Abstract
The study elucidated carbohydrase inhibition, anti-cancerous, free radical scavenging properties and also investigated the DNA and protein protection abilities of methanolic root extract of Rumex crispus (RERC). For this purpose, pulverized roots of Rumex crispus was extracted in methanol (80% and absolute conc.) for 3 hrs for 60℃ and filtered and evaporated with vacuum rotary evaporator. RERC showed high phenolic content (211 µg/GAE equivalent) and strong 2,2-diphenyl-1-picrylhydrazyl (DPPH) radical scavenging (IC50 = 42.86 (absolute methanol) and 36.91 µg/mL (80% methanolic extract)) and reduced power ability. Furthermore, RERC exhibited significant protective ability in H2O2/Fe3+/ascorbic acid-induced protein or DNA damage and percentage inhibition of the HT-29 cell growth rate following 80% methanolic RERC exposure at 400 µg/mL was observed to be highest (10.2% ± 1.03). Moreover, methanolic RERC inhibited α-glucosidase and amylase effectively and significantly (P < 0.05). Conclusively, RERC could be considered as potent carbohydrase inhibitor, anti-cancerous and anti-oxidant.
Oxidative stress has been implicated as one of prime concern in the pathogenesis of diabetes and cancer by damaging cells and their proteomes. Oxidative stress normally occurs due to the initiation of free radicals, which seeks stability through electron pairing with the proteins, lipids and DNA of cells leading to their damage [1]. Though, all living organisms, including animals and human beings, have innate antioxidant defense but this natural protective mechanism normally fail to perform well during the pathology. Therefore, dietary intake of antioxidant compound as a natural medicine becomes pertinent [2].
Rumex crispus (yellow or curled dock, sheep or french sorrel etc) is an wild herbaceous perennial weed plant, belongs to polygonacae (buckwheat family) and more than 200 species of which have been identified, distributed widely in the humid regions of northern hemisphere and vegetate mostly in the acid (silicate) [3]. Traditionally, its roots have been largely recommended by herbalists for range of skin diseases, icterus and GI tracts ailments in general [4]. However, reports about its fruits being used in dysentery and leaves as vegetables have been documented by Tyler [5]. Rumex isn't readily consumed by domestic livestock [6], but when consumed accidently might cause dermatitis and gastric distress in ruminants upon its large consumption [7].
Regardless of its widespread use, there is a scarcity of chemical evidence to support any claim of therapeutic values for yellow dock, except for astringent and laxative properties [5]. Therefore, the root extracts of Rumex crispus (RERC) in absolute and 80% methanol was collected to study in vitro antioxidant activities and to add knowledge to the available literature about its direct relationship with other alternative properties like carbohydrase inhibition, anti-cancerous along with DNA and protein protective abilities.
All chemicals and reagents used in this study were purchased from Sigma (St. Louis, MO, USA) until or unless specified. 2-Thiobarbituric acid (TBA) was purchased from Alfa Aesar (Karlsruhe, Germany). All the chemicals and reagents were of analytical grade.
The roots of Rumex crispus was purchased from Gangwon of Republic of Korea and roots were identified by herbalist (College of Biomedical and indentified and authenticated by Professor M. H. Wang (College of Biomedical Science, Kangwon National University). The roots of Rumex crispus (RERC) was chopped to small size of 0.5 cm long, dried in shade and powdered in mechanical grinder. The extraction of Rumex crispus were carried out using known standared procedure followed by Hu et al. [8]. In brief, two hundred grams of the pulverized roots were extracted with absolute and 80% methanol at 60℃ for 3 hours and finally the extraction was dried under vacuum rotary evaporator. The positive controls and RERC were dissolved in the respective solvent to the concentration of 10 mg/mL as stocks.
Total phenolic content in RERC was determined as described by Hu et al. [8]. Tannic acid (Tan) was used as the standard to create a calibration curve. The total phenolic content was expressed as mg Tan/g RERC.
The reducing power assay was determined according to the method of Hu et al. [9]. The absorbance of the finally incubated mixture of each sample was measured at 700 nm after mixing it with ferric chloride. α-Tocopherol and BHT were used as positive controls.
The free radical scavenging activity of RERC was determined by the DPPH test [10]. Tocopherol α and BHT were used as positive controls.
