Abstract
Bamboo salt, a Korean folk medicine, is prepared with solar salt (sea salt) and baked several times at high temperatures in a bamboo case. In this study, we compared the preventive effects of bamboo salt and purified and solar salts on hepatic damage induced by carbon tetrachloride in Sprague-Dawley rats. Compared with purified and solar salts, bamboo salts prevented hepatic damage in rats, as evidenced by significantly reduced serum levels of aspartate aminotransferase, alanine aminotransferase, and lactate dehydrogenase (P < 0.05). Bamboo salt (baked 9×) triggered the greatest reduction in these enzyme levels. In addition, it also reduced the levels of the proinflammatory cytokines interleukin (IL)-6, interferon (IFN)-γ, and tumor necrosis factor (TNF)-α. Histopathological sections of liver tissue demonstrated the protective effect of bamboo salt, whereas sections from animals treated with the other salt groups showed a greater degree of necrosis. We also performed reverse transcription-polymerase chain reaction and western blot analyses of the inflammation-related genes iNOS, COX-2, TNF-α, and IL-1β in rat liver tissues. Bamboo salt induced a significant decrease (~80%) in mRNA and protein expression levels of COX-2, iNOS, TNF-α, and IL-1β, compared with the other salts. Thus, we found that baked bamboo salt preparations could prevent CCl4-induced hepatic damage in vivo.
Bamboo salt, as a medicinal food, originated in Korea and has been widely adopted throughout East Asia. Bamboo salt is prepared by placing solar salt into bamboo joint cases (approximately 8 cm diameter, 30 cm long) from plants less than three years old. The cases are sealed with natural red clay at both ends and then baked several times at 1,000℃-1,500℃ using pine as the combustible fuel [1,2]. Approximately 1,000 years ago, Korean medical doctors and monks began to prepare this medicinal salt by inserting sea (solar) salt in a thick bamboo stub and baking such stubs together with pine tree firewood. The ancient bamboo salt was baked only two or three times and then used in special medical treatments. After empirical testing, the doctors found that bamboo salt was most effective when baked at least nine times. They also found that the toxic characteristics of bamboo salt were removed if the salt was completely melted. Bamboo salt has been adopted as one of the most well-known traditional medical treatments, not only in Korea but also in many other Asian countries [3].
Numerous lines of evidence have implicated oxidative stress and inflammation in the etiology of liver diseases, cardiovascular diseases, and cancer [4,5]. As a result, carbon tetrachloride (CCl4), which produces reactive free radicals when metabolized, has been widely used as a solvent for induction of hepatic damage in animal models [6]. CCl4 increases lipid peroxidation in hepatic cells, and induces liver damage and necrosis [7].
Alanine aminotransferase (ALT) and aspartate aminotransferase (AST) are associated with inflammation and injury to liver cells, i.e., hepatocellular liver injury. Damage to the liver typically results in leakage of AST and ALT into the bloodstream [8]. AST is released into serum in proportion to cellular damage and is most elevated in the acute phase of cellular necrosis, whereas release of ALT occurs early in liver damage and remains elevated for a relatively longer period [9]. Lactate dehydrogenase (LDH) has also been used a biomarker for evaluation of the degree of liver injury [10]. Cytokines such as interleukin (IL)-6, interferon (IFN)-γ, and tumor necrosis factor (TNF)-α are small proteins that are produced and released from many cells under physiological and pathological stress, and the liver is highly susceptible to the action of these cytokines [11]. Acute hepatic damage is a temporary event, and is eventually resolved, thereby restoring normal liver histology and function. In fact, hepatotoxins may induce a stress response in hepatocytes with subsequent release of chemokines, followed by accumulation of inflammatory cells and subsequent hepatocellular damage [12]. Assays of serum levels of ALT, AST, LDH, and cytokines are standard approaches used for measurement of the extent of hepatic damage associated with such stress-induced pathology.
