Abstract
Hemoglobin and zinc protoporphyrin (ZPP) tests are commonly used to screen for iron deficiency, but little research has been done to systematically evaluate the sensitivity and specificity of these two tests. The goal of this study was to evaluate the effectiveness of zinc protoporphyrin/heme (ZPP/H) ratio as a point-of-service screening test for iron deficiency among preschool-aged children by comparing the sensitivity and specificity of hemoglobin, ZPP/H ratio, and serum ferritin (SF). Also completed were assessments for the prevalence of anemia, iron deficiency (ID), and iron deficiency anemia (IDA) with indicators of ferritin models. This study was carried out with 95 children ages 3 to 6 y. Anthropometric measurements were assessed, and blood samples were analyzed for hemoglobin, SF, transferrin saturation (TS), and ZPP. Anemia was common and the prevalences of anemia, ID, and IDA were 14.7%, 12.6%, and 5.2%, respectively. The ZPP/H ratio was strongly and significantly correlated with hemoglobin. And ZPP/H ratio was a more sensitive test for ID than hemoglobin or SF measurement, correctly identifying more than twice as many iron-deficient children (sensitivity of 91.7%, compared to 41.7% for hemoglobin and SF). However, ZPP/H ratio had lower specificity (60.2%, compared to 89.1% for hemoglobin or 96.4% for SF) and resulted in the false identification of more subjects who actually were not iron deficient than did hemoglobin or SF. Low hemoglobin concentration is a late-stage indicator of ID, but ZPP/H ratio can detect ID at early stages and can be performed easily at a relatively low cost. Therefore, ZPP/H ratio can serve as a potential screening test for pre-anemic iron deficiency in community pediatric practices.
Although the prevalence of iron deficiency anemia (IDA) has decreased over the past decade, recent studies indicate that it remains relatively high among low income, preschool-aged children [1]. At the same time, iron deficiency (ID) without anemia remains relatively common at some stages of development, i.e., infants and young children [2].
Anemia (low hemoglobin concentration) is a late-stage indicator of ID and has serious negative consequences on health, mental development, and cognitive achievement [3-5]. Nevertheless, iron in the central nervous system decreases before restriction of red cell production, and therefore, the cognitive defects due to iron deficiency may precede the hematologic manifestations of anemia [4]. Furthermore, the prevalence of ID without anemia is much greater than the prevalence of ID with anemia [3,6], rendering the study of ID critical for greater public health benefits.
Because many factors contribute to the vulnerability of infants and children to negative iron balance and deficiency, there is a strong need for a simple, accurate, and sensitive screening test that is effective at an earlier stage of ID without anemia [7]. ZPP/H ratio has potential as a screening test for this purpose because it can detect ID at early stages and can be performed relatively easily and at low cost in the field or clinical settings [8,9].
Hematofluorometry is currently the most widespread technique for measuring ZPP. Hematofluorometers use a drop of whole, unprocessed blood obtained by capillary sampling, and the results are available in ~1 min. Also, the results obtained with a hematofluorometer are independent of the blood volume used, and the cost of a hematofluorometer test is relatively low [10].
Protoporphyrin is an immediate precursor of the heme molecule and it needs iron for the final step of heme synthesis. When plasma iron concentration decreases and erythrocyte protoporphyrin (EPP) accumulates, increased ZPP and free erythrocyte protoporphyrin (FEP) concentration can be measured [7,11]. Although ZPP measurement obtained with a hematofluorometer reflects the fraction of blood protoporphyrin that exists as a zinc chelate, whereas EPP measurement reflects the total protoporphyrin, the results for EPP and ZPP tests should be very similar [10,12]. ZPP (EPP or FEP) values are expressed as concentrations, e.g., µg/g Hb, µg/dL blood, and µg/dL red cells, or as the ZPP/H ratio (µmol/mol heme). The latter is the most effective in assessing iron nutritional status and in diagnosing iron metabolic disorders [8,13].