The capability to scavenge the DPPH radical was calculated using the following equation: I (%) = [1 - (Ai - Aj)/Ac] × 100. Ac is the absorbance of the DPPH solution without sample (0.5 mL DPPH solution + 0.5 mL of absolute or 80% methanol); Ai is the absorbance of the test sample mixed with DPPH solution (0.5 mL sample + 0.5 mL DPPH solution) and Aj is the absorbance of the sample without DPPH solution (0.5 mL sample + 0.5 mL of absolute or 80% methanol).
The chelation of ferrous ions by RERC was estimated as described the method by Que et al. [11]. The absorbance of each sample was measured at 562 nm. EDTA at the concentration of 0.1 mg/mL was used as a positive control.
The superoxide radical scavenging activity was determined by the PMS-NADH generating system described previously [12] with minor modifications. The absorbance of all the samples was measured at 560 nm. Galic acid at the concentration of 0.125 mg/mL was used as a positive control.
α-Glucosidase inhibitory activities were determined as the methodology described by McDougall et al. [13]. Release of p-nitrophenol was measured at 405 nm spectrophotometrically (SpectraMAX Plus 384, Molecular Devices Corporation, Sunnyvale, CA, USA) 5 min after incubation with substrate. The assay was adopted from Ali et al. [14] and modified accordingly to suite microplate reading.
α-Amylase activity was determined as per the protocol of Ali et al. [14] with minor modification to suite microplate reading. Variation in the amylase activity was measured at 540 nm spectrophotometrically as above.
The percentage of enzyme inhibition in both the assays were calculated as (1-B/A) × 100 where A represents the absorbance of the control without test samples, and B represents the absorbance in the presence of test samples. All the tests were run in triplicates. The IC50 values were calculated by applying logarithmic regression analysis from the mean inhibitory values.
The effects of RERC on protein oxidation were carried out according the method described by Kızıl et al. [15]. Interactions of the samples were done with BSA protein. Samples incubated with BSA protein was further analyzed by PAGE and the band intensity was estimated after following the staining and distaining protocols with image analysis software (Quantity One, Bio-Rad, Hercules, CA).
The effects of RERC on DNA (HEK 293) damage protection were carried out according the method described earlier by Qian et al. [16]. Briefly, different concentrations of 80% and absolute methanolic RERC (100, 250, 500 and 1,000 ug/mL) and 0.5 µg of DNA extracted from HEK 293 cells were mixed and incubated for 10 min at room temperature followed by the addition of 7 µL of Fenton's reagent (3 µL of 30% H2O2, 2 µL of 5 mM FeSO4, and 2 µL of 50 mM PB). The resulting mixture (final volume 20 µL) was incubated for 30 min at 37℃. The DNA was electrophoresed on 1% agarose gels and visualized using ethidium bromide and a Mini BIS image analysis system (DNR Bio-Imaging Systems Ltd., Jerusalem, Israel). Densitometric analysis was done using image analysis software (Quantity One, Bio-Rad, Hercules, CA). The extent of DNA damage protection (%) was calculated by comparing the proportion of DNA with Fenton's reagent to that without Fenton's reagent.
Cell apoptosis as an effective potential of the root extracts of Rumex crispus were evaluated on HT-29 human cancer cell line with a conventional MTT assay. At 48 h prior to culture termination, 20 µL of the MTT solution (4 mg/mL in a phosphate-buffered saline (PBS), pH 7.4) was added to each culture plates and the cells were remained in culture for additional for 4 h. Later, 200 µL of dimethyl sulfoxide (DMSO) was added for solubilization. Absorbance was measured at 550 nm using an enzyme-linked immunosorbent assay (ELISA) plate reader (Bio-Tek, Winooski, VT, USA).
All tests were carried out independently in triplicate (n=3). The data was expressed as the mean ± standard derivation (SD). All analyses were performed using SPSS 7.5 (SPSS Institute, Cary, NC, USA); individual comparisons were made using Tukey's multiple-range test, which was used to determine the differences between the means.
Yeild % of 80% and absolute methanolic extracts were found to be 29.1 and 29.9 respectively. Amounts of phenolic compound were 175.3 mg/g and 211.2 mg/g in absolute methanolic and 80% methanolic root extracts of Rumex crispus. 80% methanolic extracts showed significantly (P < 0.05) higher reducing power activity as compared to absolute methanolic extract. However, effect on reducing power activity was increased with increasing concentrations (Fig. 1).
Metal chelation activity of 80% methanolic RERC was found to significant (P < 0.05/0.01) at the concentration ranging 500-2,000 µg/mL as compared to absolute methanolic RERC. However, the values remained lower than EDTA. Similarly, superoxide radical scavenging activity of 80% methanolic RERC was also found to be highly significant (P < 0.01) and invariably in parallel to the positive control (Gallic acid) as compared to absolute methanolic RERC (Fig. 2).