COX-2, iNOS, IL-lβ, and TNF-α genes in liver tissue could potentially be used as biomarkers for monitoring hepatic damage. Following inflammatory stimulation, both COX-2 and iNOS have been reported to induce deleterious effects in the liver [13]. Factors responsible for this hepatic response to inflammation include IL-lβ and TNF-α [12].
This study investigated the potential hepatoprotective effects of bamboo salt against CCl4-induced hepatic damage in male Sprague-Dawley (SD) rats. Serum levels of AST, ALT, LDH, triglyceride (TG), total cholesterol (TC), and inflammatory cytokines (TNF-α, IFN-γ, and IL-6) were measured in order to monitor liver damage. The extent of liver damage was also analyzed through histopathological observations. The mRNA and protein expressions of the inflammation-related genes iNOS, COX-2, TNF-α, and IL-1β in liver tissue were also determined by reverse transcription-polymerase chain reaction (RT-PCR) assay and western blot analysis, respectively.
Bamboo salts (baked 1× and 9×) and solar salt were provided by Taesung Food Company (Gochang, Jeonbuk, South Korea). Purified salt manufactured by Hanju Corporation (Ulsan, South Korea) was purchased from a local market (Jangjeon-dong, Geumjeong-gu, Busan, South Korea). The salts were dissolved in sterilized distilled water at a concentration of 20% before the experiments were performed.
Seven-week-old male SD rats (n = 60) were purchased from Hyochang Science (Daegu, South Korea). They were maintained in a temperature-controlled facility (temperature, 23℃±1℃; relative humidity, 50%±5%) with a 12-h light/dark cycle and free access to water and a standard rat diet [14].
To investigate the preventive effects of various salts against CCl4-induced hepatic damage, the animals were divided into six groups of 10 rats each. The experimental design was as follows: in the normal group, distilled water was administered for 14 days, along with a single dose of vehicle (2 mL/kg body weight [b.w.] of olive oil, p.o.); animals in the CCl4 control group received repeated oral administration of distilled water for 14 days, followed by a single administration of CCl4 (2 mL/kg b.w.; CCl4:olive oil = 1:1, v/v) on the last day for induction of liver damage. In the "salts + CCl4" groups, a dose of 400 mg/kg b.w. salt dissolved in water was administered for two weeks, and hepatic damage was induced as mentioned above. Twenty four hours after administration of CCl4, the rats were anaesthetized using carbon dioxide and sacrificed [14]. Blood and livers were collected and preserved at -70℃ until biological assays were performed. These experiments followed a protocol approved by the Animal Ethics Committee of Pusan National University (PNU-2011-000409; Busan, South Korea).
AST and ALT levels in serum were determined using commercially available kits (Asan Pharm, Seoul, South Korea). LDH levels in serum were also determined using a specific commercially available kit (Cayman Chemical Co., Ann Arbor, MI, USA)
TG and TC levels in serum were determined using commercially available kits (Asan Pharm, Seoul, South Korea).
For serum cytokine assay, blood from the inferior vena cava was collected into a tube and centrifuged (3,000 rpm, 10 min, 4℃). Concentrations of proinflammatory-related cytokines (IL-6, IFN-γ, and TNF-α) were measured using an ELISA assay according to the manufacturer's protocol (Biolegend ELISA MAX™ Deluxe kits; Biolegend, San Diego, CA, USA).
For histological investigations, liver tissues were fixed in 10% (v/v) buffered formalin for 24 h, dehydrated in ethanol, and then embedded in paraffin. Then, 4-µm-thick sections were prepared and stained with hematoxylin and eosin (H&E) for observation under an Olympus BX41 microscope (Olympus, Tokyo, Japan).
Total RNA was isolated from liver tissue using Trizol reagent (Invitrogen, Carlsbad, CA, USA) according to the manufacturer's recommendations. RNA was digested with RNase-free DNase (Roche, Basel, Switzerland) for 15 min at 37℃ and purified using an RNeasy kit (Qiagen, Hilden, Germany) according to the manufacturer's protocol. cDNA was synthesized from 2 µg of total RNA by incubating at 37℃ for l h with AMV reverse transcriptase (GE Healthcare, Little Chalfont, UK) with random hexanucleotides, according to the manufacturer's instructions [15].
