Abstract
The anti-obesity effects of a hot water extract from wasabi (Wasabia japonica Matsum.) leaves (WLE), without its specific pungent constituents, such as allyl-isothiocyanate, were investigated in high fat-diet induced mice. C57J/BL mice were fed a high-fat diet (control group) or a high-fat diet supplemented with 5% WLE (WLE group). Physical parameters and blood profiles were determined. Gene expression associated with lipid metabolism in liver and white adipose tissue were analyzed. After 120 days of feeding, significantly lower body weight gain, liver weight and epididymal white adipose tissue weight was observed in the WLE group compared to the control group. In liver gene expression within the WLE group, PPARα was significantly enhanced and SREBP-1c was significantly suppressed. Subsequent downstream genes controlled by these regulators were significantly suppressed. In epididymal white adipose tissue of the WLE group, expression of leptin, PPARγ, and C/EBPα were significantly suppressed and adiponectin was significantly enhanced. Acox, related to fatty acid oxidization in adipocytes, was also enhanced. Our results demonstrate that the WLE dietary supplement induces mild suppression of obesity in a high-fat diet induced mice, possibly due to suppression of lipid accumulation in liver and white adipose tissue.
In recent decades, the prevalence of obesity has become an urgent world-wide public health problem [1]. Obesity, attributed to an imbalance between energy intake and energy expenditure, is closely associated with lifestyle-related diseases (such as diabetes mellitus and dyslipidemia), which are included among primary risk factors for cardiovascular disease [2]. Prevention of obesity should naturally reduce the risks of developing such diseases.
Obesity is mainly characterized at cell biological levels by an increase in the size of adipocytes differentiated from preadipocytes, and in animal experiments by body weight gain and increase of fat tissue through conversion of excess energy to lipids and accumulation as triglycerides. Obesity prevention trials have attempted to determine whether certain food components have the ability to suppress intracellular lipid accumulation. For example, tea catechin is reportedly effective in inhibition of adipogenesis in 3T3-L1 cells [3] and in vivo studies have demonstrated that oral administration of green tea can result in a decrease in weight of adipose tissue in rats and mice [4,5]. The same suppressive effects on 3T3-L1 or C3H10T1/2 B2C1 adipocyte differentiation have also been demonstrated with mioga extract (Zingiber mioga Rosc.) [6], red yeast rice extract [5], flavonoids [6,7] and mushroom extracts [8]. In recent years, a large range of polyphenolic compounds occurring ubiquitously in edible plants (fruits, vegetables, and herbs) and plant-derived beverages have received considerable attention due to their biological activities. These compounds are believed to possess health-promoting properties.
Wasabi (Wasabia/Eutrema japonica Matsum.) is a native Japanese plant used as a medicinal herb in ancient times. In Japan, wasabi is used as a spice or as pickles due to its strong pungent constituents, including isothiocyanates such as allyisothiocyanate. Previous studies have reported on antimicrobial [9], antioxidative [10] and anti-carcinogenic activities [11] of isothiocyanate. However, few studies have examined the functional properties of other components in wasabi, particularly in its summer leaves, which are considered late-growth and have little pungency [12].
In a previous study, we demonstrated the potential of hot water extract from wasabi leaves in prevention of obesity and insulin resistance through inhibition of 3T3-L1 preadipocytes differentiation [13]. In the current study, we investigated the anti-obesity effects of wasabi leaf extract (WLE), absent its specific pungent constituents, such as allyl-isothiocyanate, in mice fed high-fat diets. In order to elucidate the mechanisms of the anti-obesity effects of WLE, we conducted quantitative real-time PCR analysis, comparing gene expression profiles of the WLE and control diet groups.