DPPH radical scavenging activity of absolute and 80% methanolic RERC was highly significant (P < 0.01) as compared to Vit E and BHT (Table 1). However, 80% methanolic extract was observed to be better DPPH radical scavenger compared to absolute methanolic extract. On the other hand, RERC inhibited cell growth in a dose dependent manner as estimated by MTT assay (Fig. 3). % inhibition of HT-29 cell growth rate following absolute and 80% methanolic RERC exposure at 400 µg/mL was 40.1% ± 0.87 and 10.2% ± 1.03 respectively.
Both absolute and 80% methanol RERC showed significant (P < 0.01) inhibition of α-glucosidase and α-amylase as compared to acarbose (positive control) (Fig. 4).
Absolute and 80% methanolic RERC protected the cellular proteome (BSA) significantly (P < 0.05) at the concentration higher than 500 µg/mL. However, the lower dosage was also seemed to be effective in protein protection (Fig. 5).
Absolute and 80% methanolic RERC protected the HEK 293 cellular DNA significantly (P < 0.05) at the concentration 100 µg/ml and more. However, the lower dosage was seemed to be effective in cellular DNA protection (Fig. 6).
Presence of increased phenolic moieties in the absolute and 80% methanolic RERC shows their increased antioxidant property (Fig. 1). It has been documented that the potential to function as antioxidants by scavenging a superoxide anion, singlet oxygen, lipid peroxy radicals and stabilizing free radicals involved in oxidative processes through hydrogenation with oxidizing species due to the presence of the conjugated ring structures and hydroxyl groups [17].
Polyphenolic nature of extracts of Rumex crispus seems to be responsible for the scavenging activity of the reactive oxygen species (ROS) which further makes it suitable candidate in protecting cellular proteins (Fig. 3). ROS are involved in the normal physiology of living organisms. They act as a messenger for signal transduction and also affect gene expression and are also actively involved in the pathogenesis of diseases such diabetes, cancer and neurodegenerative diseases [18]. Significant protective effect of cell proteins (BSA) by RERC as shown in our studies further confirmed that methanolic RERC's are highly anti-oxidatant which protects proteins from ROS attack. ROS normally attacks the polypeptide backbone of proteins and lead to the formation of alkyl, alkoxyl, and alkylperoxyl radical intermediates, which set the stage for cleavage of the peptide bond via several means [18].
Oxidative DNA damage has been implicated in various degenerative diseases [19]. DNA damage by ROS can initiate carcinogenesis and lead to the pathogenesis of neurodegenerative diseases such as Parkinson's and Alzheimer's disease. Hydroxy radicals are regarded as a DNA damaging agent which can significantly damage the DNA contents of the cells [20]. Though, both 80% methanolic and absolute methanolic RERC was found to be highly potential in DNA protection (HEK 293 cellular DNA) when compared with the positive control (Fig. 6). However, 80% methanolic RERC was found to be superior in protecting DNA damage over the other groups.
Alpha-glucosidase and amylase have been observed to be effectively inhibited by RERC in the present study (Fig. 4). Alpha-glucosidase and amylase are important enzymes responsible for the digestion of dietary carbohydrates in the gut. It has been reported previously that inhibitors of these enzymes could be used therapeutically in postprandial hyperglycemia [21]. Rumex crispus roots have been in the past demonstrated as broad-spectrum agent in diabetic disorders [21]. On the contrary, the reports demonstrated that α-amylase gets activated by plant extracts and inturn may aggravate glucose production in vivo [14] as it has been claimed that α-amylase breaks starch into disaccharides that are acted upon by isomaltases, especially α-glucosidase to release glucose. Therefore, presence of potent α-glucosidase inhibitory activity in our study claims strongly that Rumex crispus roots has important in controlling the release of glucose [14].
Free radicals have usually been known as the major cause of oxidative damage of biological molecules, including coronary heart disease, aging, cancer and dementia. DPPH has been used to evaluate the free-radical scavenging capacity of antioxidants (Table. 1) [22]. The reducing power of a compound has also been associated with antioxidant activity (Fig. 1). The researchers have observed and demonstrated a direct correlation between antioxidant activities and reducing power of certain plant extracts [22]. Additonally, it has been reported that transition metals are involved in both initiation and propagation of oxygen free radicals in the organisms. Transition elements, such as iron and copper, are powerful catalysts of oxidation reactions because they contain one or more unpaired electrons that can participate in electron transfer reactions [23] (Fig. 2). Scavenging of superoxide radicals (considered as harmful to cellular components as a precursor of ROS) is one of the most important ways of clarifying the mechanism of antioxidant activity [24] (Fig. 2). These results indicated that RERC had a notable effect on scavenging of superoxide radicals.
