was mandated by the US Food and Drug Administration (FDA), with the main purpose of reducing the risk of NTDs by increasing folate intake in women of child-bearing age [4].
Since the initiation of FA fortification, US National Health and Nutrition Examination Surveys (NHANES) have documented a 1.5- to 3-fold increase in the circulating concentrations of folate. This has resulted in an 80-90% decline in the prevalence of low folate, i.e. < 3 ng/mL of plasma folate and < 140 ng/mL for RBC folate, designated as folate deficiency, particularly among women of child-bearing age, from 1988-2006. An evaluation of the relationship between the risk of NTD and maternal concentrations of folate has shown that the lowest category of NTD risk occurred when maternal plasma and RBC folate concentrations were > 7 ng/mL and > 400 ng/mL [5], respectively, suggesting that achieving this level of folate rather than eliminating folate deficiency should be the goal for individual recommendations or population-wide FA fortification programs for the prevention of NTDs. According to NHANES 1999-2000, in women of child-bearing age who do not use FA supplements, only < 10% reached > 400 ng/mL RBC folate concentrations, despite the fact that serum concentrations were exceeding the acceptable level [6]. A smaller study conducted after FA fortification in 1999 in Southern Californian women who had not consumed supplemental FA within the past 12 months, however, demonstrated that 95% of socio-economically advantaged women and 78% of disadvantaged women, respectively, reached RBC folate concentrations > 400 ng/mL [7]. In a group of Australian women, 64% had RBC folate levels of > 400 ng/mL within 12 months of their FA fortification program [8]. Even though folate deficiency was reported to be virtually nonexistent in the general Canadian population after their FA fortification program, 22% of Canadian women of child-bearing age did not achieve RBC folate concentrations > 400 ng/mL [9]. Well-implemented mandatory fortification programs, unlike voluntary fortification programs, are expected to reduce disparities in folate status. However, individuals with low RBC folate status after fortification are still reported to be concentrated in groups with lower incomes and among African Americans (AAs) in the US [10]. A higher intake of FA from supplements in Caucasian Americans (CAs) compared to AAs may explain these racial differences at least partially [11,12]. Also, other studies have indicated that fortification may not reach all women of reproductive age adequately [13].
Despite the above stated issues, however, the US FA fortification program has also been under scrutiny with regard to potential adverse effects of higher concentrations of folate on many disease conditions. Approximately 23% of the US population has been reported to have supra-physiologic (SP) concentrations of plasma folate (> 19.8 ng/mL) within 2 years of exposure to the FA fortification program [14]. Higher circulating concentrations of folate have been hypothesized to mask pernicious anemia due to vitamin B12 deficiency, cause cognitive impairment in elderly subjects, reduce the efficacy of anti-folate drugs, increase the risk of insulin resistance and obesity in children and have the potential to promote cancer [15-18]. However, folate may also play a beneficial role in cancer prevention via its critical role in synthesis, repair and methylation of DNA [19]. There have also been several reports associating excess body weight with folate concentrations [20,21], and a report showing that after mandatory FA fortification, higher maternal weight was associated with increased risk of NTDs [22]. Even though the presence of un-metabolized FA associated with higher folate status has been hypothesized to exert adverse effects on some of these conditions, there have been no definitive studies to conclusively demonstrate this effect.
With this background, the purpose of this study was to evaluate the status of circulating concentrations of folate in women of child-bearing age in the US post-FA fortification era and assess the determinants of NTD-protective concentrations of RBC and plasma folate and SP concentrations of plasma folate.
The present analysis was based on a subset of women screened after the initiation of the FA fortification program in the US (2004-2011) for two studies funded by the NCI (R01 CA105448 and R01 CA102489). All women were diagnosed with abnormal cervical cells and were referred to a colposcopy clinic at the University of Alabama at Birmingham (UAB) for further examination by colposcopically directed biopsies. The current study included 843 non-pregnant women of child-bearing age (15-45 years) who tested positive for high-risk human papillomaviruses (HR-HPVs), but histologically confirmed to be free of cervical pre-cancer. Women had no previous history of cervical or other genital cancers, previous hysterectomy, or known destructive therapy of the cervix. None of them were current users of anti-folate medications.
Age, race, level of education, parity, cigarette smoking, alcohol consumption and use of hormonal/oral contraceptives and supplements containing FA and their duration of use were ascertained from the risk factor questionnaire administered by the study personnel. CDC physical activity questionnaire data were summarized as two categories according to the number of minutes of moderate physical activity min/week (< 150 or ≥ 150). Height, weight and waist circumference (WC) measurements were obtained using standard protocols by the study personnel, reducing the potential for body mass index (BMI) misclassification compared with self-report. BMI was calculated using the height and weight measurements (weight kg/[height m2]). WC data were summarized as two categories, ≤ 88 cm or > 88 cm. Percentage of body fat (% BF) was measured using a TANITA-bioelectrical impedance analysis instrument (Model TBF-300A) and summarized as two categories, ≤ 33% or > 33%. Fasting blood samples were collected from all women. All protocols were approved by the Institutional Review Board at the UAB.
Fasting blood samples collected in EDTA containing blood collection tubes were kept cold and were transported to the laboratory on ice within two hours of collection. All samples were immediately processed and stored appropriately to assess plasma folate and RBC folate by using a RBC hemolysate method. The hematocrit needed for calculating RBC folate concentrations was measured using 25 µL of whole blood. After mixing 25 µL of whole blood with 725 µL of freshly prepared 1% ascorbate (to preserve folate), samples were incubated at 37℃ to convert folate to microbiologically assayable forms. The remainder of the whole blood sample was centrifuged at 3000 rpm for 10 minutes to separate plasma from RBCs. The hemolysate preparations and plasma were immediately stored at -80℃ until used for folate analysis.