Wasabi leaves were harvested in Shimane Prefecture, Japan, from 2006 to 2007, and stored at -50℃ until used for experiments. The hot water extract from wasabi leaves was prepared as previously described [13]. During those processes, the indigestible fibers known to independently decrease body weight were removed from the WLE. The obesity-induced diets were used in previous studies as a control diet, as follows: 5 g beef tallow, 3 g cholesterol/100 g and 82 g CE-2/100 g [13-16]. The CE-2 diet was supplied from CLEA Japan, Inc. (Tokyo, Japan). The WLE diet was made from a control diet supplemented with 5% (w/w) dried wasabi leaf extract (WLE). In our previous studies, the concentration of supplemented WLE was estimated according to results of comparison of WLE with (-)-epigallocatechin [13,14]. Major nutritional components of control and WLE diets are shown in Table 1.
Twenty male C57J/BL mice, aged eight weeks, weighting 20-23 g, were purchased from Japan Charles River Laboratories (Yokohama, Japan). C57J/BL mice are prone to obesity and are frequently used in studies on obesity and type 2 diabetic mellitus. All mice were allowed to acclimate for four weeks before use in the current study and were housed in the Shimane University School of Medicine Animal Laboratory, under controlled ambient conditions: relative humidity 55 ± 10%, temperature 23 ± 2℃, a 12 h light/dark cycle (07:00-19:00 and 19:00-07:00), air change 13-15 times/h, and consumption of food and water ad libitum.
At the age of 12 weeks, the mice were randomly divided into two groups: a control group and the WLE group, with 10 mice in each group. The mice were then fed their respective experimental diets for 163 days. Food intake was recorded weekly and body weight twice weekly. After 163 days of feeding, the mice were fasted overnight and anesthetized. Blood was collected from the heart; the epididymal adipose tissue, liver, kidney, and soleus muscle were harvested, rinsed, and weighed. The liver, epididymal adipose tissue, and blood samples were stored at -80℃ until analysis.
This study and all procedures were approved by the Animal Care and Use Committee of Shimane University, Japan (IZ21-45).
Blood was placed in tubes containing EDTA2Na, and plasma was separated immediately from the blood by centrifugation at 3000 × g for 10 min at 4℃. Concentrations of plasma glucose, triglycerides (TG), high-density lipoprotein-cholesterol (HDL-C), total cholesterol (TC), free fatty acid (FFA), aspartate aminotransferase (AST), alanine aminotransferase (ALT), and γ-glutamyltranspeptidase (γ-GTP) were measured using enzymatic assay kits (Glucose C test, Triglyceride G test, HDL-cholesterol test, Cholesterol E test, NEFA test, GOT·GPT C test, γ-GTP C test, Wako Pure Chemical, Osaka, Japan). Concentrations of plasma insulin, leptin, and adiponectin were measured using an Ultrasensitive Mouse Insulin ELISA Kit (Mercodia AB, Uppsala, Sweden), Mouse Leptin Assay kit (Immuno-Biological Laboratories Co., Ltd. Gunma Japan), and Mouse/rat Adiponectin ELISA Kit (Otsuka Pharmaceutical Co., Ltd. Tokyo Japan), respectively, according to the manufacturers' protocols.
For morphological analysis, each liver tissue sample was processed in a standard fashion for light microscopic examination. Briefly, liver biopsy specimens were fixed in 10% (v/v) neutral-buffered formalin for 2-3 days and embedded in paraffin. Sections of 3-5 micro-meters were stained with hematoxylin and eosin (HE). Three histological specimens were made from each liver sample, and the area percentages of fat droplets were calculated using Image J version 1.6.0 (http://rsbweb.nih.gov/ij/download.html).
