Abstract
We compared the effects of genistein and daidzein on the expression of chemokines, cell adhesion molecules (CAMs), and endothelial nitric oxide synthase (eNOS) in tumor necrosis factor (TNF)-α-stimulated human umbilical vascular endothelial cells (HUVECs). TNF-α exposure significantly increased expression of monocyte chemoattractant protein (MCP)-1, vascular adhesion molecule (VCAM)-1, and intercellular adhesion molecule-1. Genistein significantly decreased MCP-1 and VCAM-1 production in a dose-dependent manner, whereas CAM expression was not significantly lowered by genistein treatment. However, daidzein slightly decreased MCP-1 production. The effects of genistein and daidzein on MCP-1 secretion coincided with mRNA expression. Pre-treatment with either genistein or daidzein elevated eNOS expression and nitric oxide production disturbed by TNF-α exposure. A low concentration of isoflavones significantly inhibited nuclear factor (NF)κB activation, whereas a high dose slightly ameliorated these inhibitive effects. These results suggest that genistein had a stronger effect on MCP-1 and eNOS expression than that of daidzein. Additionally, NFκB transactivation might be partially related to the down-regulation of these mRNAs in TNF-α-stimulated HUVECs.
Several lines of evidence suggest that soy isoflavones exert anti-atherogenic effects in mice, rabbits, monkeys, and humans [1-4]. Antihypertensive effects of isoflavones have also been proposed in spontaneously hypertensive rats and humans with mild to moderate essential hypertension [5-7]. The early stage of atherosclerosis is characterized by increased levels of inflammatory cytokines, oxidative stress, and the expression of adhesion molecules on vascular endothelium. Various cell adhesion molecules (CAMs), such as vascular cell adhesion molecule (VCAM)-1, intercellular adhesion molecule (ICAM)-1, and monocyte chemoattractant protein (MCP)-1, have been identified in endothelial cells [8,9]. Expression of CAMs and MCP-1 in endothelial cells is stimulated by interleukin (IL)-1β, tumor necrosis factor (TNF)-α, and lipopolysaccharide (LPS), which serve as signals to facilitate nuclear factor (NF)κB transactivation [10-12]. CAM expression is closely related to NFκB upregulation [8,13]. The endothelium also produces nitric oxide (NO), synthesized by endothelial nitric oxide synthase (eNOS), which is a vasoprotective and anti-atherosclerotic agent [5,6,14]. An eNOS isoform is constitutively expressed and can be activated and inhibited by phosphorylation through various protein kinases [5,15-17]. Therefore, any chemicals that suppress CAMs or enhance eNOS activity and expression in the vascular endothelium may be usable as therapeutic agents against the development of atherosclerosis.
Soy isoflavones are comprised of genistein (4',5,7-trihydroxyisoflavone), daidzein (4',7-dihydroxyisoflavone), glycitein (6-methoxydaidzein), and their glycosides. The molecular mechanisms of how these isoflavones affect the development and progression of atherosclerosis has not been thoroughly studied. However, genistein, a protein kinase inhibitor, exhibits anti-oxidative and anti-inflammatory activities, such as scavenging free radicals to prevent reactive oxygen species (ROS)-mediated DNA damage [18-21] and suppression of NO production through down-regulation of inducible NOS (iNOS) expression and NFκB transactivation [16,17,22]. However, contradicting data have been reported on the effect of genistein on CAM activities and mRNA expression induced by TNF-α in endothelial cells [11,12,23]. Furthermore, little information is available about the effects of daidzein, another major component of soy isoflavones, on NFκB activity and eNOS expression, which regulate CAM expression and bioactive NO production in endothelial cells. These questions led us to compare the effects of genistein and daidzein on MCP-1, eNOS, and CAM expression in TNF-α-stimulated human umbilical vascular endothelial cells (HUVECs) and to investigate possible mechanisms of action of their anti-atherosclerotic effect.
Genistein and daidzein were purchased from Sigma (St. Louis, MO, USA). EGM-2 Bullet Kit, HBSS, trypsin/EDTA solution, and trypsin neutralizing solution were obtained from Lonza (Basel, Switzerland). Human sVCAM-1 and MCP-1 enzyme linked immunosorbent assay (ELISA) kits were purchased from R&D Systems (Minneapolis, MN, USA).