Primers used for specific amplification of the genes of study were as follows: 5'-AGA GAG ATC GGG TTC ACA-3' (forward) and 5'-CAC AGA ACT GAG GGT ACA-3' (reverse) for iNOS; 5'-TTA AAA TGA GAT TGT CCG AA-3' (forward) and 5'-AGA TCA CCT CTG CCT GAG TA-3' (reverse) for COX-2; 5'-GAC CCT CAG ACT CAG ATC ATC CTT CT-3' (forward) and 5'-ACG CTG GCT CAG CCA CTC-3' (reverse) for TNF-α; and 5'-CTC CAT GAG CTT TGT ACA AGG-3' (forward) and 5'-TGC TGA TGT ACC AGT TGG GG-3' (reverse) for IL-1β. The internal control gene of GAPDH was amplified using the following primers: 5'-CGG AGT CAA CGG ATT TGG TC-3' (forward) and 5'-AGC CTT CTC CAT GGT CGT GA-3' (reverse). Amplification was performed in a thermal cycler (Eppendorf, Hamburg, Germany) with cycles of denaturation. The amplified PCR products were run on 1.0% agarose gels and visualized by ethidium bromide staining.
Total protein was obtained using RIPA buffer as described by Zhao et al. [16]. Protein concentrations were determined using a Bio-Rad protein assay kit (Hercules, CA, USA). For western blot analysis, aliquots of the lysate containing 30-50 µg of protein were separated by sodium dodecyl sulfate-polyacrylamide gel electrophoresis (SDS) and then electrotransferred onto a nitrocellulose membrane (Schleicher and Schuell, Keene, NH, USA). Antibodies against iNOS, COX-2, TNF-α, and IL-1β were obtained from Santa Cruz Biotechnology, Inc. (Santa Cruz, CA, USA), and then incubated with the horseradish peroxidase-conjugated secondary antibody (Santa Cruz Biotechnology Inc.) for 1 h at room temperature. Blots were washed three times with PBS-T and then developed with enhanced chemiluminescence (Amersham Life Science, Arlington Heights, IL, USA).
Data are expressed as the mean ± SD. Differences between the mean values for individual groups were assessed by one-way ANOVA with Duncan's multiple range tests. Differences were considered significant when P < 0.05. The SAS v9.1 statistical software package (SAS Institute Inc., Cary, NC, USA) was used for the analysis.
The AST level of normal rats was 169.5 ± 12.5 IU/L; however, that of CCl4 control rats was significantly increased to 1,693.8 ± 112.1 IU/L. Levels of AST in rats fed with purified and solar salt were 1,553.1 ± 62.0 and 1,285.3 ± 163.0 IU/L, respectively. Levels of AST in rats treated with bamboo salt baked 1× and 9 × were decreased to 1,077.0 ± 111.4 and 1,038.1 ± 77.8 IU/L (43.0% inhibition), respectively, which was lower than the levels in rats treated with purified and solar salts (Fig. 1A).
The ALT level in the normal group was 45.7 ± 6.5 IU/L, whereas that in the control group was 1,438.2 ± 214.0 IU/L, indicating a marked increase. ALT levels in purified and solar salt groups showed a decrease to 998.4 ± 93.4 and 804.9 ± 152.4 IU/L, respectively. Treatment with bamboo salt resulted in even more decreased levels of ALT. The more thoroughly baked bamboo salt (9×) showed a lower ALT level, i.e. 634.4 ± 38.3 IU/L (57.7% inhibition), whereas the level in the 1× baked bamboo salt group was 790.2 ± 16.5 IU/L (Fig. 1B). Thus, from the results for serum levels of AST and ALT, it was confirmed that salts generally inhibited hepatic damage induced by CCl4, although the 9× baked bamboo salt showed the best effect.
Levels of LDH in the purified and solar salt groups were 5,875.2 ± 298.6 and 5,432.9 ± 388.7 IU/L, respectively, and were slightly lower than the level in the control group (6,742.6 ± 524.5 IU/L). However, LDH levels in the bamboo salt (baked 1× and 9×) groups were 4,776.4 ± 247.6 and 3,877.8 ± 125.2 IU/L (51.4% inhibition), respectively, whereas the normal group showed the lowest level of LDH, i.e. 1,173.5 ± 105.2 IU/L (Fig. 1C). LDH levels in the salt groups were lower than those in the control group, similar to the findings for AST and ALT levels.
Serum TG levels in rats fed with different kinds of salt were higher than those in rats in the normal group. We found that treatment with bamboo salt (baked 9×) resulted in significantly lowered levels of TG, and these findings were statistically similar to those of the normal group. On the other hand, TC levels were low in all salt-treated groups. TC levels in rats in the control group were significantly lower than those in rats in the sample groups and normal group, whereas those of the 9× bamboo salt group were significantly higher (P < 0.05) (Fig. 2). Serum TC levels in CCl4-treated rats were significantly lower than those in control rats, and bamboo salt (baked 9×) showed the largest reversal of this effect among the sample groups.
