Abstract
At present, lifestyle-related diseases are one of the most critical health issues worldwide. It has been reported that lipopolysaccharide derived from a Gram-negative bacteria (IP-PA1) symbiotic with wheat exhibited several advantageous biological effects, such as the reduction of plasma glucose levels in NOD mice and low-density lipoprotein (LDL) levels in WHHL rabbits. In this study, the beneficial effects on plasma glucose and lipids of a tea (SI tea) consisting of IP-PA1 and Salacia (which contains an inhibitor of α-glucosidase) were investigated in the KK-Ay/TaJcl type 2 diabetic model mice and in human subjects with premetabolic syndrome in a double-blind, randomized study. SI tea significantly decreased plasma glucose levels in KK-Ay/TaJcl mice. A clinical trial of SI tea was performed with 41 subjects between the ages of 40 and 69, who belonged either to a high plasma glucose group (HG: FPG 100-125 mg/dl) or to a hyperlipidemia group (HL: TG ≥ 150 mg/dl, or LDL ≥ 120 mg/dl, or HDL < 40 mg/dl). These subjects ingested either Salacia without IP-PA1 (the control) or SI tea. Blood samples were collected at 0, 30, and 60 days after initiating SI tea treatment, and were measured for FPG, HbA1c, TG, LDL, and HDL. These results showed that SI tea reduced FPG and HbA1c more rapidly than the control in the HL group, and also significantly improved LDL and HDL levels in the HG group. Thus, SI tea may be helpful in preventing lifestyle-related diseases.
Lifestyle-related diseases, particularly diabetes, are rapidly increasing in advanced countries because of a change in lifestyle and social environment [1]. In Japan, the number of people suffering from diabetes is estimated to be approximately 22.1 million, including those with suspected diabetes, and this value is predicted to increase in the future [2]. Over 95% of the cases are type 2 diabetes, a disease that is exacerbated by excessive dietary intake, lack of exercise, and increased stress [3]. It is clear that type 2 diabetes, which is accompanied by obesity and visceral fat accumulation, has a high risk of being associated with dyslipidemia and hypertension. While type 2 diabetes can be prevented by healthy diets and adequate exercise, the number of diabetics is increasing at present because of the difficulty in maintaining healthy diets or adequate exercise.
Salacia, which has been traditionally used in Indian Ayurvedic medicine, is effective for the prevention and treatment of diabetes [4]. The active ingredients are α-glucosidase inhibitors such as salasinol [5,6] and kotakanol [7]. These substances inhibit the breakdown of oligosaccharides and polysaccharides into monosaccharides by competitive inhibition of α-glucosidase activity within the lumen of the intestinal tract, which inhibits the rapid increase of plasma glucose after meals [8]. Diabetes is often associated with visceral fat accumulation, dyslipidemia, and hypertension [9,10]. Diabetics with these symptoms use different medicines for the treatment of each symptom. This gives rise to many treatment problems because of the synergistic side effects of the numerous medicines used [3]. For the prevention of diabetes, it is important to improve not only plasma glucose levels but to also decrease visceral fat accumulation, plasma lipid levels, and blood pressure levels. Salacia was reported to improve obesity and decrease triglyceride and cholesterol levels in mice and rats [11,12]. However, not much information on these effects in humans, except for a report on the improvement of plasma glucose levels, is available [8].
The innate immune system plays an essential role in the maintenance of animal health and the prevention of various diseases. The appropriate activation of macrophages plays a central role in innate immunity and functions not only for defense against infections but also for the maintenance of homeostasis (such as the elimination of foreign substances and metabolic control) [13]. We identified a lipopolysaccharide (LPS) (molecular weight approximately 5 kD) derived from a symbiotic Gram-negative bacteria, Pantea agglomerans [14]. This LPS was capable of activating macrophages in a water extract of wheat flour [15] and was designated IP-PA1, which is an abbreviation of "immune potentiator from P. agglomerans" [13]. Under certain limited conditions, LPS may act as an endotoxin, causing severe systemic inflammation, but more commonly, it has beneficial effects. LPS functions that have been recently discovered include the regulation of intestinal homeostasis, such as preventing enteric infectious diseases or the regulation of enteric immunity through interactions with gut-associated lymphoid tissue [16,17], modulation of proliferation and differentiation of the epithelial lineages [18], and regulation of angiogenesis [19] by oral administration. Thus, LPS is now recognized as an exohormone rather than simply an endotoxin [20].
