Abstract
Inhibition of angiotensin I-converting enzyme (ACE) activity is the most common mechanism underlying the lowering of blood pressure. In the present study, five organic extracts of a marine brown seaweed Ecklonia cava were prepared by using ethanol, ethyl acetate, chloroform, hexane, and diethyl ether as solvents, which were then tested for their potential ACE inhibitory activities. Ethanol extract showed the strongest ACE inhibitory activity with an IC50 value of 0.96 mg/ml. Five kinds of phlorotannins, phloroglucinol, triphlorethol-A, eckol, dieckol, and eckstolonol, were isolated from ethanol extract of E. cava, which exhibited potential ACE inhibition. Dieckol was the most potent ACE inhibitor and was found to be a non-competitive inhibitor against ACE according to Lineweaver-Burk plots. Dieckol had an inducible effect on the production of NO in EAhy926 cells without having cytotoxic effect. The results of this study indicate that E. cava could be a potential source of phlorotannins with ACE inhibitory activity for utilization in production of functional foods.
Hypertension is one of the major risk factors for the development of cardiovascular diseases, including stroke, and may also play a role in the development of vascular cognitive impairment and vascular dementia [1,2]. Angiotensin I-converting enzyme (EC 3.4.15.1; ACE), which plays an important role in the rennin-angiotensin system, is a carboxyl-terminal dipeptidyl exopeptidase that catalyzes the conversion of angiotensin I to angiotensin II [3-6]. Specifically, ACE converts an inactive form of angiotensin I, a decapeptide, to the potent vasoconstrictor angiotensin II, an octapeptide. In addition, since ACE is a multifunctional enzyme, it also catalyzes the degradation of bradykinin, which is a known vasodilator [4,7]. Therefore, inhibition of ACE activity leads to a decrease in the concentration of angiotensin II and increases the level of bradykinin, resulting in reduced blood pressure [8].
The discovery of captopril as a potent inhibitor of ACE has led to the recent development of many series of novel structures with similar biological activity [9]. To date, a wide variety of ACE inhibitors from various land and marine food sources have been reported, including from milk [10], cheese [11], egg white [12], canola [13], peanut [14], rapeseed [15], antler [16], fish muscle [17], seaweeds [18], and tuna [2].
Many academic, scientific, and regulatory organizations are considering ways to establish a scientific basis to support and further validate claims of functional components or foods containing them. Consumer interest in the relationship between diet and health has increased the demand for information about functional foods. More recently, the food industry, agricultural community, and now consumers have all shown growing interest in the field of functional foods [19,20].
Marine organisms are a rich source of structurally novel and biologically active metabolites [21]. Secondary or primary metabolites produced by these organisms may potentially be bioactive compounds of interest in the food and neutraceutical industries. The worldwide demand is growing for seaweeds as useful resource for food ingredients and processed foods [22,23]. Therefore, algae are a very interesting natural source of new compounds with biological activities that could be used as functional ingredients. E. cava, a kind of brown alga (Laminariaceae) that is found abundantly in the subtidal regions of Jeju Island, Korea, and Japan [24]. It is plentifully produced in Jeju Island, Korea for commercial purposes. This brown seaweed is popular in Korea and Japan as a food ingredient and supplement for animal feed and fertilizers [25]. In addition, an increasing number of scientific papers published over last few years have identified various biological activities and possible industrial applications of the brown seaweed E. cava.
Polyphenols have emerged as one major category of natural products important to human health. Increasing scientific evidence shows that polyphenols are good antioxidants, are effective in preventing cardiovascular and inflammatory diseases, and can also be used as chemo-preventative agents for cancer [26]. Phlorotannins (brown-algal polyphenols), a subgroup of tannins, are the least studied group of tannins and are found only in brown algae. They are produced entirely by polymerization of phloroglucinol [27,28]. Moreover, brown seaweeds have been recognized as potential sources of these compounds [29]. It is expected that the high content of phlorotannins present in Ecklonia species is responsible for various bioactivities [24,25]. Little attention has been given to either the qualitative or quantitative effects of phlorotannins on hypertension. ACE inhibitory activity of phlorotannins from E. cava and their kinetics have not been reported so far. Therefore, the purpose of the present study was to examine the ACE inhibitory activity of phlorotannins from the brown seaweed E. cava.
The brown seaweed E. cava was collected along the cost of Jeju Island, South Korea. Salt, epiphytes, and sand were removed from the samples using tap water. Then, the E. cava samples were carefully rinsed in fresh water and stored at -20℃. The frozen samples were lyophilized and homogenized with a grinder prior to extraction. ACE, Hippuryl-L-hystydyl-L-leusine (HHL), 3-(4,5-dimethyl-2-thiazolyl)-2,5-diphenyl tetrazolium bromide (MTT), and Dulbecco's modified eagle medium (DMEM) were obtained from Sigma Chemicals Co. (St. Louis, MO, USA). All the other chemicals used in this study were of analytical grade.
Marine brown alga, E. cava, was extracted separately using five organic solvents. Organic solvents used were ethanol, hexane, ethyl acetate, chloroform, and diethyl ether. One gram of E. cava powder was mixed with 100 ml of each organic solvent separately and kept in a shaking incubator (120 rpm) for 24 h at room temperature. Then, the extracts were filtered and evaporated under vacuum at 40℃ in order to obtain dry extracts.
Total phenolic content (TPC) was determined according to the protocol described by Chandler and Dodds [30]. One milliliter of sample was mixed in a test tube containing 1 ml of 95% ethanol, 5 ml of distilled water, and 0.5 ml of 50% Folin-Ciocalteu reagent. The mixture was allowed to react for 5 min, after which 1 ml of 5% Na2CO3 was mixed thoroughly and placed in the dark for 1 h. Absorbance was measured at 725 nm using a UV-VIS spectrometer (Opron 3000 Hansan Tech. Co Ltd., Korea). A gallic acid standard curve was obtained for calibration of TPC.
Isolation of phlorotannins was carried out according to the method described by Heo et al. [29]. Dried E. cava powder (500 g) was extracted three times with 80% methanol, followed by filtration. The filtrate was evaporated at 40℃ to obtain the methanol extract. After that, the extract was suspended in distilled water and partitioned with ethyl acetate. The ethyl acetate fraction was mixed with celite. The mixed celite was dried and packed into a glass column, and eluted in the order of hexane, methylene chloride, diethyl ether, and methanol. The diethyl ether fraction was further purified by sephadex LH-20 column chromatography using a stepwise gradient chloroform/methanol (2/1→/0/1) solvents system. The phlorotannins were purified by high performance liquid chromatography (HPLC) using a Waters HPLC system equipped with a Waters 996 photodiode array detector and C18 column (J'sphere ODS-H80, 150 × 20 mm, 4 µm; YMC Co) chromatography by stepwise elution with a methanol-water gradient (UV range: 230 nm, flow rate: 0.8 ml/min). Finally, the purified compounds were identified by comparing their 1H and 13C NMR data to the literature report.
