Abstract
The aim of the present study was to investigate the hypocholesterolemic effect and potential of tyramine derivatives from Lycii Cortex Radicis (LCR), the root bark of lycium (Lycium chenese Miller) in reducing lipid peroxidation. The activities of enzymes, hepatic 3-hydroxy 3-methylglutaryl (HMG) CoA reductase and acyl-CoA:cholesterol acyltransferase (ACAT) and LDL oxidation were measured in vitro and animal experiments were also performed by feeding LCR extracts to rats. The test compounds employed for in vitro study were trans-N-p-coumaroyltyramine (CT) and trans-N-feruloyltyramine (FT), LCR components, N-(p-coumaroyl)serotonin (CS) and N-feruloylserotonin (FS) from safflower seeds, ferulic acid (FA) and 10-gingerol. It was observed that FT and FS at the concentration of 1.2 mg/mL inhibited liver microsomal HMG CoA reductase activity by ~40%, but no inhibition of activity was seen in the cases of CT, CS, FA and 10-gingerol. Whereas, ACAT activity was inhibited ~50% by FT and CT, 34-43% by FS and CS and ~80% by 10-gingerol at the concentration of 1 mg/mL. A significant delay in LDL oxidation was induced by CT, FT, and 10-gingerol. For the animal experiment, five groups of Sprague-Dawley male rats were fed high fat diets containing no test material (HF-control), 1 and 2% of LCR ethanol extract (LCR1 and LCR2), and 1% of extracts from safflower seed (Saf) and ginger (Gin). The results indicated that total cholesterol level was significantly lower in Saf, LCR2 and Gin groups, and HDL cholesterol level was lower only in Gin group when compared with HF-control group; while there was no difference in the serum triglyceride levels among the five experimental groups. The level of liver cholesterol was significantly lower in LCR1 and LCR2 groups than HF-control. Serum levels of TBARS were significantly lower only in LCR2 group when compared with HF-control group. From the observed results, we concluded that LCR can be utilized as a hypocholesterolemic ingredient in combination with ginger, especially for functional foods.
Atherosclerosis is a multi-factorial disease. Hypercholesterolemia and oxidation of plasma low density lipoprotein (LDL) are known to be the main factors for causing atherogenesis. Sixty to seventy percent of the body cholesterol has been known to be of endogenous origin. The most important step in endogenous cholesterol synthesis is the reaction mediated by a rate-limiting enzyme, 3-hydroxy 3-methylglutaryl (HMG) CoA reductase. A number of commercially available, serum cholesterol lowering agents had been developed on the basis of inhibition of HMG CoA reductase activity [1-4]. Since the development of such agents, many other derivatives have been synthesized and prescribed to hyperlipidemic patients. As most of the aforementioned drugs are imported from foreign pharmaceutical companies and are therefore expensive, they cause significant economic burden on individuals as well as on the whole nation. In addition, many hyperlipidemic subjects are reluctant to use pills, and practitioners look for some other options rather than medicines to offer to the public, who just need an advice. Several vegetable food substances, like tocotrienol in barley [5], various organosulfur compounds in garlic [6], bioflavonoids [7,8], and extracts of sorghum and prosmillet [9] have been reported to inhibit HMG CoA reductase activity. Furthermore, HMG CoA reductase inhibitory activity has also been identified in polyphenols from safflower seed [10], soybean peptide [11], and several plants [12,13]. Many patients who received HMG CoA reductase inhibitors, however, did not achieve the desired cardiovascular benefits. Accordingly, other mechanisms via which cholesterol metabolism is regulated have drawn great attention, amongst which acyl-CoA:cholesterol acyltransferase (ACAT) activity has been known to be a typical one. ACAT, a cholesterol-esterifying enzyme in tissues plays key roles in intracellular cholesterol storage, lipoprotein assembly in the liver, and the development of fatty streaks in arteries. Accumulation of cholesteryl esters within macrophages constitutes the hallmark of foam cells during atherogenesis. Therefore, considerable interest has been emerged in developing ACAT inhibitors. In Korea, several natural substances [14], flavonoids isolated from the roots of Glycine max (L.) Merr. [15], and dammarane triterpenes from Rhus chinensis [16] have been identified as materials, which inhibit ACAT activity. Internationally, pactimibe sulfate (CS-505;[7-(2,2-dimethylpropanamido)-4,6-dimethyl-1-octylindolin-5-yl]acetic acid), a well characterized compound which had been developed as a synthetic ACAT inhibitor, exhibited positive results in studies where ACAT activity inhibition and anti-atherosclerotic potency of macrophages were investigated by employing animal models [17-19]. Although no effect was observed in human study unlike in animal ones [20], the effect of ACAT inhibitor on prevention of hyperlipidemia still remains to be investigated. As transformation of macrophages into foam cells due to serum LDL oxidation and vascular plaque formation accelerates atherosclerosis [21], it is very important to identify materials suppressing LDL oxidation. Antioxidants like, vitamins E and C [22], soybean isoflavone [23], and vegetable polyphenols obtained from safflower seeds [10] were reported to be major materials inhibiting LDL oxidation. In addition, various substances are routinely being explored as inhibitors of LDL oxidation.