HUVECs were purchased from Modern Tissue Technologies Inc. (Seoul, South Korea) and cultured in 75-cm2 flasks with medium from the EGM-2 Bullet Kit, which contained 10% fetal bovine serum, hydrocortisone, human fibroblastic growth factor-B, vascular endothelial growth factor, R3-insulin-like growth factor-I, ascorbic acid, human epidermal growth factor, GA-1000, and heparin. Confluent cultures were washed with HEPES buffered saline solution. The collected cells were pelleted by centrifugation at room temperature for 10 min at 500 × g, the supernatant was discarded, and the cell pellet was gently resuspended in EGM-2 Bullet Kit medium. HUVECs were seeded at a density of 1 × 106 cells/100 mm dish and incubated for 2 days at 37℃ in a humidified 5% CO2 incubator. The medium was replaced every 2 days until confluence (3-5 days). Genistein and daidzein were dissolved in dimethyl sulfoxide and stored at -20℃. Cells were pretreated with or without genistein and daidzein (20, 50, or 100 µM) for 2 hr before stimulation with or without TNF-α (10 ng/mL) for 20 hr. Endothelial cell cultures were used at passages 3-6 in all experiments.
Cell viability was assessed by measuring the uptake of neutral red supravital dye by viable cells according to the procedure of Fautz et al. [24]. After culturing the cells as described above, the medium was removed and replaced with 0.5 mL fresh medium containing 1.14 mM neutral red. After a 3-hr incubation, the medium was removed, and the cells were washed twice with phosphate buffered saline solution (pH 7.4). The incorporated neutral red was released from the cells by a 15-min incubation at room temperature in 1 mL of cell lysis buffer. To measure the dye taken up, the cell lysis products were centrifuged and absorbance of the supernatant was measured spectrophotometrically at 540 nm.
The concentrations of sVCAM-1 and MCP-1 were measured in the culture supernatants utilizing a highly sensitive ELISA according to the manufacturer's instructions (R&D Systems Inc.).
Total RNA was isolated using Trizol-reagent (Invitrogen, Carlsbad, CA, USA) and first strand cDNA was synthesized using the Superscript First Strand cDNA Synthesis kit (Invitrogen), according to the manufacturer's methods. The PCR primer sequences are shown in Table 1. The amplification profile consisted of an initial denaturation at 94℃ for 5 min followed by denaturation at 94℃, annealing from 58℃ to 63℃ and extension at 72℃ for 1 min. The total number of cycles for the best amplification profiles was 35, 22, 30, 22, and 20 for eNOS, VCAM-1, ICAM-1, MCP-1, and GAPDH, respectively. The intensity of the PCR products was densitometrically measured using the Gel Doc EQ System (Bio-Rad, Hercules, CA, USA). All signals were normalized to GAPDH house keeping gene mRNA levels and expressed as ratios.
Nitrite that accumulated in the culture medium was used as an indicator of NO production according to the Griess reaction [25]. One hundred µL of each medium supernatant was mixed with 50 µL of 1% sulfanilamide (in 5% phosphoric acid) and 50 µL of 0.1% naphthylenediamine dihydrochloride and incubated at room temperature for 10 min. The absorbance was measured at 550 nm against a NaNO2 serial dilution standard curve, and nitrite production was determined.
Nuclear protein was extracted with a slight modification of the protocol from Dignam et al. [26]. Cells in 10 mm dishes were lysed with buffer, vortexed, kept on ice for 5 min, and centrifuged at 500 × g for 5 min at 4℃. Pelleted nuclei were resuspended in 50 µL extraction buffer. Following gentle mixing and an incubation on ice for 20 min, the samples were centrifuged at 500 × g for 5 min at 4℃. The supernatant fraction was transferred to new tubes and stored at -70℃. Protein concentration was determined by the Bradford assay. For the EMSA, NFκ B-specific oligonucleotide was end-labeled with [γ-32P]-ATP using T4 polynucleotide kinase (Promega, Madison, WI, USA) and purified using a Microspin G-25 column (GE Healthcare, Buckinghamshire, UK). EMSAs were performed according to the Promega instruction manual. Five µg of nuclear protein, binding buffer, 32P-labeled NFκB, and loading buffer were incubated for 30 min at room temperature. DNA-protein complexes were separated from unbound DNA probe by electrophoresis on 4% polyacrylamide gels with 0.5 × Tris-Borate EDTA buffer used as the running buffer. The gels were exposed to a phosphor screen (PerkinElmer, Waltham, MA, USA) for 2 hr at -20℃, and the bands were quantified with a phosphor imager (PerkinElmer).
All data are expressed as means ± SDs. Statistical analyses were performed using the SPSS program (SPPS, Inc., Chicago, IL, USA. A one-way analysis of variance and Duncan's multiple range test were used to examine the differences among treatments. P-values < 0.05 were considered significant.