ACE inhibitory activity was determined according to the methods of Cushman and Cheung [31] with slight modifications. For each assay, 50 µl of sample solution with 50 µl of ACE solution (25 mu/ml) were pre-incubated at 37℃ for 10 min, after which the mixture was subsequently incubated with 100 µl of substrate (25 mM Hipuril-His-Leu in 50 mM sodium borate buffer containing 500 mM NaCl at pH 8.3) at the same temperature for 60 min. The reaction was terminated by adding 250 µl of 1 M HCl. After that, the resulting hippuric acid was extracted with 500 µl of ethyl acetate. After centrifugation at 4,000 rpm for 10 min, 200 µl of the supernatant was transferred into a glass tube and dried in a dry oven at 80℃ for 1 h. The residue was dissolved in 1 ml of distilled water, and the absorbance was measured at 228 nm using an UV-specrtophotometer (Biochrom Ltd., Cambridge, CB4, OFJ, England). The extent of inhibition was calculated as follows.
% inhibition = [(Ac-As)/Ac-Ab)] × 100
Ac - Absorbance of control solution
As - Absorbance of sample solution
Ab - Absorbance of blank solution
The IC50 value was defined as the concentration of inhibitor required to inhibit 50% of ACE inhibitory activity.
To determine the ACE inhibitory pattern of the ACE inhibitor, different concentrations of dieckol (in 0.1% DMSO) were added to each reaction mixture according to the method of Bush et al. [32], with slight modifications. ACE quantity was maintained at a constant level, and the ACE inhibitory activities of the compound and substrate (HHL) were measured at various concentrations. As a control, 0.1% DMSO was used. The ACE inhibitory pattern was determined using Lineweaver-Burk plots.
The endothelial cell line EAhy926 from the inner lining of human blood vessels, was cultured in DMEM containing 10% heat-inactivated calf serum, streptomycin (100 µg/ml), and penicillin (100 unit/ml) at 37℃ in an incubator, under humidified atmosphere containing 5% CO2.
Nitric oxide is a regulator of blood pressure. Nitric oxide causes the smooth muscle cells surrounding blood vessels to relax, thereby decreasing the blood pressure. Therefore, in the present study we investigated the effect of dieckol on the production of NO in the EAhy926 cell line. Cells (1.5 × 105 cells/well) in 24-well plates were pre-incubated with different concentrations of dieckol (in 0.1% DMSO) for 24 h, and the nitrite accumulation in the supernatant was assessed by Griess reaction [33]. Each 50 µl of culture supernatant was mixed with an equal volume of Griess reagent [0.1% N-(1-naphthyl)-ethylenediamine, 1% sulfanilamide in 5% phosphoric acid] and incubated at room temperature for 10 min. The absorbance at 550 nm was measured by a microplate absorbance reader, and a series of known concentrations of sodium nitrite was used as a standard. As control 0.1% DMSO was used.
Cell viability was estimated using MTT assay according to the method described by Mosmann [34]. EAhy926 cells were seeded in a 96-well plate at a concentration of 1.0 × 105 cells/ml. After 16 h of incubation at 37℃, the cells were treated with 10 µl of the compound (in 0.1% DMSO) at different concentrations and incubated at 37℃ under a humidified atmosphere for 24 h. After that, MTT stock solution (50 µl; 2 mg/ml) was added to each of the wells to a total reaction volume of 200 µl. After 4 h of incubation, the plates were centrifuged for 5 min at 800 rpm and the supernatant aspirated. The formazan crystals in each well were dissolved in 150 µl of DMSO, and the absorbance was measured using an enzyme linked immune sorbent assay (ELISA) reader (Sunrise; Tecan Co. Ltd., Australia) at 540 nm. Relative cell viability was evaluated in accordance with the quantity of MTT converted to insoluble formazan salt. As a control, 0.1% DMSO was used. The optical density of the formazan generated in the control cells was considered to represent 100% viability. The data are expressed as mean percentages of the viable cells versus the respective control.
All the data were expressed as mean ± standard deviation (SD) of three determinations. Statistical comparison was performed via one-way analysis of variance (ANOVA) followed by Duncan's multiple range test (DMRT). P-values of less than 0.05 (P < 0.05) were considered as significant.
In the present study, the TPC showed significant differences (P < 0.05) among the five different extracts, ranging from 4.80 to 33.49% (Fig. 1). Drastically high TPC was obtained from the ethanol extract of E. cava. In addition, the value was almost 3.5-fold higher than the 2nd highest TPC obtained from the ethyl acetate extract. The results indicate a considerable variation in extraction efficiencies among the different solvents used. Further, when considering the TPC, ethanol was found to be the most efficient extractable solvent among the used organic solvents.
In the present study, the five organic extracts of E. cava were tested for their potential ACE inhibitory activities. Inhibitory activities were varied according to the extractants used (Table 1). Ethanol extract showed the highest ACE inhibitory activity compared to the other four extracts. In contrast, ethanol extract exhibited the strongest ACE inhibitory activity with the lowest IC50 value of 0.96 mg/ml (P < 0.05). As we mentioned above, the highest TPC was also obtained from the ethanol extract. Furthermore, all the other organic extracts exhibited weak inhibitory activities against ACE.
Since ethanol extract of E. cava contained ACE inhibitory compounds, it was subjected to HPLC in order to detect the potential ACE inhibitors (Fig. 2-a). We were able to identify five peaks (A-E) with potential ACE inhibitory activity. As Fig. 2-c shows, all five fractions showed potential ACE inhibitory activity, with fraction D exhibiting the strongest ACE inhibitory activity among them (P < 0.05). However, according to the results, considerable variation was observed in ACE inhibitory activities among the fractions. Therefore, ethanol extract containing the potential ACE inhibitory compounds was subjected to HPLC. As a result, five different phlorotannins, phloroglucinol, triphlorethol-A, eckol, dieckol, and eckstolonol, were purified and had different chemical structures and molecular weights (Fig. 3). According to the results of the present study, dieckol was the strongest inhibitor and IC50 value of dieckol is 2-fold lower than that of eckstolonol, which is the weakest inhibitor among the five phlorotannins (Table 2).
The ACE inhibition pattern of dieckol from E. cava was investigated using Lineweaver-Burk plots. The inhibition mode of dieckol was found to be as a non-competitive inhibitor against ACE (Fig. 4).
In the present study, the effect of dieckol on NO levels was determined in the EAhy926 cell line. Dieckol had an inducible effect on the production of NO at concentrations higher than 0.27 mM (Fig. 5) (P < 0.05). The cells showed a higher than 95% survival rate at all of the concentrations used, and the results indicate that dieckol had no cytotoxic effect on EAhy926 cells (Fig. 6).