To elucidate the mechanisms of the anti-obesity effects of WLE action, we measured the gene expressions of enzymes related to glucose and lipid metabolism in liver and epididymal adipose tissue using quantitative RT-PCR analysis. The total RNA was extracted from the liver and epididymal adipose tissue, using TriPure Isolation Reagent (Roche Applied Science, Mannheim, Germany) and the High Pure RNA Isolation Kit (Roche Applied Science, Mannheim, Germany) according to the supplier's protocols. Quality and quantity were checked by agarose gel electrophoresis and spectrophotometry. Reverse transcription was performed using a ReverTra Ace-α-cDNA Synthesis kit (Toyobo Co., Ltd. Osaka, Japan). Quantitative RT-PCR was performed using an Applied Biosystems 7900HT system and PRISM 7000 Sequence Detection System, using Power SYBR Green PCR Master Mix (Applied Biosystems, California, USA) according to the supplier's manual. 18S rRNA was used for internal control. Primers used in the current study were shown previous studies [14,15].
Data analyses were performed using SPSS software version 13.0J (SPSS Inc., Tokyo, Japan). Results are expressed as mean ± SD (standard deviation). Comparisons of two groups of mice were performed using Student's t-test, and P-value of less than 0.05 was used for assessment of significance.
As shown in Fig. 1, average body weights of control and WLE mice were nearly identical at the onset of the experiment; however, significant differences in body weight were observed between the two groups at 120 days. A summary of the effects of WLE on body and organ weights after 164 days of feeding is shown in Table 1. Final body weight was significantly lower in the WLE group (36.0 ± 2.8 g), compared to the control group (38.2 ± 1.8 g, P = 0.01), as was body weight gain: WLE group, 9.5 ± 1.8; control group, 11.9 ± 1.2, P < 0.01. During the 164 days, no significant differences were observed in daily and total energy intake from diets between the WLE group and the control group. Epididymal adipose tissue weight was significantly lower in the WLE group (1.16 ± 0.28 g), compared with that of the control group (1.69 ± 0.25 g, P < 0.01). The liver weight of the WLE group (1.92 ± 0.24 g) was also significantly lower than that of the control group (2.42 ± 0.45 g, P < 0.01). No significant differences were observed in the soleus muscle and kidney weights between the two groups.
A summary of the effects of WLE on plasma parameters is shown in Table 1. Only fasting plasma total cholesterol was significantly lower in the WLE group than in the control group (P < 0.05). No significant differences in plasma triglycerides, free fatty acid, HDL cholesterol, and glucose levels were observed between the two groups. For the liver function marker, significantly lower γ-GTP levels were observed in the WLE group than in the control group (P < 0.05).
As liver weight was significantly lower in the WLE group compared with the control group (Table 1), we performed morphologically evaluation of the effect of WLE on liver fat accumulation. As shown in Fig. 2 and Table 2, lipid droplets were abundant around the center vein of the liver in the control group, but to a lesser extent in the WLE group (1.00 ± 0.07 and 0.91 ± 0.08, respectively, P < 0.05).
In order to further clarify the mechanism of anti-obesity effects of WLE, we measured the effects of the two experimental diets on expression of key genes in liver and white adipose tissue. The typical genes were selected according to their metabolic function in terms of lipid metabolism in liver and white adipose tissue.
Results of gene expressions in the liver are shown in Table 3. Gene expression of PPARα, a regulatory molecule in fatty acid β-oxidation, was significantly enhanced in the WLE group (1.44 fold) compared to that of the control group. Conversely, expression of PPARγ and SREBP-1c, major regulators related to lipid synthesis and TG accumulation in hepatic cells, were significantly suppressed in the WLE group compared to the control group (0.61 and 0.32 fold, respectively). In addition, expressions of ACC, FAS, and HMGR, the central enzymes of TG and cholesterol synthesis, were significantly suppressed in the WLE group compared to the control group (0.52, 0.56, and 0.60 fold, respectively). No significant differences in expression of LXRβ, PGC-1β, SREBP-2, and ChREBP1 were observed between the two groups.