The 96-well plate adaptation of the L. casei (ATTC 7469) microbiological assay was used to measure the total folate levels in the appropriately treated samples of RBC and plasma [23-25]. Briefly, 20 µL of sample and folate standard (15 ng/mL) was used in the assay. The volumes of the sample and the standard were adjusted to 300 µL using 0.1 M phosphate buffer (pH 8.6) containing 10 mg/mL of ascorbic acid. Six serial dilutions of sample and standard (folate standards in the range of 0.005 to 0.15 ng/0.3 mL assay well volume after serial dilutions) were made using a 12-channel pipetter. 150 µL of L. casei medium containing L. casei, at a concentration of 5 µL/10 mL of medium, was pipetted into all wells and incubated for 18 hours at 37℃. After incubation, the contents of each well were suspended by repeated aspiration and flushing several times with a 12-channel pipetter. Bacterial growth was measured by reading the optical density at 655 nm. This assay is based on the ability of the folate in the sample to stimulate L. casei growth relative to folatecontaining standards. The coefficient of variation and recovery of this assay were 5-7% and 96-105% respectively. To monitor the reproducibility of these assays, two pooled samples (low and high) prepared from plasma obtained from the American Red Cross were assayed at least 30 times and the means and standard deviations were determined. These served as the basis for the quality control for the assays. The low and high pools were included in every plate. The study sample assays were repeated if the values of the control samples in each run were beyond two standard deviations from the mean. Plasma and RBC hemolysate from the same individuals were assayed for folate under the same experimental conditions (same plate, same day, etc). All assays were performed within 3 months of sample collection by one research associate throughout the study period using samples which were never subjected to freeze-thaw conditions.
The following formula was used to calculate RBC folate concentrations.
This formula makes a correction for plasma folate present in the whole blood hemolysate and for the proportion of blood that consists of RBCs.
Simple descriptive statistics (mean ± SD or percentages) were used to evaluate the distribution of study subjects according to NTD-protective concentrations of RBC folate (≤ 400 ng/mL vs. > 400 ng/mL), NTD-protective concentrations of plasma folate (≤ 7 ng/mL vs. > 7 ng/mL) and SP concentrations of plasma folate (≤ 19.8 ng/mL vs. > 19.8 ng/mL) by demographic and life-style factors (age, race, education, parity, smoking, alcohol consumption, use of hormonal contraceptives or multi-vitamins containing FA, physical activity and indicators of excess body weight, namely, BMI, WC and % BF). A two-sided chi-square test or Fisher's exact test was used to test the differences in the distribution of RBC or plasma folate concentrations according to demographic and life-style factors, and the Student's t-test was used for comparisons of means. Statistical significance was set as α < 0.05. Logistic regression models specified NTD-protective concentrations of RBC and plasma folate or SP concentrations of plasma folate as dependent variables, and age (as a continuous variable), race (AA vs. CA), educational status (high school or greater vs. less than high school), parity (0 vs. > 0), smoking (non-current vs. current), alcohol consumption (non-current vs. current), use of hormonal contraceptives (non-user vs. user) and multi-vitamins containing FA (infrequent or non-user vs. < 1 year or ≥ 1 year user), physical activity and indicators of excess body weight as independent predictors of interest. Separate models were run using the entire population, those who did not use FA containing multi-vitamins and those who used such supplements. Logistic regression models were used to evaluate the effects of these factors simultaneously. We evaluated the strength and precision of each association by estimating the odds ratio (OR), its 95% confidence interval (CI) and its statistical significance using Wald chi-square statistic of the null hypothesis that the OR was equal to 1. All statistical analyses were conducted using SAS 9.1.3 version (SAS Institute, Inc, Cary NC).
As shown in Table 1, the study included 843 women (43% CAs and 57% AAs) of child-bearing age with a mean age of 24.7 years. Approximately 81% of women reported having a high school or greater level of education while ~65% reported giving birth to 1 or more children. Consumption of alcohol was more common (61%) than smoking (38%) in this population. Approximately 80% of women used hormonal contraceptives. Approximately 14% of women reported regular use (≥ 4 times per week) of a multi-vitamin containing FA and these women were defined as supplement users (SUs). The other 86% of women who did not use FA containing supplements or who were infrequent users of such supplements (< 4 times per week) were defined as non-supplement users (NSUs). Among SUs, 60% and 40% reported the duration of supplement use as < 1 year and ≥ 1 year, respectively. The frequency of regular use (10% among AA vs. 20% among CA) as well as the duration of use of multivitamins containing FA (3% of AA and 9% of CA reporting ≥ 1 year duration) were significantly lower among AA women compared to CA women, P = 0.0001 and P < 0.0001, respectively. The population appeared to have largely a sedentary life style; only 30% of women reporting ≥ 150 minutes of moderate physical activity per week. More than 50% of women had excess body weight as indicated by BMI ≥ 25 kg/m2, WC > 88 cm or % BF > 33%.
The percentage of the population that reached NTD-protective concentrations of RBC and plasma folate was 86% and 89% respectively. These percentages differed by race, with 92% of CA women reaching NTD-protective concentrations of both RBC and plasma folate and 82% and 87% of AA women reaching NTD-protective concentrations of RBC folate and plasma folate. AA women were significantly less likely to have > 400 ng/mL RBC folate (P < 0.0001) and > 7 ng/mL plasma folate (P = 0.0184) or SP concentrations of plasma folate (P < 0.0001). SP concentrations of plasma folate were significantly less prevalent among women with lower educational status (P = 0.0482). The frequency of regular use of FA containing multi-vitamins and duration of their use were only higher among those with SP concentrations of plasma folate (P < 0.0001 and P = 0.0550, respectively). SP concentrations of plasma folate were significantly more prevalent among those reporting higher physical activity (P = 0.0361).