Serum IL-6, IFN-γ, and TNF-α levels in rats in the bamboo salt-treated groups (baked 1× and 9×) were significantly lower than those in the control and purified and solar salt groups (Fig. 3). Reductions in levels of IL-6 and IFN-γ in the bamboo salt group (9×) were 34.6% and 39.9%, respectively, compared with the control group. TNF-α levels in rats treated with bamboo salt (9×) also decreased by 18.5%. However, the levels of these proinflammatory cytokines in rats treated with purified salt were similar to those in rats in the control group.
H&E staining showed CCl4-induced histopathological changes in the liver, with significant degeneration and necrosis of hepatocytes in the centrilobular region and perivenular inflammatory infiltrates. Histological tissue sections of rats in the normal group showed normal histological morphology of liver tissue samples. However, histopathological evaluation showed indications of hepatic damage in all CCl4-treated rats (Fig. 4). Sections of control rats exhibited a normal organizational structure, with no sign of inflammation. Sections from rats treated with CCl4 showed widespread areas of congestion and hemorrhage in the centrilobular zone, and necrosis involving many hepatocytes was observed in this area. The purified salt group showed a mild hepatic damage of grade 3, indicating that it had little ability to limit hepatic damage, compared with the control group. Sections from rats treated with solar salt showed moderate congestion and hemorrhage of the area around the centrilobular vein, which extended to the midzonal cells, and most lobules were affected. Areas of confluent necrosis were limited to liver cells surrounding the centrilobular vein. Bamboo salt (baked 1×) exerted a better anti-inflammatory effect, compared with solar salt, as only mild hepatic damage (grade 2) was observed. Tissue sections from the 9× baked bamboo salt group appeared to provide greater protection from liver damage. The liver showed minimal congestion and necrosis of single hepatocytes, limited to the area immediately around the centrilobular vein; indeed, many lobules were not affected at all. In addition, a greater anti-inflammatory effect was observed for the 9× baked bamboo salt, compared with the 1× baked bamboo salt.
RT-PCR and western blot analyses were performed for investigation of whether the inhibitory effect of the salts on inflammation was due to transcriptional regulation of inflammatory mediators such as iNOS, COX-2, TNF-α, and IL-1β in the liver. As shown in Figs. 5 and 6, treatment with CCl4 resulted in significantly increased levels of mRNA and protein of these inflammatory mediators. However, bamboo salt (baked 9×) induced a significant decrease in mRNA and protein expressions of COX-2, iNOS, TNF-α, and IL-1β (P < 0.05). Liver tissues from bamboo salt (baked 1×)-treated rats showed decreased expression of these genes compared with the purified and solar salt groups.
AST and ALT are enzymes located in liver cells that leak out into the general circulation when liver cells are injured. AST is found in many body tissues, including heart, muscles, kidneys, brain, and lungs. ALT was found predominately in the liver, while lesser quantities were found in kidneys, heart, and skeletal muscles [17]. LDH is an enzyme found in many body tissues, including the liver, and elevated levels of LDH may indicate liver damage. AST and ALT levels have also been used for evaluation of the hepatoprotective activities of medicinal plants in CCl4-induced hepatic damage in animal models [18-20]. In this study, we used a salt concentration of 400 mg/kg for oral administration in rats. Bamboo salt was safe and did not exhibit any toxic effects at oral administration levels of 0.01-1 g/kg [2]. A dose of 400 mg/kg administered to a rat is approximately equivalent to 4 g/day for a 60 kg adult human [21], calculated by a conversion rate of 6.3 [22]. Therefore, this level of bamboo salt supplied in a daily diet may have hepatoprotective effects. Raju et al. [23] reported that serum AST and ALT levels in CCl4-treated rats showed a remarkable increase, compared with rats in the normal group, indicating that liver damage was significantly induced by CCl4. Multiple pathological insults render the liver more susceptible to bacterial infections. Because salt has a strong sterilization effect [24], this activity might help to alleviate bacterial infection and decrease the severity of liver