Expression of leptin, a master regulator of lipid metabolism and obesity, was significantly enhanced in the WLE group (1.21 fold), compared to the control group. Adiponectin, also a master regulator of lipid metabolism and obesity, was suppressed in the WLE group (0.51 fold). PPARγ and C/EBPα, major translational factors related to lipid synthesis and TG accumulation, were also significantly suppressed in the WLE group compared to the control group (0.75 and 0.73 fold, respectively). Acox1, which affects the level of fatty acid β-oxidation, was significantly enhanced in the WLE group compared with that of the control group (1.28 fold). No significant differences in expression of CD36, Dok1, aP2, and HSL were observed between the two groups (Table 4).
In the current study, we investigated the anti-obesity effect of hot water extract of Wasabi leaves in mice fed high-fat diets. Our results demonstrated that supplementation with WLE is effective in prevention of diet-induced obesity, in attenuation of TG accumulation in liver and adipose tissue, and in enhancement of β-oxidation in adipose tissue.
Various bioactive ingredients from plants appear to act as regulators of obesity in mice or rats fed with high-fat diets [15-18]. In previous studies, we reported on the remarkable effects of WLE in suppression of TG accumulation and inhibition of cellular differentiation in 3T3-L1 adipocytes [13]. The results of those studies led us to hypothesize that WLE may contain bioactive ingredients responsible for anti-obesity effects through stimulation of lipid metabolism and suppression of TG accumulation in liver and adipose tissue. In this study, we have demonstrated that body weight gain was significantly lower in the WLE group in comparison with the control group (P < 0.01), although average and total energy intakes did not differ significantly between the two groups (Table 1). Liver weight and epididymal white adipose tissue weight were also significantly lower in the WLE group compared with the control group. Lipid droplets in the livers of the WLE group were not as widely distributed as livers in the control group (Fig. 2). As the WLE used in this study did not include indigestible fiber and the energy intake of the two groups was essentially the same, we attempted to determine whether WLE has potential to stimulate metabolism-rerated organs, particularly liver and adipose tissue. In blood profile, significant suppression of increasing total cholesterol was observed only in the WLE group. Triglyceride and glucose levels showed a trend toward suppression in the WLE group, which was considered to result from the mild effect of WLE. In fact, in our previous studies using 3T3-L1 cells, the effect of WLE was milder than that of (-)-epigallocatechin.
At the molecular level, WLE enhanced the gene expression levels of PPARα, and suppressed levels of PPARγ and SREBP-1c, in the liver (Table 3), all regarded as important key transcriptional regulators of lipid metabolism in the liver. Increase of PPARα and decrease of SREBP-1c are thought to induce decrease of TG synthesis and accumulation in the liver, through regulation of downstream lipogenesis gene expression, such as FAS, ACC1 and SCD1, thereby preventing the onset of steatotic liver [19]. Our results showed a significantly smaller liver lipid droplet area in the WLE group, accompanied by an increase in PPARα and a decrease in expression of SREBP-1c, ACC1 and FAS. The role of PPARγ and its effect on the liver remain unclear and controversial, however, it does seem certain that overexpression of PPARγ leads to acute hepatic steatosis in ob/ob or diet/drug induced obese mice [20,21]. Expression of PPARγ in liver of the WLE group was down-regulated, possibly a factor in the presence of fewer fat droplets in their livers (Fig. 2).
Concurrent with prevention of hepatic steatosis, an increase in white adipose tissue in the WLE group was suppressed, and the expression trend of adipocytokines in white adipose tissue was the same as that seen in our previous study using preadipocytes 3T3-1L [22]. In that study, the suppressed TG accumulation and cellular differentiation were mediated, and we noted that the suppressing effects may have been caused by altered regulation of adipogenic transcriptional factors, PPARγ and C/EBPα. In the current study, expression of PPARγ and C/EBPα in adipose tissue of the WLE group was down-regulated; subsequently, Acox1, a PPAR-regulated fatty acid oxidation gene [23], was up-regulated. Thus, it is suspected that suppression of PPARγ and C/EBPα molecules affected functional factors, such as Acox1, and its involvement in energy storage and expenditure, thus resulting in suppression of fat storage in the WLE group.