It has been demonstrated that several foods contain Gram-negative bacteria or LPS. P. agglomerans is found not only in wheat but also in other food plants, such as rice [21], sweet potatoes [22], apples, and pears [23]. Moreover, it has been demonstrated that growth of P. agglomerans is mandatory for the fermentation of rye sourdough because it produces and provides folicacid to Lactobacillus. Considering this information and the long history of human intake, the bacteria P. agglomerans is believed to be nonpathogenic. In addition, in Europe, live P. agglomerans is used as a biocontrol agent for preventing fungal spoilage of fruit [24]. This suggests that IP-PA1 is a safe substance, and this has been confirmed with a conventional oral-administration safety test [13].
Previous studies in animals and human clinical trials reported suppressive effects of IP-PA1 on IgE-dependent allergies and an analgesic effect on the acetic acid writhing test [13]. In addition, IP-PA1 was also reported to delay the occurrence of type 1 diabetes in mice [25] and decrease low-density lipoprotein (LDL) levels in familial hyperlipidemia [26]. However, it is not yet clear whether IP-PA1 is able to suppress type 2 diabetes. This is because the etiology and therapeutic approaches for diabetics are quite different for type 1 and type 2 diabetes. Also, it is not yet clear whether IP-PA1 improves diabetes associated with dyslipidemia. Thus, in this study, a tea (SI tea) was prepared that combined IP-PA1 (a regulator of immunity) and Salacia (an inhibitor of α-glucosidase), with the expectation that a beneficial effect would be observed in cases of diabetes and dyslipidemia. SI tea was first tested to determine whether it decreased the plasma glucose in KK-Ay/TaJcl mice (type 2 diabetes model mice). It was then tested to determine if it improved HbA1c values, blood glucose values, or LDL values in blood lipids of humans. This study used a randomized and double-blind design. Subjects had a routine general diet and test tea 2 times/day.
Salacia oblonga (Salacia) tea was prepared by Takama Co., Ltd, Japan, and the fermented flour containing 1% IP-PA1 was prepared by Macrophi Inc., Japan. IP-PA1 is a bacterial LPS that is derived from P. agglomerans. SI tea was a combination of Salacia tea, fermented flour, and vitamins. The C vitamin and B-group vitamins were premixed (B1, 0.32 mg/ml; B2, 0.27 mg/ml; B6, 0.28 mg/ml; B12, 0.75 µg/ml; B3, 1.63 mg/ml; B5, 0.96 mg/ml; and C, 7.5 mg/ml). The amount of vitamin premix was not expected to have any effect by itself on diabetes and hyperlipidemia [27,28]. In this study, 1 g of SI tea containing 20 mg/g of fermented flour extract (200 µg/g as IP-PA1) was used by dissolving in 100 ml of ambient or hot water. The control was Salacia tea without the fermented flour extract (Table 1).
Five-week-old male KK-Ay/TaJcl mice were obtained from CLEA Japan, Inc. (Tokyo, Japan), and the experiments lasted for 12 weeks after 1 week of acclimatization. The mice were maintained in a temperature-controlled room at 24 ± 2℃ and 50%-70% humidity with a 12-h light cycle (light from 06:00 to 18:00). The mice were housed (1 per cage), and had access to tap water and laboratory food ad libitum (CE-2; CLEA Japan, Inc.). The mice were divided into three groups: distilled water (DW; n = 10), Salacia tea (control; n = 10), and SI tea (n = 11). The oral daily dose administered for IP-PA1 was 10 µg/kg body weight and for Salacia was 3 mg/kg body weight, accomplished by drinking SI tea for 12 weeks. Body weight and fasting plasma glucose (FPG) levels were measured every week during the course of the study for 12 weeks. FPG was measured after an overnight fast of at least 10 hours. Mice were immobilized with a stereotaxic apparatus. Blood glucose levels were determined by measuring the absorbance using an Accu-Chek Aviva (Roche). This study was approved by the Laboratory Animal Committee of Kagawa University; the mice were maintained in accordance with the Guide for Animal Experiments at Kagawa University.
Forty-seven subjects (age range, 40-69 years) from a clinic in Kagawa participated in this study. The high-plasma glucose (HG) group consisted of 14 subjects whose FPG levels were 100-125 mg/dl; the hyperlipidemia (HL) group consisted of 33 subjects whose triglyceride (TG) levels were higher than 150 mg/dl, or whose LDL levels were higher than 120 mg/dl, or whose HDL levels were lower than 40 mg/dl (Table 2). This study was randomized in each group, and conducted using a double-blinded design. Of these, 3 subjects being treated for hyperlipidemia or hypertension were excluded from the study because of their poor physical condition or deconditioning during the intake period. The study protocol was approved by the Ethics Committee of the Non-profit Organization, Linking Setouchi Innate Immune Network, and was carried out in accordance with the principles of the Declaration of Helsinki. Procedures were carefully explained to the participants, and written informed consent was obtained from them.