injury. Following repeated baking cycles, bamboo salt contains more hydroxyl groups, which may also increase its protective effect in liver damage because of its enhanced antioxidant activity [25]. Although TG levels did not show correlation with hepatic damage in other studies [26], a previous study suggested an association of the serum TC level with hepatic damage, as reflected by monitoring AST, ALT, and LDH, although it was not found to be the best indicator [27]. When cholesterol levels are high in the bloodstream, excess LDL begins to seep into the inner wall of the artery. This triggers an inflammatory response, which actually speeds up accumulation of cholesterol in the artery wall. This in turn produces more inflammation [28].
Serum cytokine (IL-6, TNF-α, IL-1β, IFN-γ, etc.) levels in patients with inflammatory diseases are higher than those in healthy people [29]. Thus, lower levels of IL-6, IFN-γ, and TNF-α are indicative of improved anti-inflammatory effects, and baked bamboo salt (9×) showed the best protective effect against hepatic damage. Hepatocytes bear a variety of cytokine receptors. Inflammatory cytokines of IL-6, IFN-γ, and TNF-α play a pathogenic role in liver disease [30]. Although systemic IL-6 levels are elevated after traumatic hemorrhage, hepatocellular function is impaired and liver injury occurs [31]. IFN-γ increases such injury through stimulation of hepatic inflammation and aggravation of liver damage [32]. TNF-α is also a key mediator in many experimental liver injury models [33,34].
Histopathology is an important clinical standard for diagnosis of hepatic damage [35]. In addition, histopathological examination of rat liver sections has been reported as an effective method for evaluation of hepatoprotective activity in a CCl4-induced rat hepatic damage model [36]. From the sections examined, the 9×baked bamboo salt exerted a preventive effect against CCl4-induced hepatic damage. As mentioned above, bamboo salt exerts both antioxidative and anticancer effects [37]. Compounds with antioxidant properties may also have anti-inflammatory effects and can actually prevent activation of inflammatory signals [38]. Solar salt appears to be more effective than the purified salt used in our previous studies [16,25,39]. Solar salt also contains higher concentrations of minerals such as Ca, K, and Mg than purified salt. Due to significant differences in mineral contents, solar salt showed less co-mutagenicity and induced apoptosis in HCT-116 colon cancer cells, regulating expression of apoptosis-related genes, including Bcl-2 and Bax [39]. Bamboo salt is known to contain higher concentrations of minerals and other antioxidant compounds than both solar and purified salt. In particular, the nine-time baked bamboo salt was found to contain more K, Ca, Mg, and Mn than purified and solar salts. In addition, a higher content of OH- in bamboo salt resulted in a pH of 11.04, while the pH of purified and solar salts was 6.29 and 9.13, respectively. Finally, bamboo salt is more alkaline and shows higher antioxidative activity than purified and solar salts [25]. Zhao et al. [16] described the anticancer activity of bamboo salt using HCT-116 colon cancer cells. A 1% salt concentration of bamboo salt (nine-time baked) suppressed growth of cancer cells by 53%, while suppression by purified and solar salt was only 18% and 22%, respectively. In addition, one- and three-time baked bamboo salt achieved suppression by 41% and 44%, respectively. In this study, the functionality of solar salt was superior to that of purified salt, although inferior to that of bamboo salt. For this reason, we focused on the functionality of bamboo salt. Mechanistic studies have demonstrated that bamboo salt significantly promoted the expression of apoptosis-related genes (Bax, caspase-3, and caspase-9) and suppressed anti-apoptotic (Bcl-2) and inflammatory genes (NF-κB, iNOS, and COX-2). The higher mineral content and antioxidative property of bamboo salt could show a superior anti-inflammatory action, compared with purified and solar salts. The unique combination of minerals and antioxidant activity may contribute to the efficacy of bamboo salt in prevention of hepatic damage and inflammation.