Polyphenols are secondary metabolites very widely distributed in plants [35]. It is well known that solvents with different polarities can extract different classes of compounds [36]. Extractable polyphenols can be extracted from plants by using solvents such as water, methanol ethanol, acetone, ether, or their mixtures. Naturally occurring polyphenols are known to have numerous biological activities [37]. Further, various extractants can be used to release soluble pholyphenols from plant materials. According to previous experiments, it was also reported that water extract of E. cava has considerable ACE inhibitory activity (around 36%) at a concentration of 1 mg/ml [18]. Based on the results of the current study, we found that ethanol extract of E. cava enhanced ACE inhibition and was superior compared to water extract. It could have been that ethanol increased the TPC by inhibiting interactions between tannins and proteins during extraction [38] or even by breaking hydrogen bonds between tannin-protein complexes [39].
It is well established that brown algae contain polyphenolic compounds. Polyphenols have become the focus of intense research due to their perceived beneficial effects on health [40,41]. Phlorotannins are systematically grouped according to the bond type between the phloroglucinol units (diphenyl ethers or biphenyls) as well as the presence of additional hydroxyl groups [42]. They are secondary metabolites with a wide range of molecular sizes and an astringent taste, and they are able to bind to metal ions and precipitate proteins [43]. Based on the increasing evidence of the importance of phlorotannins, this is the first study to demonstrate ACE inhibitory activity of phlorotannins of E. cava.
Marine algae produce a great variety of secondary metabolites possessing different skeletal types and biological activities [44,45]. Accordingly, E. cava contains a variety of compounds, including carotenoids, fucoidans, and phlorotannins, showing different biological activities [29,41]. Furthermore, Ahn et al. [46] reported that phenolic secondary metabolites from E. cava have many biological activities. ACE is a carboxyl-terminal dipeptidyl exopeptidase that catalyzes the conversion of angiotesin I to angiotensin II in the rennin angiotensin system [47]. Since all enzymes are proteins, inhibition of ACE may be closely associated with the protein-binding abilities of phlorotannins, which are characteristic of all tannins. Furthermore, it has been well described that tannins have the ability to form strong complexes with proteins, either reversibly by hydrogen bonding through peptide or amide linkages or irreversibly by covalent condensations [48]. Therefore, we can suggest that the inhibition was due to the reduced efficiency of ACE after binding with phlorotannins from E. cava.
Kang et al. [49] also reported that phlorotannins components, which are mainly oligomeric polyphenols composed of phloroglucinol units, are responsible for the biological activities of Ecklonia. They identified phlorotannins such as eckol (a closed-chain trimer of phloroglucinol), 6, 6-bieckol (a hexamer), dieckol (a hexamer), and phlorofucofuroeckol (a pentamer) in Ecklonia species. Eckol, eckstolonol, and triphlorethol-A are trimers while dieckol is a hexamer of phlorolucinol units. We already mentioned that the ACE inhibitory activity of E. cava may be due to the protein-binding abilities of phlorotannin compounds. In contrast, this protein-binding ability mainly depends on the structure of the protein as well as the length and structure of the phlorotannin [50]. Furthermore, the structure of phlorotannin compounds plays a vital role in the formation of the tannin-protein complex. Stern et al. [51] also reported that tannins interact strongly with proteins; in addition, they investigated the factors affecting the precipitation of proteins by phlorotannins from three species of marine brown algae. Therefore, the ACE inhibitory activity varies according to the compound used. Furthermore, Shibata et al. [52] reported that the molecular size of phlorotannins is important for strong interaction with enzyme molecules, and they have found that pentamers or hexamers of phloroglucinols act as better inhibitors. In the present study, dieckol, which is a hexamer, showed the strongest inhibitory activity against ACE.
The observed nature of the kinetic interaction of the compound suggests that it can combine with the ACE molecule but not with the active site, thus inhibiting the activity of ACE. Phlorotannins are found to form covalent bonds with proteins; further, the protein precipitation ability of phlorotannins varies in a pH-dependent and concentration dependant manner [51]. These kinetic results also give a clear view of the ACE inhibitory activity of phlorotannins in the present study. Shibata et al. [52] reported that glycosidases from the viscera of the turban shell Turbo cornutus are inhibited by dieckol isolated from brown algae, which led them to suggest that dieckol acts as a non-competitive inhibitor of the enzyme. In addition, Tsai et al. [53] reported that captopril, the most widely used antihypertensive drug at present, shows competitive inhibition with the substrate for binding to the active site of the enzyme. However, this is the first time the mode of ACE inhibition by phlorotannins has been reported.
Nitric oxide is an important messenger molecule involved in many physiological and pathological processes within the mammalian body with both beneficial and detrimental effects [54]. The inner lining of the blood vessels uses nitric oxide to signal the surrounding smooth muscle to relax, thus resulting in vasodilation and increased blood flow. Nitric oxide is considered to be an antianginal drug as it causes vasodilation, which can help with ischemic pain known as angina by decreasing the cardiac workload. In the rennin angiotensin system, ACE inactivates the vasodilator bradykinin. ACE also cleaves the dipeptide portion of angiotensin I from its C-terminus to produce the potent vasopressor angiotensin II [6]. Recent evidence suggests that nitrates may be beneficial for treatment of angina due to reduced myocardial oxygen consumption both by decreasing preload and after load and by direct vasodilation of coronary vessels [55]. The effect of dieckol on cell viability was measured via MTT assay, which is a test of metabolic competence predicated upon the assessment of mitochondrial performance. Further, it is a colorimetric assay dependent on the conversion of yellow tetrazolium bromide to its purple formazan derivative by mitochondrial succinate dehydrogenase in viable cells [34].
In conclusion, E. cava is a very interesting resource due to the presence of unique phlorotannin derivatives with special bioactivities, including ACE inhibitory activity. Ethanol enhances the extraction of phlorotannins, especially dieckol, from the brown seaweed E. cava. In contrast, in this study, phloroglucinol derivative dieckol exhibited the strongest activity against ACE. Based on the results of this study, we can suggest that the brown seaweed E. cava could be used for the development of promising and potential functional food products. Moreover, it is expected that these findings will contribute to basic research and potential applications of phlorotannins in relevant fields.
References
1. Amenta F, Mignini F, Rabbia F, Tomassoni D, Veglio F. Protective effect of anti-hypertensive treatment on cognitive function in essential hypertension: Analysis of published clinical data. J Neurol Sci. 2002; 203-204:147–151. PMID: 12417374.
2. Lee SH, Quian ZJ, Ki SK. A novel angiotensin I converting enzyme inhibitory peptide from tuna frame protein hydrolysate and its antihypertensive effect in spontaneously hypertensive rats. Food Chem. 2010; 118:96–102.