This study initiated on October 1, 2008, and continued for 60 days. It was conducted by a double-blinded method, randomized by envelopes. Subjects ingested the routine general diet and test tea 2 times/day, and their intake time and physical conditions were recorded each day. To measure glucose metabolism markers (FPG and HbA1c) and lipid metabolism markers (TG, LDL, and HDL), blood samples were collected on days 0, 30, and 60. Subjects fasted after 22:00 on days before blood collection.
Data are expressed as mean ± SD. Statistical analyses were performed using Excel Statistics 2008 (Microsoft, USA). In the animal experiment, differences between the DW group, the Salacia tea control group, and the SI tea group were analyzed using the Tukey-Kramer multiple comparison post tests. In a clinical trial, differences between day 0 and day 30, or day 0 and day 60, were compared using the Tukey-Kramer multiple comparison post tests. Differences with P < 0.05 were considered to be significant.
Before these clinical experiments, antidiabetic effects of SI tea were investigated using KK-Ay/TaJcl type 2 diabetic model mice. The mice had free access to tap water and laboratory food. The body weights for each group before intake were as follows: the DW group, 26.39 ± 1.07 g; the control group, 26.69 ± 1.19 g; and the SI tea group 26.84 ± 1.81 g. After 12 weeks, the body weights for each group increased in all groups as follows: the DW group, 45.64 ± 3.45 g; the control group, 45.47 ± 3.22 g; and the SI tea group 44.75 ± 3.18 g (Fig. 1). FPG levels for each group before intake were as follows: the DW group, 93.6 ± 17.6 mg/dl; the control group, 95.7 ± 18.1 mg/dl; and the SI tea group, 103.5 ± 60.9 mg/dl. After 2 weeks from the start of the experiment, FPG levels for each group were as follows: DW > control > SI tea, and this order did not change during the rest of the experiment as shown in Fig. 2. The average FPG level of each group in all periods of intake was: DW group, 118.5 ± 24.0 mg/dl; control group, 108.9 ± 24.0 mg/dl; and SI tea group, 98.5 ± 21.5 mg/dl (Fig. 2). FPG levels in DW and the control (Salacia tea) group showed a tendency to increase; while in the SI tea group, they decreased slightly. These results indicate that a combination of fermented wheat flour with IP-PA1 and Salacia effectively decreased FPG in type 2 diabetic mice more than with Salacia tea alone.
The above-mentioned animal data indicates that SI tea improved blood glucose levels in KK-Ay/TaJcl type 2 diabetic-model mice more than Salacia tea. Moreover, we previously found that IP-PA1 decreases LDL levels in hyperlipidemic rabbits (WHHL) [26]. We then investigated the anti-diabetic effect of SI tea in human volunteers with high-plasma glucose (HG group) or hyperlipidemia (HL group). Characteristics of each group before the clinical trial are shown in Table 3. While this study was randomized to the control or SI tea in the HG group and HL group, the body fat in the HG control group was higher than in other groups. However, the change of body fat was not significantly different for all groups.
First, the effect of SI tea on the improvement of FPG levels in human HG and HL groups was examined. Fig. 3 shows the change in glucose metabolism markers over the 60-day period. FPG levels in each group before initiating the intake were as follows: the HG control group, 105.2 ± 7.6 mg/dl; the HG SI tea group, 105.4 ± 5.8 mg/dl; the HL control group, 92.3 ± 3.0 mg/dl; and the HL SI tea group, 91.8 ± 5.5 mg/dl. Sixty days after initiating the intake of teas, the FPG values were as follows: the HG control group, 98.7 ± 6.3 mg/dl; the HG SI tea group, 103.7 ± 16.2 mg/dl; the HL control group, 87.2 ± 6.2 mg/dl; and the HL SI tea group, 85.0 ± 6.7 mg/dl. Thus, the level of FPG in the SI tea group did not change. However, the FPG level of the HL group that consumed SI tea showed a significant decrease earlier than the control. HbA1c levels in each group before initiating the intake were as follows: the HG control group, 5.2% ± 0.6%; the HG SI tea group, 5.2% ± 0.3%; the HL control group, 4.9% ± 0.3%; and the HL SI tea group, 5.0% ± 0.2%. Sixty days after the intake of teas, the HbA1c values were as follows: the HG control group, 5.0% ± 0.6%; the HG SI tea group, 5.0% ± 0.4%; the HL control group, 4.9% ± 0.3%; and the HL SI tea group, 4.9% ± 0.2%. As with FPG, the level of HbA1c in the HG group was almost the same in the control and SI tea groups. In contrast, the level of HbA1c in the HL group significantly decreased after 30 days in the SI tea group. These results suggest that IP-PA1 promoted a decrease of this glucose metabolism marker when combined with Salacia tea. In addition, this combination effect of IP-PA1 with Salacia was more prominent in the HL group than in the HG group.