Serum ferritin (SF) concentration is an early indicator of the status of iron stores and is the most specific indicator of depleted iron stores, especially when used in conjunction with other tests to assess iron status. However, the high cost of assessing SF concentration and the unavailability of clinic-based methods hamper the use of SF measurements in ID screening [14]. Also, because SF is an acute-phase protein and its level becomes elevated during infection or inflammation, its concentration is only used to the best advantage by evaluating various iron disorders in combination with ZPP/H ratio [8].
Hemoglobin can, by definition, diagnose ID only at the stage of anemia. The use of hemoglobin tests to screen children for iron status cannot identify those who are pre-anemic but iron depleted, and thus probably require iron supplementation [12]. Although a hemoglobin test is simple to perform, it is neither as sensitive nor as specific as ZPP/H, which can be used effectively in routine pediatric practices [7].
Several different iron indexes (storage, transport, end product, and receptor) for the assessment of iron nutritional status measure a different facet of iron metabolism; and therefore, they do not necessarily correlate with one another and their test results range widely in specificity and sensitivity [7,15]. In fact, no single biochemical indicator in routine use is consistently diagnostic of ID, particularly in the pre-anemic state. Although the use of multiple tests is an appropriate approach to assess the iron status of populations, it is less practical and not commonly used in clinical settings [10,16].
The sensitivity of an index refers to the ability of the index to identify and classify those persons who are actually iron deficient, as a positive test result. The specificity of an index refers to the ability of the index to identify and classify those persons who are actually iron-sufficient, as a negative test result [17].
In this study, we compared the sensitivity and specificity of hemoglobin, SF, and ZPP/H ratio to evaluate the effectiveness of the ZPP/H ratio as a point-of-service screening test for ID among preschool-aged children. We also estimated the prevalence rates of anemia, ID, and IDA with indicators of ferritin models.
One-hundred children aged 3-6 years were recruited from preschools located in Ulsan between May and June, 2004. All parents of participating children were informed of the details of the study and their written consent was obtained. Demographic questionnaires were filled out by the parents.
Of the 100 children initially enrolled in the study, 95 completed biochemical tests after the exclusion of 5 children due to their refusal of venous puncture, and technical problems related to collection.
Height to the nearest millimeter and weight to the nearest 100 g were measured by nurses working at a local public health center. In order to analyze biochemical parameters, 5 mL of blood was taken by puncture of the cubital vein. The blood was tested immediately for hemoglobin with an Automatic Coulter Counter and for ZPP with a hematofluorometer, Model ZP206 (AVIV Biomedical, Lakewood, NJ). ZPP values were measured as µg/dL and converted to µmol/mol heme (ZPP/H ratio) by calculating with the following equation [18].
Serum was harvested through centrifugation and was stored at -20℃. SF level was assessed by immunoradiometric assay (IRMA). Furthermore, serum iron (SI) and total iron binding capacity (TIBC) were determined by the colorimetric ferrosine method, and transferring saturation (TS) was calculated by dividing SI concentration by TIBC.
Anemia was defined as a hemoglobin concentration < 11.1 g/dL for 3-4 y of age or < 11.5 g/dL for 5-6 y of age [14]. IDA was defined as having ID and a low hemoglobin concentration.
Ferritin models were used to define actual ID as having abnormal values for ≥ 2 of the following 3 indicators: SF, TS, and ZPP/H ratio [19,20].