This study was conducted to investigate the anti-hyperlipidemic effect of Lycii Cortex Radicis (LCR), the dried root bark of lycium, which is cultivated in Korea. Lycium (Lycium chenese Miller), which belongs to the solanacea family, has been extensively used in traditional Chinese herbal medicine as an antipyretic agent and to treat pneumonia, night-sweats, cough, hematemesis, inflammation, and diabetic mellitus. Hypoglycemic effect of lycium was confirmed in alloxan-induced diabetic mice, and a decrease in blood cholesterol and triglyceride level was also observed [24]. LCR has been reported to inhibit skin damage caused by UV [25] and hepatic damage caused by CCl4 [26], and proposed to possess antioxidant activity; but no reports are available on the substances responsible for the antioxidant potential. However, a group of phenolic amide were isolated from the LCR and shown to have anti-fungal effects [27]. Amongst them are trans-N-p-coumaroyltyramine (CT) and trans-N-feruloyltyramine (FT). As can be seen in Fig. 1, their structures are similar to the structures of N-(p-coumaroyl)serotonin (CS) and N-feruloylserotonin (FS), the serotonin derivatives in safflower seeds. As HMG CoA reductase activity has been reported to be inhibited by the serotonin derivatives [10], it is also necessary to examine the effect of the LCR phenolic amides. Hence, in our present study, the inhibitory effects of CT, and FT, the LCR phenolic amides on HMG CoA reductase activity were investigated. In addition, the inhibitory effects of CT and FT on ACAT activity and LDL oxidation were also examined. Furthermore, the effect of gingerols, important components of ginger, was also investigated to explore its utilization in association with LCR. Based on the results of the in vitro studies, an in vivo study was conducted to investigate the effects of high fat diets with ethanol extracts of LCR, safflower seed, and ginger on serum and liver lipid levels and lipid peroxidation in rats.
The enzymes, NADPH, HMG CoA, mevalonic acid, and oleoyl CoA used in HMG CoA reductase and ACAT assay were purchased from Sigma-Aldrich (St. Louis, USA), and HMG CoA 14C and Oleoyl-CoA 14C were purchased from PerkinElmer (NEN) (Boston, USA). Ferulic acid, coumaric acid and 10-gingerol were purchased from Sigma-Aldrich (St. Louis, USA), and simvastatin was from MSD Korea (Zokor). Mineral mix, vitamin mix casein, cornstarch, and cellulose were purchased from Central Lab Animal Inc. (Seoul, Korea). Sucrose, soybean oil and lard were purchased from a local market. All other chemicals used in this study were of analytical grade.
The serotonin derivatives, CS and FS were isolated from safflower seeds by following a method developed by Kang et al. [28]. The phenolic amides CT and FT, were prepared via a method developed by Rajan et al. [29] instead of carrying out isolation from LCR. The two tyramine derivatives were synthe-sized by using either coumaric acid or ferulic acid and tyramine hydrochloride as reactants and N-(3-dimethylaminopropyl)-N-ethylcarbodiimide hydrochloride as a coupling reagent. The purification of the derivatives was carried out by silica gel column chromatography (CHCl3-MeOH = 8:1, v/v), followed by re-crystallization. The synthetic tyramines were confirmed to be identical with the ones purified from LCR by HPLC analysis coupled with UV detection at 310 nm [30]. The synthetic tyramines were used in in vitro experiments, because it was more convenient to prepare small amounts in pure forms based on requirement of the experiment. For animal experiments, 80% ethanol extracts of defatted safflower seed containing CS and FS, LCR containing CT and FT, and ginger containing gingerols were used as test materials. The extracts were prepared from dry powder of safflower seed, LCR and ginger. Hundred grams of individual powder was extracted twice with 1 L of 80% aqueous EtOH for 2 hr, filtered and evaporated under reduced pressure. Each extract was variously diluted and passed through Sep-Pak C18 cartridges (Waters, USA) and injected into HPLC [Waters 2695 Alliance HPLC system coupled with Water 2998 photodiode array detector (Waters, Milford, MA, USA)]. Based on the calibration curves of standard compounds, CS, FS, and gingerol were quantified at 280 nm by using a linear gradient elution from 0.05% H3PO4 to 100% MeOH. Similarly, CT and FT were quantified at 310 nm by using another linear gradient from 0.05% trifluoroacetic acid to 100% acetonitrile [30]. Contents of each of the compounds in the extracts were identified as, CS, 3.4 wt%, FS, 5.0 wt%, CT, 0.39 wt%, FT, 0.29 wt%, and gingerols, 6.3 wt%.
Male Sprague-Dawley rats weighing 150 ± 10 g were fed with a commercial non-purified diet (to which 2% cholestyramine was added) for 10 days. After the completion of feeding, the rats were anesthetized with ether at 8 pm, and the microsome was isolated from liver tissue and used as a source of HMG CoA reductase and ACAT [31]. HMG CoA reductase assay by using 14-C HMG CoA was carried out as described previously [10]. Typically, 2 µl of test materials (5-20 mg/mL DMSO) were added to a total 33 µl of the enzyme reaction mixture. ACAT assay by using [14-C]oleoyl-CoA was carried out according to the method of Lee and Choi [12]. About 10 µl of test materials (5-20 mg/mL DMSO) were added to a total 200 µl of ACAT reaction mixture.
LDL was prepared from human serum and LDL oxidation was induced by CuSO4, and measured according to the method of Hirano et al. [32]. LDL oxidation was initiated by the addition of 5 µM Cu2SO4 at a final concentration in 0.8 ml of LDL solution (~100 µg protein/mL PBS, pH 7.4). To examine the anti-oxidative effects of the test compounds, 4 µl of the test materials were added to the LDL solution such that the final concentration was 2.5 µg/mL. The kinetics of the LDL oxidation were determined spectrophotometrically by monitoring the change in conjugate diene formation at 234 nm by Thermo Model 9423AQA2200E spectrophotometer equipped with 7-positioned automatic sample changer. The absorbance curve at 234 nm was divided into three phases; lag time, propagation phase and decomposition phase. The lag time was measured as the interval between the intercept of tangent of the slope of the curve in the propagation phase with the base line and was expressed in minutes as defined by Hirano et al. [32].