The mean plasma folate and RBC folate concentrations were significantly higher among those who reached NTD-protective concentrations of plasma and RBC folate and those who had SP concentrations of plasma folate (P < 0.0001 for all comparisons). None of the women had RBC folate concentrations ≤ 140 ng/mL. Only one woman (0.1%) had plasma folate ≤ 3 ng/mL. The percentage of the population that had SP concentrations of plasma folate was 27%. The proportion of women with SP concentrations of plasma folate was also significantly higher among those who reached NTD-protective concentrations of plasma and RBC folate, compared to those who did not. Among women who reached NTD-protective concentrations of RBC and plasma folate, 30.6% and 30% had SP concentrations of plasma folate, respectively. Whereas among women who didn't reach such concentrations, only 2.6% and 0% had SP concentrations of plasma folate, respectively (P < 0.0001 for both comparisons). Among supplement users who reached NTD-protective concentrations of RBC and plasma folate, however, 56% and 54% had SP concentrations of plasma folate (data not shown).
As shown in Table 2, in the regression model which included all women, AA women were significantly less likely to achieve NTD-protective concentrations of RBC and plasma folate (OR = 0.25, 95% CI = 0.15-0.43, P < 0.0001, OR = 0.50, 95% CI = 0.30-0.84, P = 0.0094, respectively) and SP concentrations of plasma folate (OR = 0.36, 95% CI = 0.25-0.53, P < 0.0001). Women with less than high school education were less likely to have SP concentrations of plasma folate (OR = 0.54, 95% CI = 0.34-0.88, P = 0.0123). Smokers were less likely to achieve NTD-protective concentrations of both RBC and plasma folate or have SP concentrations of plasma folate, but the results were only statistically significant for RBC folate (OR = 0.49, 95% CI = 0.31-0.79, P = 0.0031). Women who used hormonal contraceptives were more likely to have SP concentrations of plasma folate (OR = 1.45, 95% CI = 0.94-2.24, P = 0.0948). Women who used supplements for ≥ 1 year compared to NSUs were 3 times more likely to achieve NTD-protective concentrations of RBC folate (OR = 3.01, 95% CI = 0.91-9.99, P = 0.0722). Women who used supplements for < 1 year compared to NSUs were also ~3 times more likely to achieve NTD-protective concentrations of plasma folate (OR = 3.11, 95% CI = 1.11-8.77, P = 0.0315). Women who used supplements for < 1 year or ≥ 1 year compared to NSUs were ~2 and 5 times more likely to have SP concentrations of plasma folate (OR = 2.09, 95% CI = 1.32-3.33, P = 0.0018 and OR = 5.30, 95% CI = 3.04-9.26, P < 0.0001, respectively). Women with higher BMI (≥ 30 kg/m2) were more likely to achieve NTD-protective concentrations of both RBC and plasma folate and have SP concentrations of plasma folate, but the results were only statistically significant for RBC folate (OR = 1.74, 95% CI = 1.07-2.84, P = 0.0268). When BMI was replaced with WC and % BF, however, this association was non-significant with all cut points, NTD-protective concentrations of RBC and plasma folate or SP concentrations of plasma folate.
In the regression models which included only NSU, AA women were significantly less likely to achieve NTD-protective concentrations of RBC and plasma folate (OR = 0.22, 95% CI = 0.13-0.39, P < 0.0001, OR = 0.45, 95% CI = 0.26-0.78, P = 0.0044, respectively) and SP concentrations of plasma folate (OR = 0.37, 95% CI = 0.24-0.55, P < 0.0001) (Table 3). Among SU, AA women were less likely to achieve NTD-protective concentrations of RBC and plasma folate, but these associations were statistically non-significant (Table 4). AA women were significantly less likely to have SP concentrations of plasma folate even among SU (OR = 0.28, 95% CI = 0.10-0.79, P = 0.0164) (Table 4). Among NSU, smokers were less likely to achieve NTD-protective concentrations of both RBC and plasma folate or have SP concentrations of plasma folate, but the results were only statistically significant for RBC folate (OR = 0.43, 95% CI = 0.26-0.71, P = 0.0010) (Table 3). Among SU, smoking had no significant effect on achieving NTD-protective concentrations of both RBC and plasma folate or SP concentrations of plasma folate (Table 4). Among NSU, women with higher BMI (≥ 30 kg/m2) were more likely to achieve NTD-protective concentrations of both RBC and plasma folate, but the results only approached statistical significance for RBC folate (OR = 1.64, 95% CI = 0.96-2.79, P = 0.0686) (Table 3). Among SU, women with BMI ≥ 25 kg/m2 were more likely to achieve NTD-protective concentrations of both RBC and plasma folate, but the results only approached statistical significance for RBC folate (OR = 3.43, 95% CI = 0.82-14.28, P = 0.0902) (Table 4). When BMI was replaced with WC and % BF, however, none of the associations were statistically significant. Among SU, the duration of supplement use was not significantly associated with NTD-protective concentrations of RBC or plasma folate, but women who consumed supplements for ≥ 1 year were ~2 times more likely to have SP concentrations of plasma folate, the association approaching statistical significance (OR = 2.39, 95% CI = 0.98-5.84, P = 0.0568) (Table 4). Among NSU, women with less than high school education were less likely to have SP concentrations of plasma folate (OR = 0.51, 95% CI = 0.30-0.87, P = 0.0140) (Table 3). Among SU, those who used hormonal contraceptives were significantly more likely to have SP concentrations of plasma folate (OR = 8.11, 95% CI = 2.72-24.22, P = 0.0002) (Table 4).
Even though few public health interventions have been as far reaching as FA fortification programs, many concerns remain surrounding these programs and their projected impact on the prevention of NTDs. There is little doubt that the risk of NTD pregnancies can be reduced by raising FA consumption, but the magnitude of reduction for a given increase has been uncertain. Using 13 studies of FA supplementation on plasma folate concentrations and results from a cohort study of the risk of NTDs according to plasma folate, Wald et al. [26] documented that based on a typical western background plasma folate concentration of 5 ng/mL, ~0.2 mg/day fortification levels (current US level) is expected to reduce NTDs by ~20%. In fact, a 19% reduction in NTD birth prevalence has been reported following FA fortification of the US food supply [27]. An analysis of US data by race showed that statistically significant decreases in the prevalence of NTDs during fortification in the US occurred among CA women (~32% decline), but not among AA women (~13% decline) [28]. Although it is possible that the remaining NTD-affected pregnancies in AA women are not responsive to FA and may be associated with low vitamin B12 [29] or other factors, it is also possible that AA women are not reaching NTD-protective concentrations of circulating folate and therefore require higher folate intakes to prevent NTDs. Wald et al. [26] estimated that an increase of 0.4 mg, 1 mg and 5 mg of FA per day would reduce the NTD risk by 36%, 57% and 85% respectively, and suggested that FA fortification levels should be increased. However, on the other hand, even at the current lower level of US FA fortification, the program has been criticized for its potential adverse effects on other vulnerable population groups [15]. Further, it has been hypothesized that any lower decline in NTD prevalence will likely be due to the relatively low NTD prevalence in the general population in the pre FA fortification era, rather than to the current level of FA in fortified food, which argues against an increase in the fortification levels [30].