3. Jung WK, Mendis E, Je JY, Park PJ, Son BW, Kim HC, Choi YK, Kim SK. Angiotensin I-converting enzyme inhibitory peptide from yellowfin sole (Limanda aspera) frame protein and its antihypertensive effect in spontaneously hypertensive rats. Food Chem. 2006; 94:26–32.
4. Bougatef A, Nedjar-Arroume N, Ravallec-Plé R, Leroy Y, Guillochon D, Barkia A, Nasri M. Angiotensin I-converting enzyme (ACE) inhibitory activities of Sardinelle (Sardinella aurita) by-products protein hydrolysates obtained by treatment with microbial and visceral fish serine proteases. Food Chem. 2008; 111:350–356.
5. Park EJ, Jhon DY. The antioxidant, angiotensin converting enzyme inhibition activity, and phenolic compounds of bamboo shoot extracts. LWT-Food Sci Technol. 2010; 4:655–659.


6. Kim JE, Hwang K, Lee SP. ACE inhibitory and hydrolytic enzyme activities in textured vegetable protein in relation to the solid state fermentation period using Bacillus subtilis HA. Food Sci Biotechnol. 2010; 19:487–495.
7. Kim SK, Byun HG, Park PJ, Shahidi F. Angiotensin I converting enzyme inhibitory peptides purified from bovine skin gelatin hydrolysate. J Agric Food Chem. 2001; 49:2992–2997. PMID: 11409999.