Sprague-Dawley male rats weighing 150 ± 10 g obtained from Orient Bio Inc. (Kyunggido, Korea) were housed in a room with controlled temperature (20-22 degrees C), humidity (55-65%), and lighting (8 am-8 pm). After acclimatization for one week with a commercial non-purified purina chow, the rats were randomly divided into five groups of eight animals each and fed with high fat experimental diets [33,34]. Table 1 shows the details of five experimental diets according to AIN-93G [35] except for the modification in the carbohydrate contents. One group of rats (HF-control) was fed the high fat diet with no test material and two groups of rats were fed with the experimental diets to which 1% of the 80% ethanol extract of defatted safflower seed (Saf), and the ginger extract (Gin) were added. Whilst, another two groups of rats were fed diets containing 1 and 2% of the ethanol extract of root bark of lycium (LCR1 and LCR2), respectively. The amount of the dried extracts added to the diets was determined in accordance with a previous study [36]. As described above, the amount of CT and FT, active components in the LCR extract was as low as about 10% of CS and FS in safflower seed or gingerols in ginger extracts. Therefore, a diet containing 2% of the LCR extract was prepared for the LCR2 group in addition to LCR1. Inclusion of higher than 2% of the LCR extract in the experimental diet was considered as undesirable due to probable imbalances in the contents of macronutrients amongst the test diets. The rats were on experimental diets for 4 weeks and body weight was measured during the feeding period, after which they were sacrificed to conduct biochemical analysis. This study was approved by Animal Use Committee of Catholic University of Daegu and maintained in accordance with the guidelines of Catholic University of Daegu on the care and use of laboratory animals.
Total cholesterol, HDL-cholesterol, and triglyceride in serum obtained from the rats were quantified by using an enzyme kit (Asan Pharmaceutical, Seoul). Liver cholesterol and triglyceride were measured by using the same enzymatic kit and by the aid of a detergent, triton X-100, as described before [36]. Serum thiobarbituric acid reactive substances (TBARS), and index of lipid peroxidation, were measured according to a method developed by Yagi [37], which employed 1,1,3,3-tetraethoxy-propane as a reference material. Glutamate oxaloacetate transaminase (GOT) and glutamate pyruvate transaminase (GPT) activities, which are the indices of hepatic damage, and blood urea nitrogen (BUN), an index of protein metabolism disorder, were measured by using an enzyme kit (Asan Pharmaceutical, Seoul).
The effects of CT and FT, LCR tyramines, on HMG CoA reductase and ACAT activities are presented in Fig. 2 (A, B) along with the effects of CS and FS, serotonin derivatives from safflower seed, and FA, and 10-gingerol. The concentrations of the test materials in the reaction mixtures of HMG CoA reductase and ACAT were 1.2 and 1 mg/mL, respectively. As shown in Fig. 1A, there was no change in the HMG CoA reductase activity (296.5 ± 39.8 pmole NADPH/min/mg protein) with no test material (none), despite the addition of CS or CT. However, when FS and FT were added, the enzyme activity was decreased to 186.4 ± 7.2 and 188.1 ± 10.2 pmole NADPH/min/mg protein, respectively, showing the activity inhibition rate as ~40%. On the hand, neither FA nor 10-gingerol had any inhibitory effect on HMG CoA reductase. Conversely, all the test materials used in this study, except FA had an inhibitory effect on ACAT activity (Fig. 1B). CS and FS decreased the ACAT activity by 34-43% when compared to the activity (981.2 ± 56.4 pmol/min/mg protein) in the absence of the test materials (none). Amongst all the LCR tyramines, FT was more effective in inhibiting ACAT activity (~50%) than CT. Among all the test materials, 10-gingerol was the most effective in inhibiting ACAT activity by 80%. To examine the inhibitory actions of FS and FT at lower concentrations, the concentrations were reduced to 0.3 and 0.25 mg/mL from 1.2 and 1 mg/mL in the reaction mixture of HMG CoA reductase and ACAT, respectively and the results are shown in Table 2. The inhibitory action of FS on HMG CoA reductase decreased from 49 to 34% and from 40 to 19% in the case of ACAT. Similarly, at FT's concentration of 0.6 mg/mL, there was a decrease in HMG CoA reductase activity from 56 to 36% and from 49 to 16% in the case of ACAT. FS appeared to be more effective inhibitor of HMG CoA reductase activity than FT at low concentration, but both the compounds had the same efficacy in inhibiting ACAT activity. When FS and FT were compared with simvastatin, a well-known inhibitor of HMG CoA reductase, their efficacy was revealed to be 1/40th of simvastatin.
The inhibition of LDL oxidation by the test materials was shown as increase in lag time required for initiation of LDL oxidation (Fig. 3). LDL oxidation was significantly delayed by CT, FT, and 10-gingerol, while no significant change was observed in the presence of CS, FS and FA.