We also examined the effects of WLE on plasma adiponectin and leptin concentrations as well as gene expression of adiponectin and leptin in adipose tissues. The gene expression level of adiponectin in adipose tissues was enhanced in the WLE group (1.21 fold), although plasma adiponectin levels were only slightly higher (1.12 fold, no significant difference). This discrepancy between plasma concentration and gene expression of adiponectin may be due to differences in total amounts of adipose tissue between the two groups of mice. Reduced plasma or serum adiponectin concentrations have been observed in humans with high levels of visceral fat [24-27], and intraperitoneal injection of adiponectin has been shown to improve insulin resistance by decreasing TG concentrations in muscle and liver of obese mice [28].
The plasma concentration and gene expression level of leptin in white adipose tissue remained low in the WLE group compared with the control group (0.65 fold and 0.51 fold, respectively, Table 4). Leptin is secreted from adipocytes and conveys information about energy storage from adipose tissue to the central nervous system (CNS). Uno et al.[29] demonstrated a neuronal pathway in mice, consisting of the afferent vagus from the liver and efferent sympathetic nerves to adipose tissue, which is involved in regulation of energy expenditure and fat distribution between the liver and its periphery. Therefore, in the current study, Leptin may have played an important role, and may crosstalk between adipose tissue and other fat accumulation organs, particularly the liver. In addition, elevation of fasting plasma or serum leptin concentrations in obese humans in proportion to the degree of obesity has been demonstrated [30-32]. Thus, attenuation of leptin and enhancement of adiponectin by WLE suggest that WLE may have a potentially useful role in prevention of obesity-induced metabolic disorders.
There are some limitations in the current studies. We have not been able to determine the functional components of WLE prior to its use in this experiment. We previously reported that TG accumulation and differentiation of 3T3-L1 preadipocytes were suppressed by WLE, and that the benefits of WLE were independent of dietary fiber and allyl-isothiocyanate. To the best of our knowledge, there are a significant number of bioactive components related to obesity and obesity related disease in natural foods, such as polyphenole, which affect gene expression in liver and adipose tissue. We therefore believe that it is the bioactive compounds in wasabi leaves that provide the main contribution to the anti-obesity effects. The current studies were a preliminary animal experiment on feeding with a WLE-supplemented diet. Conduct of future studies will be required in order to determine with greater specificity the anti-obesity compounds in wasabi leaves and to investigate any possible toxicities.
Findings of the current studies demonstrates that WLE from wasabi leaves can induce mild prevention of obesity in high-fat-fed mice, and these effects may be related to the decrease in TG synthesis and accumulation in the liver, and the decrease of TG accumulation and enhancement of fatty acid oxidization in adipose tissue. The current studies demonstrates the potential for use of extract from Wasabi leaves as a health supplement to help reduce obesity.
Figures and Tables
Fig 1
Effects of WLE on body weight changes in mice. Each point represents mean ± SD (n = 10). Asterisks indicate significant difference between the WLE and control groups (P < 0.05).
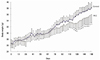
Fig 2
Histological staining of liver sections and fat droplet areas of the control and WLE groups after feeding for 164 days