Studies evaluating folate status using appropriate biomarkers are critical for solving existing conflicts surrounding FA fortification programs, and making them more acceptable as well as justified for population wide coverage. The current study evaluated the folate status in a subset of women exposed to the US FA fortification program. Even though our study did not use a nationally representative sample of the US population, it represents 13% of US women with abnormal pap who are not only likely to encounter NTD pregnancies, and who are also at risk for developing cervical cancer and other HPV related cancers, as well as cancers common in this age group such as breast and ovarian cancer. The study was limited to women of child-bearing age, as the FA fortification was implemented specifically to improve the folate status in this subgroup. All women were diagnosed with abnormal pap, tested positive for HR-HPVs and were histologically confirmed to be free of pre-cancer, providing homogeneity in the study sample. Blood samples from these women were not requested to investigate possible folate deficiency or higher folate concentrations, and thus are unlikely to be biased toward low or high folate concentrations.
The study has several strengths because of the use of carefully selected biomarkers and biologically meaningful cut points. Most commonly used methods to measure circulating concentrations of folate are the microbiological assay, competitive binding assays or liquid chromatography-tandem mass spectrometry. The CDC previously reported that at least one competitive binding assay results in ~30% lower RBC folate values compared to competitive binding assays or liquid chromatography-tandem mass spectrometry [31], indicating that comparisons between studies need to be performed with caution. Comparisons between studies are further complicated by the fact that the same RBC folate cut off point is used regardless of the type of folate assay used. In our study, we used the microbiological assay to measure RBC folate and the NTD-protective RBC cut point used in our study, > 400 ng/mL or 906 nmol/L (400 × 2.266), was initially established by Daly et al. [5] using the same folate assay (microbiological). We also used the NTD-protective concentration of plasma folate cut point in addition to RBC folate cut point for the following reason: Even though RBC folate is considered a good indicator of the long-term folate status, it may be influenced by an individual's genetic makeup and the participation of adequate amounts of other methyl donor micronutrients, especially vitamin B2, vitamin B6 and vitamin B12, which influence reactions in the folate pathway [32]. We were able to observe similar results with both plasma and RBC data, and to our knowledge this approach has rarely been used in previous studies. Further, unlike the NHANES surveys, we used the same folate assay with the entire study population, avoiding the need to apply a correction factor to account for incorrect calibration issues. All of our folate assays were also performed by one research associate highly trained for this assay, further strengthening the quality of folate data used in our study.
Similar to a previous report [6], we observed that the US FA fortification has been very effective in eliminating folate deficiency in this population. In contrast to NHANES results reported for 1999-2000 [6], however, we observed that a much higher proportion (> 85%) of even NSUs reached NTD-protective concentrations of both plasma and RBC folate in our population. The differences in study populations, i.e., heterogeneous and nationally representative samples in NHANES vs. more homogeneous self-selected samples, or the longer period of exposure to the FA fortification program in our study population may explain the disagreement between our results and the NHANES results. Since 86% of our population did not use supplements containing FA, the most likely explanation for this discrepancy would be a higher consumption of folate-rich or fortified foods in our study population.
NHANES has also attempted to address whether there is a relationship between obesity and circulating concentrations of folate, an important question considering that > 50% of women of child-bearing age have excess body weight, but the results reported have been largely inconclusive. NHANES III in the pre-fortification era (1998-1994) and early post-fortification era (1999-2000) reported that obese adults compared to normal weight adults had higher odds of having low concentrations of serum folate [21,20]. A post-fortification NHANES which analyzed serum folate (2003-2008) and RBC folate (2007-2008) reported that BMI was inversely associated with serum folate among those who did not use supplements containing FA, but not among supplement users. This report also documented that regardless of supplement use, obese women had the highest RBC folate concentrations [33]. Our results suggest that obese women have higher odds of having NTD-protective concentrations of folate, and especially RBC folate, irrespective of supplement use and independent of several other covariates. Our results, therefore, do not support the notion that obese women in our population need to increase FA intake in comparison to non-obese women to confer a similar level of protection against NTDs.