Next, we investigated the changes in lipid metabolism markers. Table 3 shows that before the experiment, the TG level in the HL control group was high, 152.5 ± 80.3 mg/dl. Also, the LDL level was high in all of groups: the HG control group, 140.0 ± 26.9 mg/dl; the HG SI tea group, 136.1 ± 29.9 mg/dl; the HL control group, 140.8 ± 31.7 mg/dl; and the HL SI tea group, 148.1 ± 32.2 mg/dl. The TG level did not change in any of the groups. However, the TG level in the HL group that consumed SI tea and Salacia tea showed a tendency to decrease (Fig. 4). The LDL level for those who consumed SI tea decreased both in the HG group and the HL group after 60 days, 122.1 ± 34.6 mg/dl and 142.1 ± 39.1 mg/dl, respectively. The HDL level significantly increased in the HG group after consuming SI tea for 30 days (58.1 ± 13.0 mg/dl). As a result of the study on TG, LDL, and HDL values, it was confirmed that LDL values, which were higher than normal levels before the trial, had decreased significantly after administration of SI tea.
In Japan, over 95% of diabetics have type 2 diabetes. While suppressive effects of IP-PA1 on the occurrence of diabetes development in NOD type 1 diabetic-model mice have been reported [25], the effect of IP-PA1 on type 2 diabetes is still unclear. This is why we investigated the beneficial effects of SI tea on FPG levels in a type 2 diabetic mouse model (KK-Ay/TaJcl mice), which is based on a lifestyle disease. The result of body weight change was confirmed to increase in all groups, and it was not different among the groups. It was reported that there was a slight weight loss in mice and rats that were fed with Salacia [11,12]. In another study, after Salacia intake for 3 weeks, it was reported that body weight did not differ between the control and Salacia groups [29]. Thus, the addition of IP-PA1 did not induce abrupt body weight change similar to Salacia alone, and if our study was prolonged, it may have been different between the control group and the SI group. The results indicated that levels of FPG in DW and control (Salacia tea) groups gradually tended to increase but slightly decreased in the SI tea group (Fig. 2). Since FPG levels of the control Salacia tea group showed a tendency to increase, this suggests that SI tea has the potential to improve type 2 diabetes and that this effect is superior to Salacia tea alone.
Salacia tea reduces high blood glucose levels because it contains salacinol and kotalanol, both of which are inhibitors of α-glucosidase. It is known that guava polyphenol, mulberry, and Gymnema also have similar inhibitory activity against α-glucosidase but Salacia is the most effective [30]. While the antidiabetic mechanism of IP-PA1, a macrophage-activating substance, remain elusive, macrophage activation has been reported to be effective in treatment of type 1 and type 2 diabetes [31-34].
Type 1 diabetes is caused by the progressive loss of insulin-producing pancreatic β cells by inducing auto-reactive cytotoxic T lymphocytes [35]. Moreover, it has recently been demonstrated that killer T cells initiate an inflammatory cascade within the adipose tissue, and that this induces insulin resistance in type 2 diabetes [36,37]. Therefore, type 1 and type 2 diabetes are similar inflammatory diseases.
Recently, two different states of macrophages have been defined: the M1 (or classically activated) and M2 (or alternatively activated) macrophages [38]. M1-activated macrophages typically produce pro-inflammatory cytokines (tumor necrosis factor (TNF)-α, interleukin (IL)-1β). M2 macrophages increase anti-inflammatory cytokines (IL-10) and arginase 1 activity. Functionally, M2 macrophages down-regulate inflammatory responses and promote tissue repair. Adipose tissue macrophages in obesity induce an inflammatory reaction (such as TNF secretion) in cytokines, whereas macrophages in healthy adipose tissue release anti-inflammatory cytokines such as IL-10 and TGF-β [39,40]. We found an anti-inflammatory response to IP-PA1 in atopic dermatitis experiments [13]. Porta et al. [41] reported that M2 macrophages are induced by a small amount of LPS for a long period. In animal models, nicotine treatment reduces the incidence of type 1 diabetes animal models by increasing the pancreatic levels of the M2 cytokines IL-4 and IL-10 [42]. These results suggest that M2 type macrophages released IL-10 from macrophage activated by IP-PA1, suppressing the inflammatory response. Details of the mode of action for the anti-diabetic effect of IP-PA1 requires further investigation, such as the measurement of diabetes-associated markers (TNF-α, IL-1β and IL-10), insulin, and leptin.
The improvement in human plasma glucose levels is shown in Fig. 3. To examine the preventive effect on diabetes, non-diabetic subjects were chosen with high FPG levels (HG: FPG 100-125 mg/dl). The results indicate that SI tea did not change FPG levels, although it showed a tendency to reduce HbA1c levels. Type 2 diabetes is often accompanied by obesity and dyslipidemia. The most common pattern of diabetes is characterized by the secretion of TNF-α from adipose tissue because of dyslipidemia, which leads to impaired insulin sensitivity and impaired glucose tolerance (IGT) [43]. As stated above, in healthy individuals with normal body weight, macrophages are present in moderate quantities in adipose tissue, in which inflammatory signals are low or nonexistent. On the other hand, the adipose tissue macrophages in obese individuals produce pro-inflammatory cytokines and induce IGT [44]. It is suggested that macrophages are either suppressors or enhancers in the development of diabetes. However, several issues are still unclear about the mechanism that regulates the differentiation of macrophages, and the relationship between the onset of diabetes and macrophage behavior.