In the inflammatory process, activated inflammatory cells (neutrophils, eosinophils, mononuclear phagocytes, and macrophages) secrete increased amounts of nitric oxide, prostaglandin E2, and cytokines such as IL-1β, IL-6, and TNF-α [40]. These substances not only induce cell and tissue damage but also activate macrophages in chronic hepatitis [41]. Minerals such as potassium, calcium, and magnesium, which are abundant in bamboo salts, appear to have anti-inflammatory effects. Levels of potassium, calcium, and magnesium in blood have shown correlation with severity of liver disease [42]. Potassium has potent anti-inflammatory effects, low calcium levels are associated with hepatic disease, and supplementation with magnesium in the appropriate context can be used for treatment of hepatitis [43,44]. The antioxidative effect of the abundant OH groups in bamboo salt prevents hepatic damage. Together, these characteristics of bamboo salt contribute to its anti-inflammatory activity in the liver.
Findings of this study demonstrate that bamboo salt is effective in prevention of CCl4-induced hepatic damage in SD rats. According to our results, the hepatoprotective effects of bamboo salt may be due to decreased serum levels of AST, ALT, LDH, and proinflammatory cytokines such as TNF-α, IL-6, and IFN-γ. Histopathological studies have also shown that the 9× baked bamboo salt could prevent CCl4-induced inflammation in the liver. In addition, mRNA and protein expression levels of inflammation-related genes such as iNOS, COX-2, TNF-α, and IL-1β in the liver were significantly reduced in 9× baked bamboo salt-treated rats (P < 0.05). These results suggest the potential usefulness of bamboo salts in treatment or prevention of chemical-induced hepatic damage in vivo.
Figures and Tables
Fig. 1
Effect of various salt samples on serum aspartate aminotransferase (AST), alanine aminotransferase (ALT), and lactate dehydrogenase (LDH) levels in CCl4-induced hepatic damage (n = 10 Sprague-Dawley rats in each group, 400 mg/kg b.w. of salt). P, purified salt; S, solar salt; BS, bamboo salt. Mean values with different letters over the bars indicate significant differences (P < 0.05) according to Duncan's multiple range test.
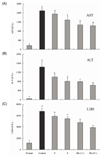
Fig. 2
Effect of salt samples on serum triglyceride (TG) and total cholesterol (TC) levels in CCl4-induced hepatic damage (n = 10 Sprague-Dawley rats in each group, 400 mg/kg b.w. of salt). P, purified salt; S, solar salt; BS, bamboo salt. Mean values with different letters over the bars indicate significant differences (P < 0.05) according to Duncan's multiple range test.
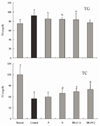
Fig. 3
Effect of salt samples on serum IL-6, IFN-γ, and TNF-α levels in CCl4-induced hepatic damage (n = 10 Sprague-Dawley rats in each group, 400 mg/kg b.w. of salt). P, purified salt; S, solar salt; BS, bamboo salt. Mean values with different letters over the bars indicate significant differences (P < 0.05) according to Duncan's multiple range test.
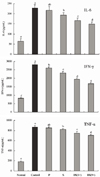
Fig. 4
Histology images of liver tissues in rats with CCl4-induced hepatic damage (×200 magnification).
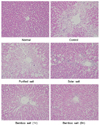
Fig. 5
Effects of bamboo salt (400 mg/kg b.w.) on mRNA expression levels of iNOS, COX-2, TNF-α, and IL-1β in liver tissues of rats treated with CCl4. P, purified salt; S, solar salt; BS, bamboo salt. The intensity of the band was measured by densitometry and expressed as fold ratios compared with the control. Fold ratio: gene expression/GAPDH × control numerical value (control fold ratio: 1). Mean values with different letters over the bars indicate significant differences (P < 0.05) according to Duncan's multiple range test.
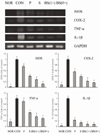
Fig. 6
Effects of bamboo salt (400 mg/kg b.w.) on protein expression levels of iNOS, COX-2, TNF-α, and IL-1β in liver tissues of rats treated with CCl4. P, purified salt; S, solar salt; BS, bamboo salt. The intensity of the band was measured using a densitometer and expressed as fold ratios compared with the control. Fold ratio: gene expression/GAPDH × control numerical value (control fold ratio: 1). Mean values with different letters over the bars indicate significant differences (P < 0.05) according to Duncan's multiple range test.
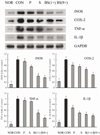
References
1. Hu C, Zhang Y, Kitts DD. Evaluation of antioxidant and prooxidant activities of bamboo Phyllostachys nigra var. Henonis leaf extract in vitro. J Agric Food Chem. 2000; 48:3170–3176.