In fact, our results suggest that most women in our study population may not need to take FA supplements in order to reach NTD-protective concentrations of circulating folate. However, in the regression analysis, we identified specific subgroups of the population that were significantly less likely to achieve NTD-protective concentrations of circulating folate. The most consistent finding was the racial disparities in achieving NTD-protective concentrations of folate. AA women who did not take FA containing supplements were 78% less likely to achieve > 400 ng/mL of RBC folate and 55% less likely to achieve > 7 ng/mL plasma folate. Previous studies have demonstrated that after the US fortification program, AA women of child-bearing age lagged behind CA women in total folate consumption and RBC folate concentrations largely due to differences in supplement use [34,35]. We observed that AA women were less likely to use FA containing supplements on a regular basis and have a shorter duration of use compared to CA women. Therefore, our results demonstrate the need for addressing racial disparities in folate status in the US post-FA fortification era, especially among AA women who do not take FA containing supplements on a regular basis. Our study also observed that smokers were significantly less likely to achieve NTD-protective concentrations of RBC folate, especially when they do not take FA containing supplements. Since the FA fortification has been estimated to result in a mean increase in FA intake that was approximately twice as large as previously projected [36], these remaining disparities in sub groups with regard to optimal NTD-protective effects of folate of this population should ideally be addressed by targeted individual improvements in folate intake.
A US post-FA fortification study conducted among CA women has shown that FA supplement use was a significant predictor of RBC folate concentrations [37]. In our study, the duration of supplement use was only weakly associated with achieving NTD-protective concentrations of plasma and RBC folate, suggesting that most women in our study population are able to achieve NTD-protective circulating concentrations of folate by consuming folate from natural or fortified sources. Even though women with SP levels of plasma folate were largely concentrated in the group of women who reached NTD-protective concentrations of folate (Table 1), the proportion of women with these high folate concentrations were only slightly higher than the US general population, except among SUs. AA women were significantly less likely to have SP concentrations of plasma folate, even among supplement users, suggesting that increasing folate intake with fortified food or FA is unlikely to result in hypothesized adverse effects of exposure to higher folate in AA women. We have previously reported that in a sub-set of the same study population, women with SP concentrations of plasma folate were at significantly lower risk of developing higher grades of cervical intraepithelial neoplasia (CIN 2+), especially in the presence of adequate plasma vitamin B12 [38], indicating that the monitoring of vitamin B12 status coupled with targeted FA interventions is a scenario that is unlikely to increase cancer risk. A recent meta-analysis of randomized clinical trials with FA doses ranging from 0.8 to 40 mg/day, even over an extended period (> 5 years), showed no increased risk of overall cancer incidence, cancer mortality or all-cause mortality [39], further suggesting that targeted folate interventions are unlikely to exert adverse effects. Further, FA is considered non-toxic across a range of recommended doses, 0.4 mg/day (for healthy adults) to 5,000 mg/day (for women with a history of NTD child). Our results also suggested that smokers were less likely to have SP concentrations of plasma folate, indicating that targeted interventions to increase folate in smokers are also unlikely to result in adverse effects. Women with less than high school education were less likely to have SP concentrations of plasma folate, but the level of education was not a significant determinant of NTD-protective concentrations of plasma or RBC folate, suggesting that targeted folate interventions based on education are unlikely to have significant influence on NTDs. Our results demonstrated that women who use hormonal contraceptives were significantly more likely to have SP concentrations of plasma folate, especially among SU. Even though a number of earlier studies have reported that contraceptives negatively impact folate status, presently available data do not support this conclusion [40]. The reduction in estrogen content in contraceptives over time [41], relatively lower negative effect of contraceptives on absorption of synthetic FA compared to natural folate [42] or the availability of contraceptive pills containing FA [43] may explain these observations. A recent randomized trial conducted with a folate-containing contraceptive documented a mean plasma folate concentration of 27 ng/mL at week 24, clearly exceeding SP levels [44]. Because folate containing contraceptives have become available only in recent years, longer term effects of this exposure are largely unknown, but this extended exposure needs careful monitoring. To our knowledge, our study is the first to report an association between contraceptive use and likelihood of having SP concentrations of plasma folate in an un-intervened population.
A notable limitation of the study is the smaller % of SUs in our study population, not allowing us to control for some variables (e.g. education) or forcing us to reduce the number of categories in variables (e.g. BMI) in models limited to SUs. Replication of findings observed in these models in a larger number of SUs will increase the scientific credibility of our findings. We also observed that the relationship between physical activity and folate concentrations were inconsistent between plasma and RBC folate. The smaller proportion of physically active women in our population may have contributed to these inconsistencies. Another limitation of the study is its cross-sectional nature, which did not allow us to establish causal inferences. Future studies that include comprehensive dietary folate intake data will complement circulating folate data and aid in implementing folate related policies in the US.
In conclusion, our results demonstrated that a large majority of women in our study population were able to reach NTD-protective concentrations of circulating folate with the current level of FA fortification. Remaining disparities in AA women and smokers should be addressed by targeted individual improvements in folate intake.
Figures and Tables
Table 1
The profile of the study population by demographic, lifestyle factors and NTD-protective concentrations of RBC and plasma folate and supra-physiologic concentrations of plasma folate (n = 843)1)
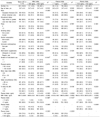
Table 2
The relationship between demographic and other factors with NTD-protective concentrations of RBC and plasma folate and supra-physiologic concentrations of plasma folate (n = 843)
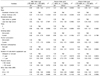
Table 3
The relationship between demographic and other factors with NTD-protective concentrations of RBC and plasma folate and supra-physiologic concentrations of plasma folate among non-supplement users (n = 724)
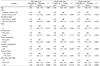
References
1. Werler MM, Shapiro S, Mitchell AA. Periconceptional folic acid exposure and risk of occurrent neural tube defects. JAMA. 1993; 269:1257–1261.