References
2. Goran MI, Ball GD, Cruz ML. Obesity and risk of type 2 diabetes and cardiovascular disease in children and adolescents. J Clin Endocrinol Metab. 2003; 88:1417–1427.


3. Furuyashiki T, Nagayasu H, Aoki Y, Bessho H, Hashimoto T, Kanazawa K, Ashida H. Tea catechin suppresses adipocyte differentiation accompanied by down-regulation of PPARgamma2 and C/EBPalpha in 3T3-L1 cells. Biosci Biotechnol Biochem. 2004; 68:2353–2359.


4. Sayama K, Lin S, Zheng G, Oguni I. Effects of green tea on growth, food utilization and lipid metabolism in mice. In Vivo. 2000; 14:481–484.
5. Zhang YT, Wang Y, Zhang XT, Wu DL, Zhang XQ, Ye WC. A new decalin derivative from red yeast rice. J Asian Nat Prod Res. 2009; 11:792–795.


6. Iwashita K, Kobori M, Yamaki K, Tsushida T. Flavonoids inhibit cell growth and induce apoptosis in B16 melanoma 4A5 cells. Biosci Biotechnol Biochem. 2000; 64:1813–1820.


7. Harmon AW, Harp JB. Differential effects of flavonoids on 3T3-L1 adipogenesis and lipolysis. Am J Physiol Cell Physiol. 2001; 280:C807–C813.


8. Nakai R, Masui H, Horio H, Ohtsuru M. Effect of maitake (Grifola frondosa) water extract on inhibition of adipocyte conversion of C3H10T1/2B2C1 cells. J Nutr Sci Vitaminol (Tokyo). 1999; 45:385–389.


9. Isshiki K, Tokuoka K, Mori R, Chiba S. Preliminary examination of allyl isothiocyanate vapor for food preservation. Biosci Biotechnol Biochem. 1992; 56:1476–1477.


10. Fukuchi Y, Kato Y, Okunishi I, Matsutani Y, Osawa T, Naito M. 6-Methylsulfinylhexyl isothiocyanate, an antioxidant derived from Wasabia japonica MATUM, ameliorates diabetic nephropathy in type 2 diabetic mice. Food Sci Technol Res. 2004; 10:290–295.


11. Fuke Y, Haga Y, Ono H, Nomura T, Ryoyama K. Anti-carcinogenic activity of 6-methylsulfinylhexyl isothiocyanate-, an active anti-proliferative principal of wasabi (Eutrema wasabi Maxim.). Cytotechnology. 1997; 25:197–203.
12. Mochida K, Ogawa T. Anti-influenza virus activity of extract of Japanese wasabi leaves discarded in summer. J Sci Food Agric. 2008; 88:1704–1708.


13. Ogawa T, Tabata H, Katsube T, Ohta Y, Yamasaki Y, Yamasaki M, Shiwaku K. Suppressive effect of hot water extract of wasabi (Wasabia japonica Matsum.) leaves on the differentiation of 3T3-L1 preadipocytes. Food Chem. 2010; 118:239–244.


14. Wang L, Yamasaki M, Katsube T, Sun X, Yamasaki Y, Shiwaku K. Antiobesity effect of polyphenolic compounds from molokheiya (Corchorus olitorius L.) leaves in LDL receptor-deficient mice. Eur J Nutr. 2011; 50:127–133.


16. Knekt P, Kumpulainen J, Järvinen R, Rissanen H, Heliövaara M, Reunanen A, Hakulinen T, Aromaa A. Flavonoid intake and risk of chronic diseases. Am J Clin Nutr. 2002; 76:560–568.


17. Williamson G, Carughi A. Polyphenol content and health benefits of raisins. Nutr Res. 2010; 30:511–519.


18. Quideau S, Deffieux D, Douat-Casassus C, Pouységu L. Plant polyphenols: chemical properties, biological activities, and synthesis. Angew Chem Int Ed Engl. 2011; 50:586–621.


19. Morise A, Thomas C, Landrier JF, Besnard P, Hermier D. Hepatic lipid metabolism response to dietary fatty acids is differently modulated by PPARalpha in male and female mice. Eur J Nutr. 2009; 48:465–473.


20. Schadinger SE, Bucher NL, Schreiber BM, Farmer SR. PPAR gamma2 regulates lipogenesis and lipid accumulation in steatotic hepatocytes. Am J Physiol Endocrinol Metab. 2005; 288:E1195–E1205.
21. Motomura W, Inoue M, Ohtake T, Takahashi N, Nagamine M, Tanno S, Kohgo Y, Okumura T. Up-regulation of ADRP in fatty liver in human and liver steatosis in mice fed with high fat diet. Biochem Biophys Res Commun. 2006; 340:1111–1118.