Our previous study showed that the serum cholesterol level in WHHL rabbits decreased with the uptake of IP-PA1 [26]. Kishino et al. [12] indicated that Salacia improves obesity and decreases plasma lipid levels by lipase inhibition in mice and rats. Thus, it was expected that herbal tea containing Salacia and IP-PA1 would improve not only plasma glucose but also serum cholesterol levels in subjects with dyslipidemia. We investigated the SI tea effects on plasma glucose levels and plasma lipid levels in a group of subjects which consisted not only of a high glucose group but also a high lipid group (TG: ≥ 150 mg/dl, or LDL: ≥ 120 mg/dl, or HDL: < 40 mg/dl). As shown in Fig. 3, significant changes in levels of FPG and HbA1c were observed in the HL group as in the HG group. Especially, the FPG level of the HL group that consumed SI tea was significantly lower than that of the HG group. In this study, the FPG level of the HL group stayed within the normal level. However, even if the FPG level is normal, Japanese people tend to develop IGT because their insulin secretion ability is lower than in other ethnic populations [45]. High IGT levels increase the risk of occurrence of diabetes and arteriosclerosis to a greater extent than high FPG levels [46,47]. Moreover, a correlation between a decrease in IGT and FPG was reported [48]. Since a significant effect of decreasing plasma glucose levels due to SI tea was observed in the HL group but not the HG group, it is believed that SI tea may be effective for IGT. This would not be obvious from the FPG values alone. SI tea significantly reduced LDL levels in the plasma lipid of both HG and HL groups. In addition, there was a significant increase in the HDL level of HG groups that consumed SI tea. LDL levels of all subjects from all groups before the experiment were high (Table 3). While in the present study, a change in obesity was not observed with Salacia (Table 3), Akase et al. [11] reported that Salacia decreased body weight and visceral fat in mice and rats. The difference in the results was most likely because of the difference between a regulated rodent model and a human experiment in which it is difficult to control food and life style.
In conclusion, SI tea not only decreased FPG levels more effectively than Salacia tea alone but it also improved LDL values. Thus, it can be anticipated that SI tea will be used as a preventive food for lifestyle-related diseases, such as diabetes, obesity, and dyslipidemia.
Figures and Tables
Fig. 2
Change in plasma glucose levels by SI tea in KK-Ay/TaJcl mice. The values are means ± SD. a) Differences between the DW group and the Salacia tea control group, or the DW group and the SI tea group were analyzed using the Tukey-Kramer multiple comparison post tests at P < 0.01. b) Differences between the Salacia tea control group and the SI tea group were analyzed using the Tukey-Kramer multiple comparison post-tests at SI, P < 0.01.
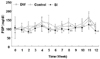
Fig. 3
Change in glucose metabolism markers. Effect of SI tea on FPG and HbA1c in humans during a 60-day period. ▪: HG control; ♦: HG SI tea; □: HL control; ⋄: HL SI tea. Values are mean ± SD. Differences in 30 day- or 60 day-values adjusted for variation in 0 day-values using the Tukey-Kramer multiple comparison post-tests. *P < 0.05, **P < 0.01.
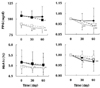
Fig. 4
Change in lipid metabolism markers. Effect of SI tea on TG, LDL, and HDL in humans during a 60-day period. ▪: HG control; ♦: HG SI tea; □: HL control; ⋄: HL SI tea. Values are means ± SD. Differences in 30 day- or 60 day-values adjusted for variation in 0 day-values using the Tukey-Kramer multiple comparison post-tests. *P < 0.05, **P < 0.01.
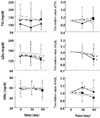
Table 3
Change of measured values in the subjects
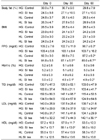
1)Values are mean ± SD.
2)Normal range: body fat (%): male, 17%-23%; female, 20%-27%; BMI: 18.5-< 25; FPG (mg/dl): <110 mg/dl; HbA1c (%): 4.4%-5.8%; TG (mg/dl): < 150 mg/dl; LDL (mg/dl): < 120 mg/dl; HDL (mg/dl): ≥ 40 mg/dl
3)P value is for treatment differences in 30 day- or 60 day-values adjusted for variation in 0 day-values using the Tukey-Kramer Multiple Comparison post tests. *P < 0.05, **P < 0.01.
References
1. Wild S, Roglic G, Green A, Sicree R, King H. Global prevalence of diabetes: estimates for the year 2000 and projections for 2030. Diabetes Care. 2004. 27:1047–1053.