In this study, the cutoff point of SF < 15 µg/L was chosen based on the following rationale. Although cutoff points for SF as low as 10 to 12 µg/L have been used for ID in population surveys [3], the assessment of ID in the National Health and Nutrition Examination Survey (NHNES) 2003-2006 was completed with a value of 14 µg/L [20]. Furthermore, in general, the acceptable cut-off level for SF, below which iron stores are considered to be depleted, is < 15 µg/L [14,21]. Moreover, the lower limit of SF (-2SD) was 16.6 µg/L in a study of normal children and its authors suggested 15 µg/L as a working limit [22]. Finally, the American Academy of Pediatrics recommended the same level (< 15 µg/L) to confirm ID among anemic children [23].
The reference standards for ZPP/H ratio in children have not been firmly established. However, several cut-offs have been proposed for ZPP/H to define ID. A cut-off of > 40 µmol of ZPP/mol heme was recommended in an adult study using a hematofluorometer on washed erythrocytes [24], while a cut-off of > 80 µmol of ZPP/mol heme was proposed using unwashed erythrocytes to diagnose ID [8]. Soldin et al. [11] suggested a cutoff of 55.2 µmol of ZPP/mol heme in 5-9 year-old boys and girls, and Stanton et al. [18] suggested the lead-screening cutoff for Protofluor-Z as 70 µmol of ZPP/mol heme. In a study of low-income, preschool-aged children, Crowell et al. [1] used a ZPP level of > 69 µmol/mol heme as an indicator of iron depletion. Thus, a ZPP level of > 70 µmol/mol heme was determined as a reasonable indicative cutoff of iron depletion in this study.
Although a TS of less than 16% among adults is often used to confirm ID [14], TS cut-offs of < 14% at 3-4 y and < 15% at 5-6 y of age, which were used in the analysis of NHANES II data [19], were used for abnormal values in this study. The thresholds for defining the abnormal values of hemoglobin, TS, SF, and ZPP/H ratio for ID are shown in Table 1.
Data processing and statistical analyses were performed using SPSS software (version 12.0). Stepwise multiple linear regression analyses were completed to determine the factors associated with iron status, for age, sex, height, and weight as independent variables. Correlations and linear regression analysis to examine the relationships between iron status indicators (hemoglobin, SF, TS, and ZPP/H ratio) were performed after controlling for age.
Mean values were compared using Student's t test for iron status indicators between the 3-4 y and 5-6 y aged groups. Frequencies were run for prevalence data.
The positive predictive value, sensitivity, and specificity of hemoglobin, SF, and ZPP/H ratio were calculated in screening for ID, defined as having abnormal values for ≥ 2 of the following 3 indicators: SF, TS, and ZPP/H ratio (ferritin models). The numerical definitions are shown in Table 2.
The demographic characteristics of the participating children are shown in Table 3. The ages of the children participating in this study were 3-6 y old, and 45% of the 95 children were female. Mean weight and height were 18.1 ± 3.1 kg and 104.7 ± 6.9 cm, respectively.
Table 4 shows the factors associated with iron status by stepwise multiple linear regression analysis. Height was the strongest predictor of hemoglobin (P = 0.008) and SF (P = 0.019) in the children. SF and ZPP/H ratio were associated with age, and significantly declined with increasing age (P < 0.001 and P = 0.002, respectively). Weight and sex were not significantly associated with any measurement of iron status in the children (data not shown).
Because SF concentration and ZPP/H ratio had a strong association with age, correlations between the iron status indicators were performed after controlling for age in linear regression analysis. The results showed a statistically significant correlation between ZPP/H ratio and hemoglobin (r = -0.444, P < 0.001), but no significant correlation between ZPP/H ratio and SF concentration or TS (Table 5). The correlations by linear regression analysis between hemoglobin and ZPP/H ratio (P < 0.001) and between hemoglobin and SF are shown in Fig. 1.