Initial and final body weights, body weight gains, food intakes, and food efficiency ratios of the five rat groups fed with experimental diets (Table 1) for 4 weeks are presented in Table 3. The average initial body weight of rats was shown to be 209 ± 10 g prior to the intake of experimental diets and there was no difference among the five groups, but there was a major difference in the final body weights of the groups. The final weights of the groups were in the descending order as HF-control, Saf, LCR1, LCR2 and Gin groups, the latter two of which had significantly lower body weights than HF-control and Saf group. This observation corresponded with the body weight gains and food intakes. However, amongst the five experimental groups, food efficiency ratio was significantly lower only in Gin group. Table 4 shows weights of body fat pad in various parts of the animal body. Subscapular fat weight was lower in Gin group, whereas epididymal fat weights were significantly lower in LCR2 and Gin groups than HF-control group. Total fat weights were also lower in LCR2 and Gin groups than HF-control, Saf and LCR1 groups.
Fig. 4 shows serum levels of total cholesterol, HDL-cholesterol and triglyceride in the five groups of rats fed with the experimental diets. Total cholesterol level was significantly lower in Saf, LCR2 and Gin groups, and HDL cholesterol level was lower only in Gin group than in HF-control group, while there was no difference in the serum triglyceride levels among the five experimental groups, although it appeared to be lower in Gin group. The HDL-cholesterol/total cholesterol ratios (%) were in range from 63.3 ± 3.4 in HF-control group to 70.2 ± 2.9 in Saf group, but no significant difference was observed amongst the five groups.
Fig. 5 shows levels of liver cholesterol and triglyceride of the five experimental groups of rats. The level of liver cholesterol was significantly lower in LCR1 and LCR2 groups but not in Saf and Gin groups, when compared with HF-control. On the other hand, the level of liver triglyceride was lower in the four test groups than HF-control.
Serum levels of TBARS were significantly lower only in LCR2 group than in HF-control group, and the results are shown in Fig. 6. Serum GOT and GPT levels, hepatic damage indices, BUN, index of protein metabolism disorder, are presented in Table 5. When compared to HF-control group, no change in the values was observed in Saf, LCR1, LCR2, and Gin groups.
The present study revealed that the extract of Lycii Cortex Radicis (LCR), as well as safflower seed and ginger extracts, inhibited in vitro activities of HMG-CoA reductase and ACAT and also exhibited its effects on reduction of serum total cholesterol in vivo. In particular, FT, a LCR phenolic amide, inhibited in vitro enzyme activities as effectively as FS from safflower seed. Furthermore, it was interesting to note that the LCR extract containing the phenolic amides at much lower concentrations than serotonins in safflower seed extract, reduced in vivo serum cholesterol in a manner similar to safflower seed extract. On the basis of contents of CS, FS, CT, FT and gingerols of each extract, LCR1 and LCR2 diets contained about 0.07 g and 0.14 g of LCR tyramines per kg while Saf and Gin diets contained 0.84 g of serotonin derivatives and 0.63 g/kg of gingerols, respectively. Since FT, LCR tyramine did not have extensive effect on in vitro inhibition of HMG CoA reductase as FS at low concentrations (0.5 and 1.0 mg/mL), the presence of other substances in the bulk of the LCR extract might have contributed to hypocholesterolemic effect. Major substances present in the LCR extract are regarded to be carbohydrates, including minor amount of fiber and negligible amount of protein. The carbohydrates in the LCR extract are likely to be comprised of oligosaccharides, since plant roots such as burdock [38] and chicory [39] have been reported to contain inulin-type oligosaccharide. The oligosaccharides derived from plants had been reported to lower serum cholesterol levels [40,41] and dietary fructooligosaccharide were reported to reduce serum triglyceride levels in rats [42]. Therefore, the hypocholesterolemic effect shown by the LCR extract could partly be due to the presence of oligosaccharides and/or other substances, which remains to be investigated in the future; while the effect can be explained on the basis of direct inhibitory action of the FT on HMG CoA reductase to a certain degree. In the present study, it was also notable that the serum cholesterol level was reduced in vivo by ginger extract containing gingerols, including 10-gingerol that was shown as an effective inhibitor of ACAT but not of HMG-CoA reductase. Only fewer reports are available on the simultaneous studies conducted both, in vitro and in vivo on inhibitory effects of materials from plants in conjunction with hyperlipidemia on enzymes, except for certain drugs with similar activity [2-4]. In our previous study [10], FS was reported to inhibit HMG CoA reductase activity but the effects of CS were not investigated. In this study, neither CS nor CT inhibited the enzyme activity. Therefore, it was inferred that feruloyl group was essential for the inhibition of the enzyme activity. But, the inhibitory action apparently seemed to require complex structures such as FS and FT, as ferulic acid alone did not inhibit the enzyme activity. Simvastatin (structure shown in Fig. 1) has been known to be a strong competitive inhibitor of HMG CoA reductase, because of the presence of 6-oxotetrahydro-2H-pyran-2-yl (marked part) group in the structure that binds to the active site of the enzyme. The action mechanisms of FS and FT in inhibiting HMG CoA reductase activity are yet to be resolved. Previous in vivo [7,8,43] and in vitro [5,9-13] studies have reported on inhibitions of HMG CoA reductase activity by various plant substances and extracts. However, in vitro inhibitory effect of FT from LCR on HMG CoA reductase was first reported in this study. Ferulic acid, which did not show an in vitro inhibitory effect on HMG CoA reductase, was reported to decrease the enzyme activity in vivo when it was offered to Apo-E deficient mice [44] or hyperlipidemic diabetic rats [45].