2. Adachi M, Yamaoka K, Watanabe M, Nishikawa M, Hida E, Kobayashi I, Tango T. Effects of lifestyle education program for type 2 diabetes patients in clinics: study design of a cluster randomized trial. BMC Public Health. 2010. 10:742.


3. Hamdy O, Porramatikul S, Al-Ozairi E. Metabolic obesity: the paradox between visceral and subcutaneous fat. Curr Diabetes Rev. 2006. 2:367–373.


4. Wolf BW, Weisbrode SE. Safety evaluation of an extract from Salacia oblonga. Food Chem Toxicol. 2003. 41:867–874.


5. Yoshikawa M, Morikawa T, Matsuda H, Tanabe G, Muraoka O. Absolute stereostructure of potent alpha-glucosidase inhibitor, Salacinol, with unique thiosugar sulfonium sulfate inner salt structure from Salacia reticulata. Bioorg Med Chem. 2002. 10:1547–1554.


6. Ghavami A, Johnston BD, Pinto BM. A new class of glycosidase inhibitor: synthesis of salacinol and its stereoisomers. J Org Chem. 2001. 66:2312–2317.


7. Yoshikawa M, Murakami T, Yashiro K, Matsuda H. Kotalanol, a potent alpha-glucosidase inhibitor with thiosugar sulfonium sulfate structure, from antidiabetic ayurvedic medicine Salacia reticulata. Chem Pharm Bull (Tokyo). 1998. 46:1339–1340.


8. Williams JA, Choe YS, Noss MJ, Baumgartner CJ, Mustad VA. Extract of Salacia oblonga lowers acute glycemia in patients with type 2 diabetes. Am J Clin Nutr. 2007. 86:124–130.


9. Deguchi Y, Miyazaki K. Anti-hyperglycemic and anti-hyperlipidemic effects of guava leaf extract. Nutr Metab (Lond). 2010. 7:9.


10. Blonde L. Current antihyperglycemic treatment strategies for patients with type 2 diabetes mellitus. Cleve Clin J Med. 2009. 76:Suppl 5. S4–S11.
11. Akase T, Shimada T, Harasawa Y, Ikeya Y, Nagai E, Iizuka S, Nakagami G, Iizaka S, Sanada H, Aburada M. Preventive effects of Salacia reticulata on obesity and metabolic disorders in TSOD mice. Evid Based Complement Alternat Med. 2009. [Epub ahead of print].
12. Kishino E, Ito T, Fujita K, Kiuchi Y. A mixture of the Salacia reticulata (Kotala himbutu) aqueous extract and cyclodextrin reduces the accumulation of visceral fat mass in mice and rats with high-fat diet-induced obesity. J Nutr. 2006. 136:433–439.


13. Kohchi C, Inagawa H, Nishizawa T, Yamaguchi T, Nagai S, Soma G. Applications of lipopolysaccharide derived from Pantoea agglomerans (IP-PA1) for health care based on macrophage network theory. J Biosci Bioeng. 2006. 102:485–496.


14. Tsukioka D, Nishizawa T, Miyase T, Achiwa K, Suda T, Soma G, Mizuno D. Structural characterization of lipid A obtained from Pantoea agglomerans lipopolysaccharide. FEMS Microbiol Lett. 1997. 149:239–244.