2. Shin HY, Na HJ, Moon PD, Seo SW, Shin TY, Hong SH, Lee KN, Park RK, Kim HM. Biological activity of bamboo salt. Food Ind Nutr. 2004; 9:36–45.
3. Shin HY, Lee EH, Kim CY, Shin TY, Kim SD, Song YS, Lee KN, Hong SH, Kim HM. Anti-inflammatory activity of Korean folk medicine purple bamboo salt. Immunopharmacol Immunotoxicol. 2003; 25:377–384.


4. Cauwels A, Brouckaert P. Survival of TNF toxicity: dependence on caspases and NO. Arch Biochem Biophys. 2007; 462:132–139.


5. Seitz HK, Stickel F. Risk factors and mechanisms of hepatocarcinogenesis with special emphasis on alcohol and oxidative stress. Biol Chem. 2006; 387:349–360.


6. Rechnagel RO, Glende EA Jr. Carbon tetrachloride hepatotoxicity: an example of lethal cleavage. CRC Crit Rev Toxicol. 1973; 2:263–297.


7. Weber LW, Boll M, Stampfl A. Hepatotoxicity and mechanism of action of haloalkanes: carbon tetrachloride as a toxicological model. Crit Rev Toxicol. 2003; 33:105–136.


8. Raoul JL, Bruix J, Greten TF, Sherman M, Mazzaferro V, Hilgard P, Scherubl H, Scheulen ME, Germanidis G, Dominguez S, Ricci S, Nadel A, Moscovici M, Voliotis D, Llovet JM. Relationship between baseline hepatic status and outcome, and effect of sorafenib on liver function: SHARP trial subanalyses. J Hepatol. 2012; 56:1080–1088.


9. Pal M, Ghosh M. Studies on comparative efficacy of α-linolenic acid and α-eleostearic acid on prevention of organic mercury-induced oxidative stress in kidney and liver of rat. Food Chem Toxicol. 2012; 50:1066–1072.


10. Small DM, Gobe GC. Cytochrome c: potential as a noninvasive biomarker of drug-induced acute kidney injury. Expert Opin Drug Metab Toxicol. 2012; 8:655–664.


13. Suliburk JW, Helmer KS, Gonzalez EA, Robinson EK, Mercer DW. Ketamine attenuates liver injury attributed to endotoxemia: role of cyclooxygenase-2. Surgery. 2005; 138:134–140.


14. Park CM, Youn HJ, Chang HK, Song YS. TOP1 and 2, polysaccharides from Taraxacum officinale, attenuate CCl4-induced hepatic damage through the modulation of NF-κB and its regulatory mediators. Food Chem Toxicol. 2010; 48:1255–1261.


15. Zhao X, Deng X, Park KY, Qiu L, Pang L. Purple bamboo salt has anticancer activity in TCA8113 cells in vitro and preventive effects on buccal mucosa cancer in mice in vivo. Exp Ther Med. 2013; 5:549–554.


16. Zhao X, Kim SY, Park KY. Bamboo salt has in vitro anticancer activity in HCT-116 cells and exerts anti-metastatic effects in vivo. J Med Food. 2013; 16:9–19.


17. Delić R, Štefanović M. Optimal laboratory panel for predicting preeclampsia. J Matern Fetal Neonatal Med. 2010; 23:96–102.


18. Li R, Guo W, Fu Z, Ding G, Zou Y, Wang Z. Hepatoprotective action of Radix Paeoniae Rubra aqueous extract against CCl4-induced hepatic damage. Molecules. 2011; 16:8684–8694.


19. Ranawat L, Bhatt J, Patel J. Hepatoprotective activity of ethanolic extracts of bark of Zanthoxylum armatum DC in CCl4 induced hepatic damage in rats. J Ethnopharmacol. 2010; 127:777–780.


20. Surendran S, Eswaran MB, Vijayakumar M, Rao CV. In vitro and in vivo hepatoprotective activity of Cissampelos pareira against carbon-tetrachloride induced hepatic damage. Indian J Exp Biol. 2011; 49:939–945.
22. U.S. Food and Drug Administration [Internet]. Guidance for Industry, Estimating the Maximum Safe Starting Dose in Initial Clinical Trials for Therapeutics in Adult Healthy Volunteers. Silver Spring (MD): U.S. Food and Drug Administration;2005. cited 2013 February 01. Available from: http://www.fda.gov/downloads/Drugs/GuidanceComplianceRegulatoryInformation/Guidances/ucm078932.pdf.
23. Raju K, Anbuganapathi G, Gokulakrishnan V, Rajkapoor B, Jayakar B, Manian S. Effect of dried fruits of solanum nigrum LINN against CCl4-induced hepatic damage in rats. Biol Pharm Bull. 2003; 26:1618–1619.


24. Segner WP, Schmidt CF, Boltz JK. Effect of sodium chloride and pH on the outgrowth of spores of type E Clostridium botulinum at optimal and suboptimal temperatures. Appl Microbiol. 1966; 14:49–54.