It was very significant to note that phenolic amides from LCR and serotonin derivatives from safflower seed showed an inhibitory effect on ACAT as well as HMG CoA reductase activity, which possibly helps to prevent hypercholesterolemia. Gingerols were more frequently reported to show anti-cancer effect [46,47] than hypolipidemic one, as it possess antioxidant and anti-inflammatory activities [48]. In this study, 10-gingerol was shown to have a high inhibitory effect on ACAT activity. Kim et al. [14] have reported that LCR and ginger extracts inhibited in vitro ACAT activity by 8 and 30%, respectively when they examined the alcohol extracts from 303 plants. Based on the results, we inferred that FT and 10-gingerol are the main active components for the inhibitory action, as also reported by Kim et al. [14]. Decreased serum and liver cholesterol level by diet containing ginger extract seems to be associated with the potent inhibitory effect of gingerol on the ACAT activity. From the structural comparison between the test compounds used for in vitro enzyme assay in this study and pactimibe, a well-known ACAT activity inhibitor (Fig. 1), the nature of mechanisms involved in the ACAT inhibition by the compounds is not clear and further studies are needed to elucidate the underlying mechanisms.
Lower body weight gains and less body fat amounts in LCR2 and Gin when compared to other groups could be accounted due to less food intake, possibly because of bitter or repulsive taste of the diets. Although no difference in food efficiency was found between the control (HF-control) group and the two LCR groups, there was a decrease in the relative body fat amount in the LCR2 group. It seems necessary to use extracts with less repulsive taste or to do isocaloric pair-fed study in future animal experiments. To the best of our knowledge, our report is the first one to demonstrate anti-oxidative effect of LCR tyramine, FT in terms of delayed LDL oxidation, although other studies have suggested the possibility of using LCR extract for the similar purpose [25,26]. The in vitro effect was well matched with reduction of serum TBARS in vivo in LCR2 groups. As described above, the tyramine contents of LCR2 diet were much less than the other active substances in Saf or Gin diet. To perceive more confirmative effects of the LCR tyramines, it is suggested to increase its content in the diet. This can be accomplished by using more purified extract or synthesized compounds [29]. An appropriate method could be chosen with due consideration about the economic aspect. Alternatively, various mixtures of LCR and ginger extracts could be used in diet to discover best efficacy and the strategy can be applicable for the production of functional foods.
Figures and Tables
Fig. 1
Structures of serotonin derivatives (a,b), ferulic acid (c), tyramine derivatives (d,e), simvastatin (f), gingerol (g), and pactimibe (h). a, N-(p-coumaroyl)serotonin (CS); b, N-feruloylserotonin (FS); c, ferulic acid; d, trans-N-p-coumaroyltyramine (CT); e, trans-N-feruloyltyramine (FT); f, simvastatin; g, gingerol; h, pactimbe.
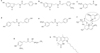
Fig. 2
Effects of serotonin derivatives (CS, FS) from safflower seed and tyramine derivatives (CT, FT) from root bark of Lycium chenese Miller, ferulic acid (FA) and 10-gingerol on HMG-CoA reductase (A) and ACAT (B) activities. CS, N-(p-cumaroyl)serotonin; FS, N-feruloylserotonin; CT, trans-N-coumaroyltyramine; FT, trans-N-feruloyltyramine; FA, ferulic acid at the concentrations of 1.2 mg/mL for HMG CoA reducatse and of 1 mg/mL for ACAT reactions. Values are means ± SD of three replicates and those with * and ** are significantly different from none at P < 0.05 and < 0.01, respectively.
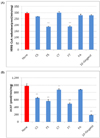
Fig. 3
In vitro effects of serotonin derivatives (CS, FS) from safflower seed and tyramine derivatives (CT, FT) from root bark of Lycium chenese Miller, ferulic acid (FA) and 10-gingerol on LDL oxidation. CS, N-(p-cumaroyl)serotonin; FS, N-feruloylserotonin; CT, trans-N-coumaroyltyramine; FT, trans-N-feruloyltyramine; FA, ferulic acid at the concentration of 2.5 µg/mL. Values are means ± SD of three replicates and those with * and ** are significantly different from none at P < 0.05 and < 0.01, respectively.
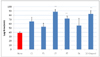
Fig. 4
Effects on serum lipid levels in five experimental groups1) of rats fed with high fat diets for four weeks. 1)Groups are same as described in Table 1. Bars are means ± SE from eight rats and those with different alphabet letters are at P < 0.05.
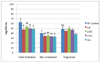
Fig. 5
Effects on liver lipid levels in five experimental groups1) of rats fed with high fat diets for four weeks. 1)Groups are same as described in Table 1. Bars are means ± SE from eight rats and those with different alphabet letters are at P < 0.05.
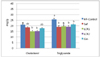
Fig. 6
Effects on serum TBARS levels in five experimental groups1) of rats fed with high fat diets for four weeks. 1)Groups are same as described in Table 1. Bars are means ± SE from eight rats and those with different alphabet letters are at P < 0.05.
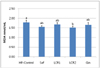
Table 2
Effects of various concentration of N-feruloylserotonin and trans-N-feruloyltyramine on activities of HMG CoA reductase and ACAT
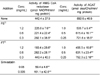
Table 3
Body weights, food intakes and food efficiency ratios of five experimental groups of rats fed with high fat diets for four weeks