8. Lee JK, Hong S, Jeon JK, Kim SK, Byun HG. Purification and characterization of angiotensin I converting enzyme inhibitory peptides from the rotifer, Brachionus rotundiformis. Bioresour Technol. 2009; 100:5255–5259. PMID: 19540110.
9. Andrews PR, Carson JM, Caselli A, Spark MJ, Woods R. Conformational analysis and active site modeling of angiotensin-converting enzyme inhibitors. J Med Chem. 1985; 28:393–399. PMID: 2983076.


10. López-Fandiñoa R, Otte J, van Camp J. Physiological, chemical and technological aspects of milk-protein-derived peptides with antihypertensive and ACE-inhibitory activity. Int Dairy J. 2006; 16:1277–1293.
11. Pripp AH, Sørensen R, Stepaniak L, Sørhaug T. Relationship between proteolysis and angiotensin-I-converting enzyme inhibition in different cheeses. LWT-Food Sci Technol. 2006; 39:677–683.


12. Miguel M, Alonso MJ, Salaices M, Aleixandre A, López-Fandiñoa R. Antihypertensive, ACE-inhibitory and vasodilator properties of an egg white hydrolysate: Effect of a simulated intestinal digestion. Food Chem. 2007; 104:163–168.


13. Wu J, Aluko RE, Muir AD. Production of angiotensin I-converting enzyme inhibitory peptides from defatted canola meal. Bioresour Technol. 2009; 100:5283–5287. PMID: 19570676.