The mean values of iron status indicators by age group are shown in Table 6. Hemoglobin concentration and TS were lower in the children aged 3-4 y than in those aged 5-6 y. However, SF concentration and ZPP/H ratio were higher in the children aged 3-4 y than in those aged 5-6 y. All Indicators were significantly different between these two age groups.
Table 7 summarizes the assessment results for iron status indicators of ferritin models and hemoglobin. Anemia and poor iron status were common in the 3-6 y old children in this study. Furthermore, 14.7% of the children were anemic, based on hemoglobin measurements. The prevalence of ID was also determined based on the combination of three test results (TS, SF, and ZPP/H ratio) and was evident in 12.6% of the children. IDA was present in 5 out of 12 iron-deficient children, accounting for 5.2%. Also, 14.7% of the children had low TS and 8.4% had a low SF concentration, while 46% showed a high ZPP/H ratio.
The sensitivity and specificity of current cutoffs for hemoglobin, ZPP/H ratio, and SF in identifying ID are shown in Table 8. The current cutoffs for hemoglobin and SF showed low sensitivity (0.417), but high specificity (0.891 for hemoglobin, 0.964 for SF). In contrast, ZPP/H ratio cutoffs showed very high sensitivity (0.971) and good specificity (0.602). The PPVs for hemoglobin and ZPP/H ratio were 0.357 and 0.333, respectively, and lower than that for SF (0.625).
Multiple linear regression analysis found that hemoglobin, SF, and ZPP/H ratio were significantly associated with height and age. In fact, assessments of hemoglobin and ZPP/H ratio in children aged 5-6 y indicated better iron status compared to those aged 3-4 y.
There was a significant correlation between higher ZPP/H ratio and lower age of the children; median ZPP concentration declined with increasing age [11]. Mean ZPP (EP) increased from 6 months of age and reached a peak value at 1.5 y, and then gradually fell to a steady value around 6 y of age. This result likely reflects immature heme synthesis and increased erythrocyte volume in younger aged children [7,11,22].
SF measurement was previously reported to increase progressively with age throughout the first 12 y of life [22]. In contrast, an inverse correlation of SF with age was observed in our study, and the mean SF value was lower in 5-6 y olds than in 3-4 y olds. This result implies a higher prevalence of inadequate iron supply for relatively accelerated heme production due to growth.
The second important finding of this study is that the prevalence rates of anemia (14.7%), IDA (5.2%), and ID (12.6%) for the given cutoffs point were higher than other recently reported results from developed countries [25-27], showing a prevalence less than 2% for anemia, around 1% for IDA, and 2.8-4.5% for ID. Nevertheless, our results are similar to those of a study in low income, preschool-aged children, who had a 5% prevalence of IDA and 11.7% of ID [1]. Comparisons of data across different independent studies can be challenging due to the following reasons: the use of single vs. multiple variables, variations in cutoff values, the methods of survey and laboratory analysis, the size of sample populations, and the range of age within subject groups [19]. However, according to the Center for Disease Control and Prevention, the risk factors for iron deficiency in children greater than 3 years of age include low family income, a low-iron diet, inflammation, and bleeding disorders [14]. Furthermore, anemia may result from a variety of underlying conditions besides iron deficiency, such as other nutritional deficiencies (eg, vitamin B12 or folate deficiency), thalassemia, malaria, or other infections [14]. Although anemia is in itself not specific for ID, we cannot overlook our results of higher prevalences of anemia, IDA, and ID. Thus, further research to control and prevent iron deficiency status in preschool-aged children needs to be supported by policy.