To examine the effects of genistein and daidzein on the expression of MCP-1 and CAMs, HUVECs were exposed to different concentrations (20-100 µM) of either genistein or daidzein and then stimulated with TNF-α. Cell viability was > 95% at the concentrations treated, as assessed by the neutral red assay (Fig. 1). Exposure of HUVECs to TNF-α induced significant MCP-1 and CAM expression, such as VCAM-1 and ICAM-1. Pre-incubation with genistein significantly (P < 0.05) decreased MCP-1 expression and production in a dose-dependent manner (Fig. 3A, 4A, and 4B). VCAM-1 production also decreased with genistein treatment (Fig. 2A), whereas VCAM-1 and ICAM-1 mRNA expression was not significantly decreased by genistein treatment (Fig. 4). However, daidzein did not affect VCAM-1 and ICAM-1 mRNA expression or VCAM-1 production (Fig. 2B, 5). Instead, MCP-1 production and expression were slightly lowered by daidzein treatment (Fig. 3B, 5), and its effect was smaller than that of genistein. These data suggest that genistein had a stronger effect on MCP-1 and CAM expression than that of daidzein.
Exposure of HUVECs to TNF-α downregulated eNOS expression and caused a significant decrease in NO production. Pre-treatment of genistein and daidzein with TNF-α significantly elevated eNOS expression and NO production (P < 0.05, Fig. 6).
TNF-α induced NFκB transactivation in HUVECs. Pre-incubating HUVECs with 20 µM genistein or daidzein inhibited NFκB activity. However, the inhibitory effect on NFκB transactivation was slightly ameliorated at > 50 µM (Fig. 7).
Early development of atherosclerosis is closely related with oxidative stress and inflammatory processes, such as the expression of chemokines and CAMs on endothelial cells. Several studies have reported that genistein and isoflavone supplementation in animal models improves endothelial dysfunction by regulating CAM and eNOS levels [2,5,6,8]. However, human trials do not consistently support the protective effects of higher isoflavone intake on cardiovascular disease risk [27], which might be due to the composition of the isoflavones used, study duration, or the supplementation amount. Therefore, this study was designed to compare the efficacy of genistein and daidzein, major soy isoflavones, on the regulation of chemokines, CAMs, and eNOS levels.
Genistein had a stronger inhibitory effect than daidzein on MCP-1 and CAM expression and production. Pro-inflammatory cytokines, such as TNF-α and IL-1β, are well known inducers of vascular adhesion molecules, including VCAM-1 and MCP-1 [10,11,28]. Tyrosine kinase inhibitors block transactivation of NFκB, a transcription factor that regulates CAMs and is induced by TNF-α and IL-1β [11,12,23]. Our study revealed that genistein, a tyrosine kinase inhibitor, selectively suppressed TNF-α-stimulated production of VCAM-1 and MCP-1 but not ICAM-1 mRNA expression. Furthermore, our study showed that genistein is not an effective VCAM-1 inhibitor compared to that of MCP-1. The inhibition of MCP-1 expression was dose-dependent and correlated with soluble MCP-1 concentrations. May et al. [11] found that genistein inhibits VCAM-1 expression induced by TNF-α or IL-1 in HUVECs, whereas it does not affect ICAM-1 expression. The selectivity of genistein for MCP-1 and VCAM-1 regulation may be desirable, as ICAM-1 is involved in leukocyte adhesion, whereas MCP-1 and VCAM-1 are primarily involved in adhesion of monocytes and lymphocytes found in atherosclerotic lesions [29]. However, CAM regulation may be affected by stimulus type. Weber et al. [28] reported that genistein does not affect CAM upregulation by phorbol myristic acid. Majewska et al. [13] also found that pre-treatment of HUVECs with genistein (10 µg/mL) for 30 min before IL-1 stimulation does not affect ICAM-1 and VCAM-1 expression. Chen et al. [30] found that isoflavoids have no obvious effect on neutrophilic CAM expression and concluded that isoflavoids protect the cardiovascular system through other mechanisms but not CAM regulation. Furthermore, genistein is ineffective for reducing LPS-stimulated E-selectin expression in HUVECs [12].
In contrast, daidzein showed very weak suppressive effects against MCP-1 expression and soluble protein excretion but no effect on ICAM-1 and VCAM-1 levels up to 100 µM. Gottstein et al. [23] also reported that daidzein decreases TNF-α-induced secretion of MCP-1 in HUVECs. However, we found that daidzein exerted weaker inhibitory effects than those of genistein for the expression of all CAMs. Our data agree with prior findings that genistein is a stronger suppressor of CAM expression than that of daidzein in TNF-α-stimulated HUVECs. Moreover, the present data contributes to our knowledge of the molecular mechanisms by which isoflavones may protect against coronary artery disease.