22. Hotamisligil GS, Arner P, Caro JF, Atkinson RL, Spiegelman BM. Increased adipose tissue expression of tumor necrosis factor-alpha in human obesity and insulin resistance. J Clin Invest. 1995; 95:2409–2415.


23. Shimoda H, Tanaka J, Kikuchi M, Fukuda T, Ito H, Hatano T, Yoshida T. Effect of polyphenol-rich extract from walnut on diet-induced hypertriglyceridemia in mice via enhancement of fatty acid oxidation in the liver. J Agric Food Chem. 2009; 57:1786–1792.


24. Ouchi N, Kihara S, Arita Y, Okamoto Y, Maeda K, Kuriyama H, Hotta K, Nishida M, Takahashi M, Muraguchi M, Ohmoto Y, Nakamura T, Yamashita S, Funahashi T, Matsuzawa Y. Adiponectin, an adipocyte-derived plasma protein, inhibits endothelial NF-kappaB signaling through a cAMP-dependent pathway. Circulation. 2000; 102:1296–1301.


25. Arita Y, Kihara S, Ouchi N, Takahashi M, Maeda K, Miyagawa J, Hotta K, Shimomura I, Nakamura T, Miyaoka K, Kuriyama H, Nishida M, Yamashita S, Okubo K, Matsubara K, Muraguchi M, Ohmoto Y, Funahashi T, Matsuzawa Y. Paradoxical decrease of an adipose-specific protein, adiponectin, in obesity. Biochem Biophys Res Commun. 1999; 257:79–83.


26. Okamoto Y, Arita Y, Nishida M, Muraguchi M, Ouchi N, Takahashi M, Igura T, Inui Y, Kihara S, Nakamura T, Yamashita S, Miyagawa J, Funahashi T, Matsuzawa Y. An adipocyte-derived plasma protein, adiponectin, adheres to injured vascular walls. Horm Metab Res. 2000; 32:47–50.


27. Yamauchi T, Kamon J, Minokoshi Y, Ito Y, Waki H, Uchida S, Yamashita S, Noda M, Kita S, Ueki K, Eto K, Akanuma Y, Froguel P, Foufelle F, Ferre P, Carling D, Kimura S, Nagai R, Kahn BB, Kadowaki T. Adiponectin stimulates glucose utilization and fatty-acid oxidation by activating AMP-activated protein kinase. Nat Med. 2002; 8:1288–1295.


28. Yamauchi T, Kamon J, Waki H, Terauchi Y, Kubota N, Hara K, Mori Y, Ide T, Murakami K, Tsuboyama-Kasaoka N, Ezaki O, Akanuma Y, Gavrilova O, Vinson C, Reitman ML, Kagechika H, Shudo K, Yoda M, Nakano Y, Tobe K, Nagai R, Kimura S, Tomita M, Froguel P, Kadowaki T. The fat-derived hormone adiponectin reverses insulin resistance associated with both lipoatrophy and obesity. Nat Med. 2001; 7:941–946.


29. Uno K, Katagiri H, Yamada T, Ishigaki Y, Ogihara T, Imai J, Hasegawa Y, Gao J, Kaneko K, Iwasaki H, Ishihara H, Sasano H, Inukai K, Mizuguchi H, Asano T, Shiota M, Nakazato M, Oka Y. Neuronal pathway from the liver modulates energy expenditure and systemic insulin sensitivity. Science. 2006; 312:1656–1659.


30. Maffei M, Halaas J, Ravussin E, Pratley RE, Lee GH, Zhang Y, Fei H, Kim S, Lallone R, Ranganathan S, Kern PA, Friedman JM. Leptin levels in human and rodent: measurement of plasma leptin and ob RNA in obese and weight-reduced subjects. Nat Med. 1995; 1:1155–1161.