Another important finding of this study is that the sole use of hemoglobin or SF values was not sufficient to identify all subjects who were ID. At the same time, a simple, inexpensive, point-of-service ZPP/H screening test identified significantly more iron-deficient children than hemoglobin and SF measurements. Other studies showed that ZPP was the most sensitive and SF the most specific in identifying children with IDA [12,28]. Also, hemoglobin measurements had a low sensitivity (23-40%) for predicting ID based on receiver operating characteristic (ROC) curves [16], and lacked sensitivity (11%) among preschool-aged children in a study by Crowell et al. [1].
In this study, we found the ZPP/H ratio to be a more sensitive test for ID than hemoglobin or SF measurement, correctly identifying more than twice as many iron-deficient children (sensitivity of 91.7% for ZPP/H ratio, compared to 41.7% for hemoglobin or SF). In other words, the use of ZPP/H ratio alone as a screening test for ID missed only 8.3% of the iron-deficient children, while hemoglobin or SF measurements missed 58.3% of the iron-deficient children. Therefore, it seems necessary to include ZPP/H ratio in future screening protocols for the early detection of ID among young children.
However, the ZPP/H ratio had lower specificity (60.2% compared to 89.1% for hemoglobin or 96.4% for SF) and resulted in false identification of more subjects who were not actually ID than did hemoglobin or SF. The false-positive rate with the established hemoglobin cutoff value was only 11% (~1 of every 10 children with a normal iron status test), whereas the false-positive rate for ZPP/H ratio was 40% (4 of every 10 normal children tested). A positive ZPP test may necessitate additional confirmatory testing (e.g., SF measurement) and result in unnecessary burdens associated with cost for the overall health care system. This is the only disadvantage of using the ZPP test as a screening tool for ID. Thus, we believe, as Crowell et al. [1] suggested, that policy decisions regarding the implementation of ZPP tests be based on considerations of whether the positive effect of correctly identifying more than twice as many iron deficient children can counterbalance the price burden incurred due to additional follow-up tests on 3 of every 10 children with normal iron status.
An increase in ZPP is the first measurable biochemical change in erythrocytes after a decline in iron status [8,28]. However the use of ZPP ratio for detecting ID in the pre-anemic stage has been rare. The general "gold standard" test for ID is bone marrow iron storage measurement, but this test is too costly and invasive for routine screening or monitoring. This limitation underscores a key benefit of the zinc protoporphyrin (ZPP) test, which has been shown to reflect bone marrow iron available during erythrocyte production [8].
The ZPP/H ratio was the most highly correlated predictor of hemoglobin concentration in this study, which was also found in other studies [7,28], suggesting the potential for ZPP/H ratio to replace hemoglobin measurements in community pediatric practices.
In conclusion, ZPP/H ratio is capable of predicting iron deficiency nearly as well as two or three laboratory tests combined, and by itself, it can detect pre-anemic deficiency better than other individual tests. Furthermore, ZPP/H ratio is a cost-effective test that is performed using as little as one drop of capillary blood. Thus, the present study confirms that the use of ZPP/H ratio deserves further consideration in examining individuals and populations for ID.
Figures and Tables
Table 1
Cutoffs for defining abnormal values of hemoglobin and transferrin saturation, serum ferritin, and ZPP/H ratio, which were considered indicative of iron deficiency for ferritin models
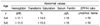
Table 2
Numerical definitions of sensitivity, specificity, and positive predictive value to assess iron deficiency in a sample group
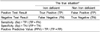
Table 4
Stepwise multiple linear regression analysis of factors associated with iron status in young children
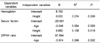
References
1. Crowell R, Ferris AM, Wood RJ, Joyce P, Slivka H. Comparative effectiveness of zinc protoporphyrin and hemoglobin concentrations in identifying iron deficiency in a group of low-income, preschool-aged children: practical implications of recent illness. Pediatrics. 2006. 118:224–232.