The RERC induced HT-29 cell apoptosis in a dose-dependent manner (Fig. 5), but we did not know which apoptotic signal pathway was involved in this phenomenon. However, we believe that RERC certainly down-regulated the expression of certain transcriptional factors (p53, caspase 3, c-Myc, Bax) which have been demonstrated previously as a multifunctional transcription factor in the regulation of critical cellular processes such as proliferation, cell cycle arrest, DNA repair, and apoptosis [25]. Methanolic extracts of RERC might have also downregulated Active caspase 3, c-Myc, cyclin D, cyclin E and Bax as observed during the experiments on HT-29 cells [26].
Methanolic RERC are anti-diabetic, anticancerous, free radical scavengers and also have ability to protect DNA and protein contents of the proliferating cells. However, 80% methanolic RERC was observed to have better potential as therapeutic candidate than absolute methanolic RERC. Therefore, detailed chemical analyses to know the exact compounds of RERC which are responsible for these biological activities are underway by using HPLC or other advanced technologies.
Figures and Tables
Fig. 1
Reducing power potential of absolute and 80% methanolic extracts of Rumex crispus roots. α-Tocopherol and BHT were used as positive controls. Each value was expressed as mean ± SD (n = 3). *(P < 0.05) representing significant values.
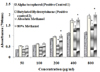
Fig. 2
Metal chelating activity (A) and superoxide radical scavenging activity (B) of absolute and 80% methanolic extracts of Rumex crispus roots. EDTA and galic acid was used as positive control. Each value was expressed as mean ± SD (n = 3). *P < 0.05 and **P < 0.01 representing significant values.
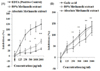
Fig. 3
Cell apoptosis representation of human cancer cells (HT-29) under the influence of absolute and 80% methanolic extracts of Rumex crispus roots. (A): Phase contrast microscopic examination of cell apoptosis of human cancer cells (HT-29); 1) Control; 2) 80% methanolic extract; 3) Absolute methanolic extract. (B): Cell apoptosis % of human cancer cells (HT-29) at different concentration of root extracts of Rumex crispus in culture for 48 h. Cell viability was measured using an MTT assay. Data represent the mean ± SD of three independent experiments.
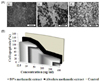
Fig. 4
Influence of absolute and 80% methanolic extracts of Rumex crispus roots. A: α-glucosidase (at 200 µg/mL). B: α-amylase (at 400 µg/mL concentration). Each value was expressed as mean ± SD (n = 3). *P < 0.05 and **P < 0.01 representing significant values.
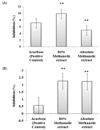
Fig. 5
Protection of BSA oxidative damage by absolute and 80% methanolic extracts of Rumex crispus. The electrophoretic pattern of BSA and densitometric analysis of the corresponding band intensity has been depicted in all the graphs representing different concentration of the extracted samples i.e. A (100 µg/mL), B (500 µg/mL), C (1,000 µg/mL) and D (5,000 µg/mL). BSA was oxidised by Fenton system (Fe3+/H2O2/ascorbic acid). Each bar represents the mean ± SD of three different experiments. Means with different letters at a time differ significantly, P < 0.05. The values sharing common letters are not significantly different at P < 0.05. *P < 0.05 compared with Fe3+/H2O2/ascorbic acid treated group.
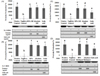
Fig. 6
HEK 293 cellular DNA protection from hydroxyl radical with methanolic (80% and absolute) extract from Rumex crispus has been shown on agarose gel electrophoresis and quantification of DNA band has been indicated on bar graphs. Histogram showing the protective effect of different concentration of extract i.e. A (100 ug/mL), B (250 ug/mL), C (500 ug/mL) and D (1,000 ug/mL) against DNA damage based on densitometric measurements. Line 1, DNA incubated without Fenton's reagent; Line 2-3, DNA incubated with Fenton's reagent in the presence of 100, 250, 500 and 1,000 µg/mL, respectively; line 4: DNA incubated with Fenton's reagent.
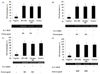
References
1. Hazra B, Sarkar R, Mandal S, Biswas S, Mandal N. Studies on antioxidant and antiradical activities of Dolichos biflorus seed extract. Afr J Biotechnol. 2009. 8:3927–3933.
2. Espín JC, Soler-Rivas C, Wichers HJ. Characterization of the total free radical scavenger capacity of vegetable oils and oil fractions using 2,2-diphenyl-1-picrylhydrazyl radical. J Agric Food Chem. 2000. 48:648–656.