25. Zhao X, Jung OS, Park KY. Alkaline and antioxidant effects of bamboo salt. J Korean Soc Food Sci Nutr. 2012; 41:1301–1304.


26. Tong Y, Yan D, Gao S. Study on sensitive indexes of acute liver damage induced by carbon tetrachloride. Chin J Food Hyg. 2000; 12:10–11.
27. Wang MY, Anderson G, Nowicki D, Jensen J. Hepatic protection by noni fruit juice against CCl4-induced chronic liver damage in female SD rats. Plant Foods Hum Nutr. 2008; 63:141–145.


28. Ridker PM, Rifai N, Pfeffer MA, Sacks FM, Moye LA, Goldman S, Flaker GC, Braunwald E. Cholesterol and Recurrent Events (CARE) Investigators. Inflammation, pravastatin, and the risk of coronary events after myocardial infarction in patients with average cholesterol levels. Circulation. 1998; 98:839–844.


29. Gratacós J, Collado A, Filella X, Sanmartí R, Cañete J, Llena J, Molina R, Ballesta A, Muñoz-Gómez J. Serum cytokines (IL-6, TNF-α, IL-1β and IFN-γ) in ankylosing spondylitis: a close correlation between serum IL-6 and disease activity and severity. Br J Rheumatol. 1994; 33:927–931.


30. Wang JY, Wang XL, Liu P. Detection of serum TNF-α, IFN-γ, IL-6 and IL-8 in patients with hepatitis B. World J Gastroenterol. 1999; 5:38–40.


31. Toth B, Yokoyama Y, Schwacha MG, George RL, Rue LW 3rd, Bland KI, Chaudry IH. Insights into the role of interleukin-6 in the induction of hepatic injury after trauma-hemorrhagic shock. J Appl Physiol. 2004; 97:2184–2189.


32. Knight B, Lim R, Yeoh GC, Olynyk JK. Interferon-γ exacerbates liver damage, the hepatic progenitor cell response and fibrosis in a mouse model of chronic liver injury. J Hepatol. 2007; 47:826–833.


33. Bradham CA, Plümpe J, Manns MP, Brenner DA, Trautwein C. Mechanisms of hepatic toxicity. I. TNF-induced liver injury. Am J Physiol. 1998; 275:G387–G392.
34. Schümann J, Tiegs G. Pathophysiological mechanisms of TNF during intoxication with natural or man-made toxins. Toxicology. 1999; 138:103–126.


35. Girish C, Koner BC, Jayanthi S, Rao KR, Rajesh B, Pradhan SC. Hepatoprotective activity of six polyherbal formulations in CCl4-induced liver toxicity in mice. Indian J Exp Biol. 2009; 47:257–263.
36. Manjrekar AP, Jisha V, Bag PP, Adhikary B, Pai MM, Hegde A, Nandini M. Effect of Phyllanthus niruri Linn. treatment on liver, kidney and testes in CCl4 induced hepatotoxic rats. Indian J Exp Biol. 2008; 46:514–520.
37. Zhao X, Song J, Lee JH, Kim SY, Park KY. Antioxidation and cancer cell (HT-29) antiproliferation effects of Rubus coreanus Miquel bamboo salt. Cancer Prev Res. 2010; 15:306–312.
38. Halliwell B. Antioxidants: the basics-what they are and how to evaluate them. Adv Pharmacol. 1997; 38:3–20.


39. Zhao X, Kim SH, Qi YC, Kim SY, Park KY. Effects of different kinds of salt in the comutagenicity and growth of cancer cells. J Korean Soc Food Sci Nutr. 2012; 41:26–32.


40. Karlsen M, Hovden AO, Vogelsang P, Tysnes BB, Appel S. Bromelain treatment leads to maturation of monocyte-derived dendritic cells but cannot replace PGE2 in a cocktail of IL-1β, IL-6, TNF-α and PGE2. Scand J Immunol. 2011; 74:135–143.


41. Nan B, Lin P, Lumsden AB, Yao Q, Chen C. Effects of TNF-α and curcumin on the expression of thrombomodulin and endothelial protein C receptor in human endothelial cells. Thromb Res. 2005; 115:417–426.


42. Mills PR, Shenkin A, Anthony RS, McLelland AS, Main AN, MacSween RN, Russell RI. Assessment of nutritional status and in vivo immune responses in alcoholic liver disease. Am J Clin Nutr. 1983; 38:849–859.