15. Inagawa H, Nishizawa T, Tsukioka D, Suda T, Chiba Y, Okutomi T, Morikawa A, Soma GI, Mizuno D. Homeostasis as regulated by activated macrophage. II. LPS of plant origin other than wheat flour and their concomitant bacteria. Chem Pharm Bull (Tokyo). 1992. 40:994–997.


16. Braun-Fahrländer C, Riedler J, Herz U, Eder W, Waser M, Grize L, Maisch S, Carr D, Gerlach F, Bufe A, Lauener RP, Schierl R, Renz H, Nowak D, von Mutius E. Allergy and Endotoxin Study Team. Environmental exposure to endotoxin and its relation to asthma in school-age children. N Engl J Med. 2002. 347:869–877.


17. Cebra JJ. Influences of microbiota on intestinal immune system development. Am J Clin Nutr. 1999. 69:1046S–1051S.


18. Uribe A, Alam M, Midtvedt T, Smedfors B, Theodorsson E. Endogenous prostaglandins and microflora modulate DNA synthesis and neuroendocrine peptides in the rat gastrointestinal tract. Scand J Gastroenterol. 1997. 32:691–699.


19. Stappenbeck TS, Hooper LV, Gordon JI. Developmental regulation of intestinal angiogenesis by indigenous microbes via Paneth cells. Proc Natl Acad Sci U S A. 2002. 99:15451–15455.


20. Marshall JC. Lipopolysaccharide: an endotoxin or an exogenous hormone? Clin Infect Dis. 2005. 41:Suppl 7. S470–S480.


21. Miao Y, Zhou J, Chen C, Shen D, Song W, Feng Y. In vitro adsorption revealing an apparent strong interaction between endophyte Pantoea agglomerans YS19 and host rice. Curr Microbiol. 2008. 57:547–551.


22. Asis CA Jr, Adachi K. Isolation of endophytic diazotroph Pantoea agglomerans and nondiazotroph Enterobacter asburiae from sweetpotato stem in Japan. Lett Appl Microbiol. 2004. 38:19–23.


23. Pusey PL. Effect of nectar on microbial antagonists evaluated for use in control of fire blight of pome fruits. Phytopathology. 1999. 89:39–46.


24. Stockwell VO, Johnson KB, Sugar D, Loper JE. Antibiosis contributes to biological control of fire blight by Pantoea agglomerans strain Eh252 in orchards. Phytopathology. 2002. 92:1202–1209.


25. Iguchi M, Inagawa H, Nishizawa T, Okutomi T, Morikawa A, Soma GI, Mizuno D. Homeostasis as regulated by activated macrophage. V. Suppression of diabetes mellitus in non-obese diabetic mice by LPSw (a lipopolysaccharide from wheat flour). Chem Pharm Bull (Tokyo). 1992. 40:1004–1006.


26. Okutomi T, Nishizawa T, Inagawa H, Takano T, Morikawa A, Soma G, Mizuno D. Homeostasis as regulated by activated macrophage. VII. Suppression of serum cholesterol level by LPSw (a lipopolysaccharide from wheat flour) in WHHL (Watanabe heritable hyperlipidemic) rabbit. Chem Pharm Bull (Tokyo). 1992. 40:1268–1270.


27. Lu Q, Björkhem I, Wretlind B, Diczfalusy U, Henriksson P, Freyschuss A. Effect of ascorbic acid on microcirculation in patients with Type II diabetes: a randomized placebo-controlled cross-over study. Clin Sci (Lond). 2005. 108:507–513.


28. Earnest CP, Wood KA, Church TS. Complex multivitamin supplementation improves homocysteine and resistance to LDL-C oxidation. J Am Coll Nutr. 2003. 22:400–407.


29. Im R, Mano H, Nakatani S, Shimizu J, Wada M. Safety evaluation of the aqueous extract Kothala himbutu (Salacia reticulata) stem in the hepatic gene expression profile of normal mice using DNA microarrays. Biosci Biotechnol Biochem. 2008. 72:3075–3083.


30. Benalla W, Bellahcen S, Bnouham M. Antidiabetic medicinal plants as a source of alpha glucosidase inhibitors. Curr Diabetes Rev. 2010. 6:247–254.


31. Geutskens SB, Otonkoski T, Pulkkinen MA, Drexhage HA, Leenen PJ. Macrophages in the murine pancreas and their involvement in fetal endocrine development in vitro. J Leukoc Biol. 2005. 78:845–852.