3. Slavnić Ž. Josifović M, editor. Polygonales-Polygonaceae. Flora of SR Serbia. 1972. Vol. III. Belgrade: Serbian Academy of Sciences and Arts;68–86.
4. Yıldırım A, Mavi A, Kara AA. Determination of antioxidant and antimicrobial activities of Rumex crispus L. extracts. J Agric Food Chem. 2001. 49:4083–4089.


5. Tyler VE. The Honest Herbal: A Sensible Guide to the Use of Herbs and Related Remedies. 1993. 3rd ed. New York: Pharmaceutical Products Press, An imprint of the Haworth Press, Inc.;325–326.
6. Burrill LC. Curly Dock and Broadleaf Dock, Rumex Crispus L. and Rumex Obtusifolius L. 1992. Oregon State University Extension Service, Washington State University Extension Service, University of Idaho Extension Service;Available from:
http://ir.library.oregonstate.edu/xmlui/bitstream/handle/1957/16791/pnw398.pdf.
7. Rutledge CR, McLendon T. An Assessment of Exotic Plant Species of Rocky Mountain National Park: Summary Information for Remaining Exotic Plant Species. 2002. Colorado: Department of Rangeland Ecosystem Science, Colorado State University;97. United States Geological Survey, Northern Prairie Wildlife Research Center Home Page. Available from:
http://www.npwrc.usgs.gov/resource/plants/explant/index.htm.
8. Hu W, Shen W, Wang MH. Free radical scavenging activity and protective ability of methanolic extract from Duchesnea indica against protein oxidation and DNA damage. J Food Sci Nutr. 2009. 14:277–282.