2. Cook JD, Skikne BS, Baynes RD. Iron deficiency: the global perspective. Adv Exp Med Biol. 1994. 356:219–228.


3. Halterman JS, Kaczorowski JM, Aligne A, Auinger P, Szilagyi PG. Iron deficiency and cognitive achievement among school-aged children and adolescents in the United States. Pediatrics. 2001. 107:1381–1386.


4. Yehuda S, Youdim MB. Brain iron: a lesson for animal models. Am J Clin Nutr. 1989. 50:618–629.
5. Lozoff B, Jimenez E, Wolf AW. Long-term developmental outcome of infants with iron deficiency. N Engl J Med. 1991. 325:687–694.


6. Looker AC, Dallman PR, Carroll MD, Gunter EW, Johnson CL. Prevalence of iron deficiency in the United States. JAMA. 1997. 277:973–976.


7. Rettmer RL, Carlson TH, Origenes ML, Jack RM, Labb RF. Zinc protoporphyrin/heme ratio for diagnosis of preanemic iron deficiency. Pediatrics. 1999. 104:e37.


8. Labbé RF, Vreman HJ, Stevenson DK. Zinc protoporphyrin: a metabolite with a mission. Clin Chem. 1999. 45:2060–2072.


9. Yip R, Schwartz S, Deinard AS. Screening for iron deficiency with the erythrocyte protoporphyrin test. Pediatrics. 1983. 72:214–219.


10. Mei Z, Parvanta I, Cogswell ME, Gunter EW, Grummer-Strawn LM. Erythrocyte protoporphyrin or hemoglobin: which is a better screening test for iron deficiency in children and women? Am J Clin Nutr. 2003. 77:1229–1233.


11. Soldin OP, Miller M, Soldin SJ. Pediatric reference ranges for zinc protoporphyrin. Clin Biochem. 2003. 36:21–25.


12. Siegel RM, LaGrone DH. The use of zinc protoporphyrin in screening young children for iron deficiency. Clin Pediatr (Phila). 1994. 33:473–479.


13. Zimmermann MB, Molinari L, Staubli-Asobayire F, Hess SY, Chaouki N, Adou P, Hurrell RF. Serum transferrin receptor and zinc protoporphyrin as indicators of iron status in African children. Am J Clin Nutr. 2005. 81:615–623.


14. Centers for Disease Control and Prevention. Recommendations to prevent and control iron deficiency in the United States. MMWR Recomm Rep. 1998. 47:1–29.
15. Hastka J, Lasserre JJ, Schwarzbeck A, Reiter A, Hehlmann R. Laboratory tests of iron status: correlation or common sense? Clin Chem. 1996. 42:718–724.


16. Schneider JM, Fujii ML, Lamp CL, Lönnerdal B, Dewey KG, Zidenberg-Cherr S. Anemia, iron deficiency, and iron deficiency anemia in 12-36-mo-old children from low-income families. Am J Clin Nutr. 2005. 82:1269–1275.


17. Gibson RS. Principles of nutritional assessment. 1990. New York: Oxford University Press.
18. Stanton NV, Gunter EW, Parsons PJ, Field PH. Empirically determined lead- poisoning screening cutoff for the Protofluor-Z hematofluorometer. Clin Chem. 1989. 35:2104–2107.


19. Expert Scientific Working Group. Summary of a report on assessment of the iron nutritional status of the United States population. Am J Clin Nutr. 1985. 42:1318–1330.
20. Cogswell ME, Looker AC, Pfeiffer CM, Cook JD, Lacher DA, Beard JL, Lynch SR, Grummer-Strawn LM. Assessment of iron deficiency in US preschool children and nonpregnant females of childbearing age: National Health and nutrition Examination Survey 2003-2006. Am J Clin Nutr. 2009. 89:1334–1342.


21. WHO. UNICEF. UNU. Iron deficiency anemia: assessment, prevention, and control. A guide for program managers. 2001. Geneva: World Health Organization;1–114.
22. Deinard AS, Schwartz S, Yip R. Developmental changes in serum ferritin and erythrocyte protoporphyrin in normal (nonanemic) children. Am J Clin Nutr. 1983. 38:71–76.


23. American Academy of Pediatrics, Committee on Nutrition. Kleinman RE, editor. Iron deficiency. Pediatric Nutrition Handbook. 2003. 5th ed. ELK Grove Village, IL: American Academy of Pediatrics;299–311.
24. Hastka J, Lasserre JJ, Schwarzbeck A, Strauch M, Hehlmann R. Washing erythrocytes to remove interferents in measurements of zinc protoporphyrin by front-face hematofluorometry. Clin Chem. 1992. 38:2184–2189.


25. Öhlund I, Lind T, Hörnell A, Hernell O. Predictors of iron status in well-nourished 4-y-old children. Am J Clin Nutr. 2008. 87:839–845.


26. Gunnarsson BS, Thorsdottir I, Palsson G. Iron status in 6-y-old children: associations with growth and earlier iron status. Eur J Clin Nutr. 2005. 59:761–767.