3. Centers for Disease Control and Prevention (CDC). Knowledge and use of folic acid by women of childbearing age-United States, 1995 and 1998. MMWR. 1999; 48:325–327.
4. Food and Drug Administration. Food standards: amendment of standards of identity for enriched grain products to require addition of folic acid. Final rule. 21 CFR parts 136, 137, and 139. Fed Regist. 1996; 61:8781–8807.
5. Daly LE, Kirke PN, Molloy A, Weir DG, Scott JM. Folate levels and neural tube defects. Implications for prevention. JAMA. 1995; 274:1698–1702.


6. Dietrich M, Brown CJ, Block G. The effect of folate fortification of cereal-grain products on blood folate status, dietary folate intake, and dietary folate sources among adult non-supplement users in the United States. J Am Coll Nutr. 2005; 24:266–274.


7. Caudill MA, Le T, Moonie SA, Esfahani ST, Cogger EA. Folate status in women of childbearing age residing in Southern California after folic acid fortification. J Am Coll Nutr. 2001; 20:129–134.


8. Brown RD, Langshaw MR, Uhr EJ, Gibson JN, Joshua DE. The impact of mandatory fortification of flour with folic acid on the blood folate levels of an Australian population. Med J Aust. 2011; 194:65–67.


9. Colapinto CK, O'Connor DL, Tremblay MS. Folate status of the population in the Canadian Health Measures Survey. CMAJ. 2011; 183:E100–E106.