1)Groups are same as described in Table 1.
2)Values are means ± SD from eight rats and those with different alphabet letters in the same column are significantly different at P < 0.05.
NS, not significant
Table 4
Fat pad weights at various regions in five experimental groups of rats fed with high fat diets for four weeks

1)Groups are same as described in Table 1.
2)Values are means ± SD from eight rats and those with different alphabet letters in the same column are significantly different at P < 0.05.
NS, not significant
Table 5
Serum GOT, GPT and BUN levels in five experimental groups
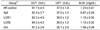
1)Groups are same as described in Table 1. Values are means ± SE from eight rats.
References
1. Endo A. Monacolin K, a new hypocholesterolemic agent that specifically inhibits 3-hydroxy-3-methylglutaryl coenzyme A reductase. J Antibiot (Tokyo). 1980. 33:334–336.


2. Endo A, Komagata D, Shimada H. Monacolin M, a new inhibitor of cholesterol biosynthesis. J Antibiot (Tokyo). 1986. 39:1670–1673.


3. Gunde-Cimerman N, Plemenitas A, Cimerman A. A hydroxymethylglutaryl-CoA reductase inhibitor synthesized by yeasts. FEMS Microbiol Lett. 1995. 132:39–43.


4. Grundy SM. HMG-CoA reductase inhibitors for treatment of hypercholesterolemia. N Engl J Med. 1988. 319:24–33.


5. Qureshi AA, Burger WC, Peterson DM, Elson CE. The structure of an inhibitor of cholesterol biosynthesis isolated from barley. J Biol Chem. 1986. 261:10544–10550.


6. Gebhardt R, Beck H. Differential inhibitory effects of garlic-derived organosulfur compounds on cholesterol biosynthesis in primary rat hepatocyte cultures. Lipids. 1996. 31:1269–1276.


7. Bok SH, Lee SH, Park YB, Bae KH, Son KH, Jeong TS, Choi MS. Plasma and hepatic cholesterol and hepatic activities of 3-hydroxy-3-methyl-glutaryl-CoA reductase and acyl CoA: cholesterol transferase are lower in rats fed citrus peel extract or a mixture of citrus bioflavonoids. J Nutr. 1999. 129:1182–1185.


8. Bok SH, Park SY, Park YB, Lee MK, Jeon SM, Jeong TS, Choi MS. Quercetin dihydrate and gallate supplements lower plasma and hepatic lipids and change activities of hepatic antioxidant enzymes in high cholesterol-fed rats. Int J Vitam Nutr Res. 2002. 72:161–169.