9. Hu W, Heo SI, Wang MH. Antioxidant and anti-inflammatory activity of Kalopanax pictus leaf. J Korean Soc Appl Biol Chem. 2009. 52:360–366.
10. Ghasemi K, Ghasemi Y, Ebrahimzadeh MA. Antioxidant activity, phenol and flavonoid contents of 13 citrus species peels and tissues. Pak J Pharm Sci. 2009. 22:277–281.
11. Que F, Mao L, Zhu C, Xie G. Antioxidant properties of Chinese yellow wine, its concentrate and volatiles. Lebenson Wiss Technol. 2006. 39:111–117.


12. Singh N, Rajini PS. Free radical scavenging activity of an aqueous extract of potato peel. Food Chem. 2004. 85:611–616.


13. McDougall GJ, Shpiro F, Dobson P, Smith P, Blake A, Stewart D. Different polyphenolic components of soft fruits inhibit α-amylase and α-glucosidase. J Agric Food Chem. 2005. 53:2760–2766.


14. Ali H, Houghton PJ, Soumyanath A. α-Amylase inhibitory activity of some Malaysian plants used to treat diabetes; with particular reference to Phyllanthus amarus. J Ethnopharmacol. 2006. 107:449–455.


15. Kızıl G, Kızıl M, Ceken B. Protective ability of ethanol extracts of Hypericum scabroides Robson & Poulter and Hypericum triquetrifolium Turra against protein oxidation and DNA damage. Food Sci Biotechnol. 2009. 18:130–136.
16. Qian ZJ, Jung WK, Byun HG, Kim SK. Protective effect of an antioxidative peptide purified from gastrointestinal digests of oyster, Crassostrea gigas against free radical induced DNA damage. Bioresour Technol. 2008. 99:3365–3371.


17. Frankel EN. Natural and biological antioxidants in food and biological systems, their mechanism of action, applications and implications. Lipid Technol. 1995. 7:77–80.
18. Kızıl G, Kızıl M, Ceken B, Yavuz M, Demir H. Protective ability of ethanol extracts of Hypericum scabrum L. and Hypericum retusum aucher against the protein oxidation and DNA damage. Int J Food Prop. 2011. 14:926–940.


19. Loft S, Fischer-Nielsen A, Jeding IB, Vistisen K, Poulsen HE. 8-Hydroxydeoxyguanosine as a urinary biomarker of oxidative DNA damage. J Toxicol Environ Health. 1993. 40:391–404.


20. Je JY, Ahn CB, Oh MJ, Kang SY. Antioxidant activity of a red seaweed Polysiphonia morrowii extract. Food Sci Biotechnol. 2009. 18:124–129.
21. Yamagishi S, Nakamura K, Takeuchi M. Inhibition of postprandial hyperglycemia by acarbose is a promising therapeutic strategy for the treatment of patients with the metabolic syndrome. Med Hypotheses. 2005. 65:152–154.


22. Ferrari CK, Torres EA. Biochemical pharmacology of functional foods and prevention of chronic diseases of aging. Biomed Pharmacother. 2003. 57:251–260.


23. Wang J, Yuan X, Jin Z, Tian Y, Song H. Free radical and reactive oxygen species scavenging activities of peanut skins extract. Food Chem. 2007. 104:242–250.


24. Je JY, Kim SK. Reactive oxygen species scavenging activity of aminoderivatized chitosan with different degree of deacetylation. Bioorg Med Chem. 2006. 14:5989–5994.