14. Quist EE, Phillips RD, Saalia FK. Angiotensin converting enzyme inhibitory activity of proteolytic digests of peanut (Arachis hypogaea L.) flour. LWT-Food Sci Technol. 2009; 42:694–699.
15. Marczak ED, Usui H, Fujita H, Yang Y, Yokoo M, Lipkowski AW, Yoshikawa M. New antihypertensive peptides isolated from rapeseed. Peptides. 2003; 24:791–798. PMID: 12948830.


16. Karawita R, Park PJ, Siriwardhana N, Jeon BT, Moon SH, Ahn DK, Cho SK, Jeon YJ. Angiotensin I-converting enzyme (ACE) inhibitory activity of elk (Cervus elaphus) velvet Antler. J Food Sci Nutr. 2005; 10:239–243.
17. Nakajima K, Yoshie-Stark Y, Ogushi M. Comparison of ACE inhibitory and DPPH radical scavenging activities of fish muscle hydrolysates. Food Chem. 2009; 114:884–851.


18. Athukorala Y, Jeon YJ. Screening for angiotensin 1-converting enzyme inhibitory activity of Ecklonia cava. J Food Sci Nutr. 2005; 10:134–139.
19. Spence JT. Challenges related to the composition of functional foods. J Food Compost Anal. 2006; 19:S4–S6.


20. Cha MH, Lee J, Song MJ. Dieticians' intentions to recommend functional foods: The mediating role consumption frequency of functional foods. Nutr Res Pract. 2010; 4:75–81. PMID: 20198212.
21. Li Y, Qian ZJ, Ryu B, Lee SH, Kim MM, Kim SK. Chemical components and its antioxidant properties in vitro: An edible marine brown alga, Ecklonia cava. Bioorg Med Chem. 2009; 17:1963–1973. PMID: 19201199.
22. Oyamada K, Tsukidate M, Watanabe K, Takahashi T, Isoo T, Terawaki T. A field test of porous carbonated blocks used as artificial reef in seaweed beds of Ecklonia cava. J Appl Phycol. 2008; 20:863–868.
23. Shahidi F. Nutraceuticals and functional foods: whole versus processed foods. Trends Food Sci Technol. 2009; 20:376–387.


24. Kim MM, Ta QV, Mendis E, Rajapakse N, Jung WK, Byun HG, Jeon YJ, Kim SK. Phlorotannins in Ecklonia cava extract inhibit matrix metalloproteinase activity. Life Sci. 2006; 9:1436–1443. PMID: 16737716.
25. Lee SH, Han JS, Heo SJ, Hwang JY, Jeon YJ. Protective effects of dieckol isolated from Ecklonia cava against high glucose-induced oxidative stress in human umbilical vein endothelial cells. Toxicol In Vitro. 2010; 24:375–381. PMID: 19896528.
26. Zhang Q, Zhang J, Shen J, Silva A, Dennis DA, Barrow C. A simple 96-well microplate method for estimation of total polyphenol content in seaweeds. J Appl Phycol. 2006; 18:445–450.


27. Koivikko R, Loponen J, Honkanen T, Jormalainen V. Contents of soluble, cell-wall-bound and exuded phlorotannins in the brown alga Fucus vesiculosus, with implications on their ecological functions. J Chem Ecol. 2005; 31:195–212. PMID: 15839490.
28. Kang KA, Lee KH, Park JW, Lee NH, Na HK, Surh YJ, You HJ, Chung MH, Hyun JW. Triphlorethol-A induces heme oxygenase-1 via activation of ERK and NF-E2 related factor 2 transcription factor. FEBS Lett. 2007; 581:2000–2008. PMID: 17467702.


29. Heo SJ, Ko SC, Cha SH, Kang DH, Park HS, Choi YU, Kim D, Jung WK, Jeon YJ. Effect of phlorotannins isolated from Ecklonia cava on melanogenesis and their protective effect against photo-oxidative stress induced by UV-B radiation. Toxicol In Vitro. 2009; 23:1123–1130. PMID: 19490939.
30. Chandler SF, Dodds JH. The effect of phosphate, nitrogen, and sucrose on the production of phenolics and solasidine in callus cultures of Solanum laciniatum. Plant Cell Rep. 1983; 2:105–110.
31. Cushman DW, Cheung HS. Spectrophotometric assay and properties of the angiotensin-converting enzyme of rabbit lung. Biochem Pharmacol. 1971; 20:1637–1648.


32. Bush K, Henry PR, Slusarchyk DS. Muraceins-Muramyl peptides produced by Nocardia Orientalis as angiotensin-converting enzyme inhibitors. J Antibiot (Tokyo). 1984; 37:330–335. PMID: 6327590.
33. Nath J, Powledge A. Modulation of human neutrophil inflammatory responses by nitric oxide: studies in unprimed and LPS-primed cells. J Leukoc Biol. 1997; 62:805–816. PMID: 9400822.