9. Ha TY, Cho IJ, Lee SH. Screening of HMG-CoA reductase inhibitory activity of ethanol and methanol extracts from cereals and regumes. Korean J Food Sci Technol. 1998. 30:224–229.
10. Cho SH, Park YY, Yoon JY, Choi SW, Ha TY. The effect of polyphenols from safflower seed on HMG-CoA reductase (HMGR) activity, LDL oxidation and apo A1 secretion. Korean J Food Sci Technol. 2006. 38:279–283.
11. Pak VV, Koo M, Lee N, Oh SK, Kim MS, Lee JS, Kwon DY. Hypocholesterolemic soybean peptide (IAVP) inhibits HMG-CoA reductase in a competitive manner. Food Sci Biotechnol. 2005. 14:727–731.
12. Lee HJ, Choi MS. Measurement of inhibitory activities on 3-hydroxy-3-methylglutaryl CoA reductase and acyl-CoA:cholesterol acyltransferase by various plant extracts in vitro. J Korean Soc Food Sci Nutr. 1999. 28:958–962.
13. Kim NJ, Jung EA, Kim DH, Lee SI. Studies on the development of antihyperlipidemic drugs from oriental herbal medicines (I)): antihyperlipidemic activities of oriental herbal medicines. Korean J Pharmacogn. 1999. 30:368–376.
14. Kim MK, Kwon BM, Bae KH, Choi DH, Lee HJ, Kim HE, Kim YK. Screening of Acyl-CoA:cholesterol acyltransferase (ACAT) inhibitors from natural sources. Korean J Pharmacogn. 1999. 30:384–396.
15. Lee JH, Seo WD, Jeong SH, Jeong TS, Lee WS, Park KH. Human acyl-CoA: cholesterol acyltransferase inhibitory effect of flavonoids from roots of Glycine max (L.) Merr. J Korean Soc Appl Biol Chem. 2006. 49:57–61.
16. Kim GS, Jeong TS, Kim YO, Baek NI, Cha SW, Lee JW, Song KS. Human acyl-CoA:cholesterol acyltransferase-inhibiting dammarane triterpenes from Rhus chinensis. J Korean Soc Appl Biol Chem. 2010. 53:417–421.


17. Kitayama K, Tanimoto T, Koga T, Terasaka N, Fujioka T, Inaba T. Importance of acyl-coenzyme A:cholesterol acyltransferase 1/2 dual inhibition for anti-atherosclerotic potency of pactimibe. Eur J Pharmacol. 2006. 540:121–130.


18. Terasaka N, Miyazaki A, Kasanuki N, Ito K, Ubukata N, Koieyama T, Kitayama K, Tanimoto T, Maeda N, Inaba T. ACAT inhibitor pactimibe sulfate (CS-505) reduces and stabilizes atherosclerotic lesions by cholesterol-lowering and direct effects in apolipoprotein E-deficient mice. Atherosclerosis. 2007. 190:239–247.


19. Ikenoya M, Yoshinaka Y, Kobayashi H, Kawamine K, Shibuya K, Sato F, Sawanobori K, Watanabe T, Miyazaki A. A selective ACAT-1 inhibitor, K-604, suppresses fatty streak lesions in fat-fed hamsters without affecting plasma cholesterol levels. Atherosclerosis. 2007. 191:290–297.


20. Nissen SE, Tuzcu EM, Brewer HB, Sipahi I, Nicholls SJ, Ganz P, Schoenhagen P, Waters DD, Pepine CJ, Crowe TD, Davidson MH, Deanfield JE, Wisniewski LM, Hanyok JJ, Kassalow LM. ACAT Intravascular Atherosclerosis Treatment Evaluation (ACTIVATE) Investigators. Effect of ACAT inhibition on the progression of coronary atherosclerosis. N Engl J Med. 2006. 354:1253–1263.


21. Witzum JL. Leaf A, Weber PC, editors. The role of monocytes and oxidized LDL in atherosclerosis. Atherosclerosis Reviews. 1990. Vol. 21. New York: Raven Press;59–69.
22. Dieber-Rotheneder M, Puhl H, Waeg G, Striegl G, Esterbauer H. Effect of oral supplementation with D-α-tocopherol on the vitamin E content of human low density lipoproteins and resistance to oxidation. J Lipid Res. 1991. 32:1325–1332.


23. Meng QH, Lewis P, Wähälä K, Adlercreutz H, Tikkanen MJ. Incorporation of esterified soybean isoflavones with antioxidant activity into low density lipoprotein. Biochim Biophys Acta. 1999. 1438:369–376.


24. Gao D, Li Q, Liu Z, Li Y, Liu Z, Fan Y, Li K, Han Z, Li J. Hypoglycemic effects and mechanisms of action of Cortex Lycii Radicis on alloxan-induced diabetic mice. Yakugaku Zasshi. 2007. 127:1715–1721.