32. Jun HS, Yoon CS, Zbytnuik L, van Rooijen N, Yoon JW. The role of macrophages in T cell-mediated autoimmune diabetes in nonobese diabetic mice. J Exp Med. 1999. 189:347–358.


33. Jansen A, Homo-Delarche F, Hooijkaas H, Leenen PJ, Dardenne M, Drexhage HA. Immunohistochemical characterization of monocytes-macrophages and dendritic cells involved in the initiation of the insulitis and beta-cell destruction in NOD mice. Diabetes. 1994. 43:667–675.


34. Meijer K, de Vries M, Al-Lahham S, Bruinenberg M, Weening D, Dijkstra M, Kloosterhuis N, van der Leij RJ, van der Want H, Kroesen BJ, Vonk R, Rezaee F. Human primary adipocytes exhibit immune cell function: adipocytes prime inflammation independent of macrophages. PLoS One. 2011. 6:e17154.


35. Wong FS, Visintin I, Wen L, Flavell RA, Janeway CA Jr. CD8 T cell clones from young nonobese diabetic (NOD) islets can transfer rapid onset of diabetes in NOD mice in the absence of CD4 cells. J Exp Med. 1996. 183:67–76.


36. Nishimura S, Manabe I, Nagasaki M, Eto K, Yamashita H, Ohsugi M, Otsu M, Hara K, Ueki K, Sugiura S, Yoshimura K, Kadowaki T, Nagai R. CD8+ effector T cells contribute to macrophage recruitment and adipose tissue inflammation in obesity. Nat Med. 2009. 15:914–920.


38. Mantovani A, Sozzani S, Locati M, Allavena P, Sica A. Macrophage polarization: tumor-associated macrophages as a paradigm for polarized M2 mononuclear phagocytes. Trends Immunol. 2002. 23:549–555.


39. Lumeng CN, Bodzin JL, Saltiel AR. Obesity induces a phenotypic switch in adipose tissue macrophage polarization. J Clin Invest. 2007. 117:175–184.


40. Fadok VA, Bratton DL, Konowal A, Freed PW, Westcott JY, Henson PM. Macrophages that have ingested apoptotic cells in vitro inhibit proinflammatory cytokine production through autocrine/paracrine mechanisms involving TGF-beta, PGE2, and PAF. J Clin Invest. 1998. 101:890–898.


41. Porta C, Rimoldi M, Raes G, Brys L, Ghezzi P, Di Liberto D, Dieli F, Ghisletti S, Natoli G, De Baetselier P, Mantovani A, Sica A. Tolerance and M2 (alternative) macrophage polarization are related processes orchestrated by p50 nuclear factor kappaB. Proc Natl Acad Sci U S A. 2009. 106:14978–14983.


42. Mabley JG, Pacher P, Southan GJ, Salzman AL, Szabó C. Nicotine reduces the incidence of type I diabetes in mice. J Pharmacol Exp Ther. 2002. 300:876–881.


43. Petersen AM, Pedersen BK. The anti-inflammatory effect of exercise. J Appl Physiol. 2005. 98:1154–1162.


44. Schenk S, Saberi M, Olefsky JM. Insulin sensitivity: modulation by nutrients and inflammation. J Clin Invest. 2008. 118:2992–3002.


45. Fukushima M, Usami M, Ikeda M, Nakai Y, Taniguchi A, Matsuura T, Suzuki H, Kurose T, Yamada Y, Seino Y. Insulin secretion and insulin sensitivity at different stages of glucose tolerance: a cross-sectional study of Japanese type 2 diabetes. Metabolism. 2004. 53:831–835.


46. Xu L, Jiang CQ, Lam TH, Cheng KK, Yue XJ, Lin JM, Zhang WS, Thomas GN. Impact of impaired fasting glucose and impaired glucose tolerance on arterial stiffness in an older Chinese population: the Guangzhou Biobank Cohort Study-CVD. Metabolism. 2010. 59:367–372.


47. Lin JD, Wan HL, Li JC, Wu CZ, Kuo SW, Hsieh CH, Lian WC, Lee CH, Kao MT, Pei D. Impaired glucose tolerance and impaired fasting glucose share similar underlying pathophysiologies. Tohoku J Exp Med. 2007. 212:349–357.


48. Payne WR, Walsh KJ, Harvey JT, Livy MF, McKenzie KJ, Donaldson A, Atkinson MG, Keogh JB, Moss RS, Dunstan DW, Hubbard WA. Effect of a low-resource-intensive lifestyle modification program incorporating gymnasium-based and home-based resistance training on type 2 diabetes risk in Australian adults. Diabetes Care. 2008. 31:2244–2250.