34. Mosmann T. Rapid colorimetric assay for cellular growth and survival: Application to proliferation and cytotoxicity assays. J Immunol Methods. 1983; 65:55–63. PMID: 6606682.


35. Shibata T, Yamaguchi K, Nagayama K, Kawaguchi S, Nakamura T. Inhibitory activity of brown algal phlorotannins against glycosidases from the viscera of the turban shell Turbo cornutus. Eur J Phycol. 2002; 37:493–500.
36. Xu ML, Wang L, Hu JH, Lee SK, Wang MH. Antioxidant activities and related polyphenolic constituents of the methanol extract fractions from Broussonetia papyrifera stem bark and wood. Food Sci Biotechnol. 2010; 19:677–682.
37. Handique JG, Baruah JB. Polyphenolic compounds: an overview. React Funct Polym. 2002; 52:163–188.


38. Hagerman AE. Extraction of tannin from fresh and preserved leaves. J Chem Ecol. 1988; 14:453–461.


39. Porter LJ. Rowe JW, editor. Condensed tannins. Natural products of woody plants I. 1989. Berlin, Germany: Springer-Verlag;p. 651–690.


40. Loganayaki N, Siddhuraju P, Manian S. Antioxidant activity of two traditional Indian vegetables: Solanum nigrum L. and Solanum torvum L. Food Sci Biotechnol. 2010; 19:121–127.
41. Lee SH, Ko SC, Kang SM, Cha SH, Ahn GN, Um BH, Jeon YJ. Antioxidative effect of Ecklonia cava dried by far infrared radiation drying. Food Sci Biotechnol. 2010; 19:129–135.
42. Glombitza KW, Hauperich S. Phlorotannins from the brown alga Cystophora torulosa. Phytochemistry. 1997; 46:735–740.
43. Lüder UH, Clayton MN. Induction of phlorotannins in the brown macroalga Ecklonia radiata (Laminariales, Phaeophyta) in response to simulated herbivory--the first microscopic study. Planta. 2004; 218:928–937. PMID: 14716562.
44. Heo SJ, Jeon YJ. Radical scavenging capacity and cytoprotective effect of enzymatic digests of Ishige okamurae. J Appl Phycol. 2008; 20:1087–1095.
45. You JS, Sung MJ, Chang KJ. Evaluation of 8-week body weight control program including sea tangle (Laminaria japonica) supplementation in Korean female college students. Nutr Res Pract. 2009; 3:307–314. PMID: 20098584.


46. Ahn GN, Kim KN, Cha SH, Song CB, Lee J, Heo MS, Yeo IK, Lee NH, Jee YH, Kim JS, Heu MS, Jeon YJ. Antioxidant activities of phlorotannins purified from Ecklonia cava on free radical scavenging using ESR and H2O2-mediated DNA damage. Eur Food Res Technol. 2007; 226:71–79.
47. Torruco-Uco J, Chel-Guerrero L, Martínez-Ayala A, Dávila-Ortíz G, Betancur-Ancona D. Angiotensin-I converting enzyme inhibitory and antioxidant activities of protein hydrolysates from Phaseolus lunatus and Phaseolus vulgaris seeds. LWT-Food Sci Technol. 2009; 42:1597–1604.
48. Appel HM. Phenolics in ecological interactions-the importance of oxidation. J Chem Ecol. 1993; 19:1521–1552.
49. Kang KA, Lee KH, Chae S, Zhang R, Jung MS, Lee Y, Kim SY, Kim HS, Joo HG, Park JW, Ham YM, Lee NH, Hyun JW. Eckol isolated from Ecklonia cava attenuates oxidative stress induced cell damage in lung fibroblast cells. FEBS Lett. 2005; 579:6295–6304. PMID: 16253238.
50. Haslam E. Natural polyphenols (Vegetable tannins) as drugs: possible modes of action. J Nat Prod. 1996; 59:205–215. PMID: 8991956.


51. Stern JL, Hagerman AE, Steinberg PD, Mason PK. Phlorotannin-protein interactions. J Chem Ecol. 1996; 22:1877–1899.


52. Shibata T, Yamaguchi K, Nagayama K, Kawagushi S, Nakamura T. Inhibitory activity of brown algal phlorotannins against glycosidases from the viscera of the turban shell Turbo cornutus. Eur J Phycol. 2002; 37:493–500.
53. Tsai JS, Lin TC, Chen JL, Pan BS. The inhibitory effects of freshwater clam (Corbicula fluminea, Muller) muscle protein hydrolysates on angiotensin I converting enzyme. Process Biochem. 2006; 41:2276–2281.
54. Hou YC, Janczuk A, Wang PG. Current trends in the development of nitric oxide donors. Curr Pharm Des. 1999; 5:417–441. PMID: 10390607.
55. Abrams J. Beneficial actions of nitrates in cardiovascular disease. Am J Cardiol. 1996; 77:31C–37C. PMID: 8540453.