10. Dowd JB, Aiello AE. Did national folic acid fortification reduce socioeconomic and racial disparities in folate status in the US? Int J Epidemiol. 2008; 37:1059–1066.


11. Bentley TG, Willett WC, Weinstein MC, Kuntz KM. Population-level changes in folate intake by age, gender, and race/ethnicity after folic acid fortification. Am J Public Health. 2006; 96:2040–2047.


12. Yang Q, Carter HK, Mulinare J, Berry RJ, Friedman JM, Erickson JD. Race-ethnicity differences in folic acid intake in women of childbearing age in the United States after folic acid fortification: findings from the National Health and Nutrition Examination Survey, 2001-2002. Am J Clin Nutr. 2007; 85:1409–1416.


13. Imhoff-Kunsch B, Flores R, Dary O, Martorell R. Wheat flour fortification is unlikely to benefit the neediest in Guatemala. J Nutr. 2007; 137:1017–1022.


14. Pfeiffer CM, Caudill SP, Gunter EW, Osterloh J, Sampson EJ. Biochemical indicators of B vitamin status in the US population after folic acid fortification: results from the National Health and Nutrition Examination Survey 1999-2000. Am J Clin Nutr. 2005; 82:442–450.


16. Ulrich CM, Potter JD. Folate supplementation: too much of a good thing? Cancer Epidemiol Biomarkers Prev. 2006; 15:189–193.


17. Morris MS, Jacques PF, Rosenberg IH, Selhub J. Folate and vitamin B12 status in relation to anemia, macrocyosis and cognitive impairment among older Americans in the age of folic acid fortification. Am J Clin Nutr. 2007; 85:193–200.


18. Yajnik CS, Deshpande SS, Jackson AA, Refsum H, Rao S, Fisher DJ, Bhat DS, Naik SS, Coyaji KJ, Joglekar CV, Joshi N, Lubree HG, Deshpande VU, Rege SS, Fall CH. Vitamin B12 and folate concentrations during pregnancy and insulin resistance in the offspring: the Pune Maternal Nutrition Study. Diabetologia. 2008; 51:29–38.


19. Kim YI. Will mandatory folic acid fortification prevent or promote cancer? Am J Clin Nutr. 2004; 80:1123–1128.


20. Mojtabai R. Body mass index and serum folate in childbearing age women. Eur J Epidemiol. 2004; 19:1029–1036.


21. Kimmons JE, Blanck HM, Tohill BC, Zhang J, Khan LK. Associations between body mass index and the prevalence of low micronutrient levels among US adults. MedGenMed. 2006; 8:59.
22. Ray JG, Wyatt PR, Vermeulen MJ, Meier C, Cole DE. Greater maternal weight and the ongoing risk of neural tube defects after folic acid flour fortification. Obstet Gynecol. 2005; 105:261–265.


23. Scott JM, Ghanta V, Herbert V. Trouble-free microbiologic serum and red cell folate assays. Am J Med Technol. 1974; 40:125–134.
24. Piyathilake CJ. Folate and vitamin B-12 status and chromosomal damage in the buccal mucosa of smokers and non-smokers [Ph.D. dissertation]. Birmingham (AL): University of Alabama;1993.
25. Piyathilake CJ, Macaluso M, Hine RJ, Richards EW, Krumdieck CL. Local and systemic effects of cigarette smoking on folate and vitamin B-12. Am J Clin Nutr. 1994; 60:559–566.