25. Ahn BY, Gwak JS, Ryu SH, Moon GS, Choi DS, Park SH, Han JH. Protective effect of water extract of Lycii Cordex Radicis on lipid peroxidation of rat skin exposed to ultraviolet B radiation. Agric Chem Biotechnol. 2002. 45:218–222.
26. Cho YJ, Kim SH. Protective effect of EA fraction of Lycii Cortex Radix on the hepatic damage in mice induced by CCl4. Korean J Orient Med Pathol. 1997. 11:63–71.
27. Lee DG, Park Y, Kim MR, Jung HJ, Seu YB, Hahm KS, Woo ER. Anti-fungal effects of phenolic amides isolated from the root bark of Lycium chenense. Biotechnol Lett. 2004. 26:1125–1130.


28. Kang GH, Chang EJ, Choi SW. Antioxidative activity of phenolic compounds in roasted safflower (Carthamus tinctorius L.) seeds. J Korean Soc Food Sci Nutr. 1999. 4:221–225.
29. Rajan P, Vedernikova I, Cos P, Berghe DV, Augustyns K, Haemers A. Synthesis and evaluation of caffeic acid amides as antioxidants. Bioorg Med Chem Lett. 2001. 11:215–217.


30. Kim EO, Son WR, Kwon SO, Choi SW. Antilipidemic activity of phenolic compounds isolated from plant sources. Abstracts from Annual Meeting of Korean Society of Food Sci & Technology. 2010. Incheon. 11–75.
31. Kleinsek DA, Dugan RE, Baker TA, Porter JW. Lowenstein JM, editor. 3-Hydroxy-3-methylglutaryl coenzyme A reductase from rat liver. Methods in Enzymology. 1981. Vol. 71. New York: Academic Press;462–479.
32. Hirano R, Kondo K, Iwamoto T, Igarashi O, Itakura H. Effects of antioxidants on the oxidative susceptibility of low-density lipoprotein. J Nutr Sci Vitaminol (Tokyo). 1997. 43:435–444.


33. Woo MN, Bok SH, Choi MS. Hypolipidemic and body fat-lowering effects of Fatclean in rats fed a high-fat diet. Food Chem Toxicol. 2009. 47:2076–2082.


34. Yang SY, Hong S, Sung MK, Kang MH, Kim MK. Effect of lecithin intake on lipid metabolism and antioxidative capacity in rats fed high fat diet. Korean J Nutr. 2007. 40:312–319.
35. Reeves PG, Nielsen FH, Fahey GC Jr. AIN-93 purified diets for laboratory rodents: final report of the American Institute of Nutrition ad hoc writing committee on the reformulation of the AIN-76A rodent diet. J Nutr. 1993. 123:1939–1951.


36. Cho SH, Lee HR, Kim TH, Choi SW, Lee WJ, Choi Y. Effects of defatted safflower seed extract and phenolic compounds in diet on plasma and liver lipid in ovariectomized rats fed high-cholesterol diets. J Nutr Sci Vitaminol (Tokyo). 2004. 50:32–37.


37. Yagi K. A simple fluorometric assay for lipoperoxide in blood plasma. Biochem Med. 1976. 15:212–216.


38. Chow LW, Wang SJ, Duh PD. Antibacterial activity of burdock. Food Sci. 1997. 24:195–202.
40. Sung HY, Jeoung HJ, Choi YS, Cho SH, Yun JW. Effects of chicory inulin and oligosaccharides on lipid metabolism in rats fed a high-cholesterol diet. J Korean Soc Food Sci Nutr. 2004. 33:305–310.


41. Her BY, Sung HY, Choi YS. Oligosaccharide-supplemented soy ice cream for diabetic patients: quality characteristics and effects on blood sugar and lipids in streptozotocin-induced diabetic rats. Korean J Nutr. 2005. 38:663–671.
42. Lee YS, Kang EY, Park MN, Choi YY, Jeon JW, Yun SS. Effects of sn-2 palmitic acid-fortified vegetable oil and fructooligosaccharide on calcium metabolism in growing rats fed casein based diet. Nutr Res Pract. 2008. 2:3–7.


43. Jung UJ, Baek NI, Chung HG, Jeong TS, Lee KT, Lee MK, Choi MS. Antilipogenic and hypolipidemic effects of ethanol extracts from two variants of Artemisia princeps Pampanini in obese diabetic mice. J Med Food. 2009. 12:1238–1244.


44. Kwon EY, Do GM, Cho YY, Park YB, Jeon SM, Choi MS. Anti-atherogenic property of ferulic acid in apolipoprotein E-deficient mice fed Western diet: comparison with clofibrate. Food Chem Toxicol. 2010. 48:2298–2303.


45. Sri Balasubashini M, Rukkumani R, Menon VP. Protective effects of ferulic acid on hyperlipidemic diabetic rats. Acta Diabetol. 2003. 40:118–122.


46. Oyagbemi AA, Saba AB, Azeez OI. Molecular targets of [6]-gingerol: Its potential roles in cancer chemoprevention. Biofactors. 2010. 36:169–178.