Fig. 1
Total phenolic content (TPC) of different organic extracts from the brown seaweed E. cava. Values are mean ± SD of three determinations. Values with different alphabets are significantly different at P < 0.05 as analyzed by Duncan's multiple range test (DMRT).
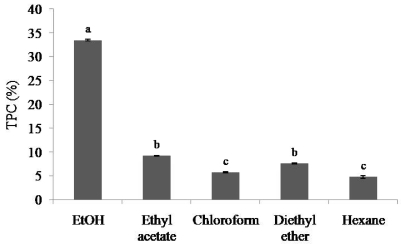
Fig. 2
HPLC chromatogram for ethanol extracts of E. cava. A Hypersil-Goold C18 column (100 mm × 2.1 mm, 1.9 µm) was used. Separation was performed with a gradient from 10-100% in 40 min at a flow rate of 0.2 ml/min. Elution was monitored at 230 nm (a). Standard chromatogram for dieckol isolated from E. cava (b). Fractions showing ACE inhibitory activity were designated as A-E, and the sample concentration was 0.125 mg/ml (c). Values are mean ± SD of three determinations. Values with different alphabets are significantly different at P < 0.05 as analyzed by Duncan's multiple range test (DMRT).
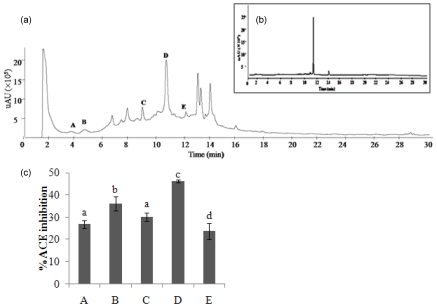
Fig. 3
The structures of phlorotannins isolated from E. cava. (a) phloroglucinol, (b) eckol, (c) triphlorethol-A, (d) dieckol, and (e) eckstolonol
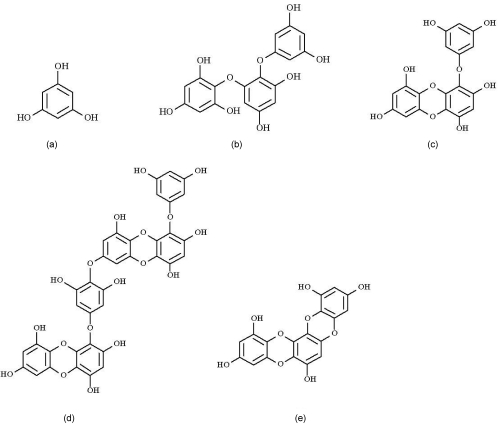
Fig. 4
Lineweaver-Burk plots of ACE inhibitory activity in the presence of dieckol. Values are mean ± SD of three determinations.
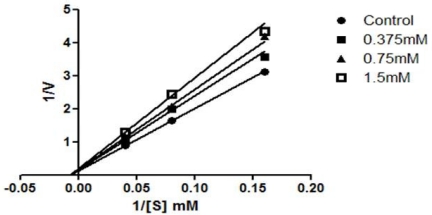
Fig. 5
Effect of dieckol on NO levels in EAhy926 cells. Values are mean ± SD of three determinations. Values with different alphabets are significantly different at P < 0.05 as analyzed by Duncan's multiple range test (DMRT).
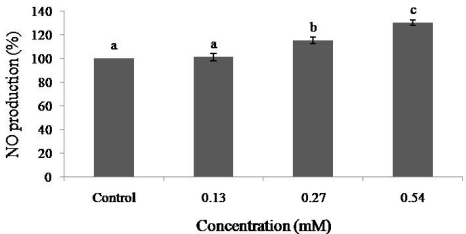
Fig. 6
Effect of dieckol on cell cytotoxicity in EAhy926 cells. The viability of cells upon dieckol treatment was determined by MTT assay. Values are mean ± SD of three determinations. Values with different alphabets are significantly different at P < 0.05 as analyzed by Duncan's multiple range test (DMRT).
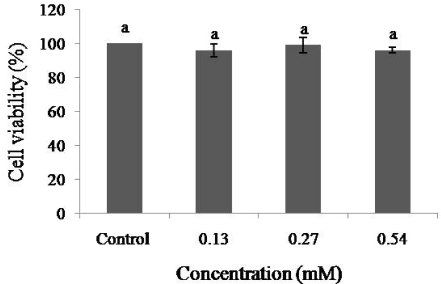