26. Wald NJ, Law MR, Morris JK, Wald DS. Quantifying the effect of folic acid. Lancet. 2001; 358:2069–2073.


27. Honein MA, Paulozzi LJ, Mathews TJ, Erickson JD, Wong LY. Impact of folic acid fortification of the US food supply on the occurrence of neural tube defects. JAMA. 2001; 285:2981–2986.


28. Williams LJ, Rasmussen SA, Flores A, Kirby RS, Edmonds LD. Decline in the prevalence of spina bifida and anencephaly by race/ethnicity: 1995-2002. Pediatrics. 2005; 116:580–586.


29. Ray JG, Wyatt PR, Thompson MD, Vermeulen MJ, Meier C, Wong PY, Farrell SA, Cole DE. Vitamin B12 and the risk of neural tube defects in a folic-acid-fortified population. Epidemiology. 2007; 18:362–366.


30. Heseker HB, Mason JB, Selhub J, Rosenberg IH, Jacques PF. Not all cases of neural-tube defect can be prevented by increasing the intake of folic acid. Br J Nutr. 2009; 102:173–180.


31. Fazili Z, Pfeiffer CM, Zhang M, Jain RB, Koontz D. Influence of 5,10-methylenetetrahydrofolate reductase polymorphism on whole-blood folate concentrations measured by LC-MS/MS, microbiologic assay, and bio-rad radioassay. Clin Chem. 2008; 54:197–201.


32. Mc Nulty H, Scott JM. Intake and status of folate and related B-vitamins: considerations and challenges in achieving optimal status. Br J Nutr. 2008; 99:Suppl 3. S48–S54.


33. Tinker SC, Hamner HC, Berry RJ, Bailey LB, Pfeiffer CM. Does obesity modify the association of supplemental folic acid with folate status among nonpregnant women of childbearing age in the United States? Birth Defects Res A Clin Mol Teratol. 2012; 94:749–755.


34. Bentley TG, Willett WC, Weinstein MC, Kuntz KM. Population-level changes in folate intake by age, gender, and race/ethnicity after folic acid fortification. Am J Public Health. 2006; 96:2040–2047.


35. Dowd JB, Aiello AE. Did national folic acid fortification reduce socioeconomic and racial disparities in folate status in the US? Int J Epidemiol. 2008; 37:1059–1066.


36. Choumenkovitch SF, Selhub J, Wilson PW, Rader JI, Rosenberg IH, Jacques PF. Folic acid intake from fortification in United States exceeds predictions. J Nutr. 2002; 132:2792–2798.


37. Brown JE, Jacobs DR Jr, Hartman TJ, Barosso GM, Stang JS, Gross MD, Zeuske MA. Predictors of red cell folate level in women attempting pregnancy. JAMA. 1997; 277:548–552.


38. Piyathilake CJ, Macaluso M, Alvarez RD, Bell WC, Heimburger DC, Partridge EE. Lower risk of cervical intraepithelial neoplasia in women with high plasma folate and sufficient vitamin B12 in the post-folic acid fortification era. Cancer Prev Res (Phila Pa). 2009; 2:658–664.


39. Clarke R, Halsey J, Lewington S, Lonn E, Armitage J, Manson JE, Bønaa KH, Spence JD, Nygård O, Jamison R, Gaziano JM, Guarino P, Bennett D, Mir F, Peto R, Collins R. B-Vitamin Treatment Trialists' Collaboration. Effects of lowering homocysteine levels with B vitamins on cardiovascular disease, cancer, and cause-specific mortality: meta-analysis of 8 randomized trials involving 37 485 individuals. Arch Intern Med. 2010; 170:1622–1631.


40. Wilson SM, Bivins BN, Russell KA, Bailey LB. Oral contraceptive use: impact on folate, vitamin B6, and vitamin B12 status. Nutr Rev. 2011; 69:572–583.


41. Gerstman BB, Gross TP, Kennedy DL, Bennett RC, Tomita DK, Stadel BV. Trends in the content and use of oral contraceptives in the United States, 1964-88. Am J Public Health. 1991; 81:90–96.


43. Holzgreve W, Pietrzik K, Koletzko B, Eckmann-Scholz C. Adding folate to the contraceptive pill: a new concept for the prevention of neural tube defects. J Matern Fetal Neonatal Med. 2012; 25:1529–1536.


44. Bart S Sr, Marr J, Diefenbach K, Trummer D, Sampson-Landers C. Folate status and homocysteine levels during a 24-week oral administration of a folate-containing oral contraceptive: a randomized, double-blind, active-controlled, parallel-group, US-based multicenter study. Contraception. 2012; 85:42–50.