Genistein improves vascular reactivity by regulating eNOS, though the molecular mechanism is not clear. Our results showed that NO production and eNOS expression were elevated by preincubating HUVECS with genistein before TNF-α stimulation. Several studies have confirmed the stimulatory effect of genistein on NO and eNOS levels. Genistein acutely stimulates eNOS synthesis in vascular endothelial cells within a 10 minute incubation period through the cAMP/protein kinase A cascade without affecting PI3K/Akt or the ERK/MAPK signaling pathways [15]. Furthermore, genistein supplementation in spontaneously hypertensive rats shows a direct effect on transcription in vascular walls, leading to increased eNOS expression, thereby improving hypertension [5,7]. In contrast, soy isoflavone does not affect eNOS expression in Watanabe heritable hyperlipidemic rabbits [31]. These findings suggest that eNOS can be regulated by genomic and nongenomic actions.
Studies reporting the effect of daidzein on eNOS levels are scarce. In our study, the significance of genistein and daidzein on NO production and eNOS expression was compared. Genistein (20 µM) significantly elevated NO production and eNOS expression, whereas daidzein showed a significant difference only at 100 µM. Thus, daidzein was less effective at enhancing NO production and eNOS expression than genistein. The mechanism by which daidzein regulates eNOS seems to be somewhat different than that of genistein. Woodman et al. [32] proposed that daidzein enhances eNOS activity through an increase in calmodulin and a decrease in caveolin-1.
MCP-1 and CAM expression in endothelial cells is stimulated by IL-1β, TNF-α, and LPS, which serve as signals facilitating NFκB transactivation [10-12,33]. Our results indicated that both genistein and daidzein down-regulated CAM expression, and that this action was mediated, in part, through down-regulation of NFκB transactivation. Although we have demonstrated that TNF-α rapidly induced NFκB nuclear translocation in HUVECs, genistein and daidzein did not block NFκB translocation in a dose-dependent manner. In this study, the effects of genistein and daidzein on NFκB transactivation were biphasic; 20 µM of either isoflavone suppressed NFκB transactivation, whereas > 50 µM slightly increased NFκB transactivation. A similar trend was found by Guo et al. [34], who reported that daidzein stimulates cancer cell growth at low concentrations (1 µM) but inhibits cell growth at higher concentrations. This implies that regulation of CAM expression by genistein and daidzein is not solely dependent on the NFκB signaling pathway. Several researchers have reported that regulation of CAM expression does not necessarily require the NFκB signaling pathway but depends on the stimulus and cell type. That is, genistein as a tyrosine kinase cannot block NFκB translocation at the concentration that inhibits iNOS and COX-2 mRNA expression in rat mesangial cells [35]. IL-4-induced upregulation of the VCAM-1 gene in HUVECs does not appear to be involved with NFκB at the transcriptional level [36].
From the above results, it can be concluded that genistein and daidzein significantly suppressed MCP-1 and VCAM-1 production and elevated eNOS expression in TNF-α-stimulated HUVECs, although the genisteineffect was stronger than that of daidzein. Amelioration of NFκB transactivation might be, in part, related to MCP-1 and CAM downregulation. The ability to regulate CAM and NFκB expression, which are activated in atherosclerotic lesions, may explain the molecular mechanisms of genistein and daidzein to retard development of the initial stage of atherosclerosis. Genistein and daidzein enhanced eNOS expression and NO production, which are promising results in the search for treatments to maintain healthy blood pressure and prevent atherosclerosis.
Figures and Tables
Fig. 1
Effects of genistein and daidzein on cell viability in tumor necrosis factor (TNF)-α-stimulated human umbilical vascular endothelial cells (HUVECs). Cells (1 × 105 cells) in 96-well plates were preincubated with and without the indicated concentrations of genistein or daidzein for 2 hr and then incubated with TNF-α (10 ng/mL) for 20 hr. Data represent the means ± SD of triplicate experiments. Values sharing the same superscript are not significantly different at P < 0.05.
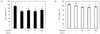
Fig. 2
Inhibitory effects of genistein and daidzein on vascular adhesion molecule (VCAM)-1 production in tumor necrosis factor (TNF)-α-stimulated human umbilical vascular endothelial cells (HUVECs). Cells (4 × 105 cells) in 24-well plates were preincubated with and without the indicated concentrations of genistein or daidzein for 2 hr and then incubated with TNF-α (10 ng/mL) for 20 hr. Data represent the means ± SD of triplicate experiments. Values sharing the same superscript are not significantly different at P < 0.05.
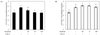
Fig. 3
Inhibitory effects of genistein and daidzein on monocyte chemoattractant protein (MCP)-1 production in tumor necrosis factor (TNF)-α-stimulated human umbilical vascular endothelial cells (HUVECs). Cells (4 × 105 cells) in 24-well plates were preincubated with and without the indicated concentrations of genistein or daidzein for 2 hr and then incubated with TNF-α (10 ng/mL) for 20 hr. Data represent the means ± SD of triplicate experiments. Values sharing the same superscript are not significantly different at P < 0.05.
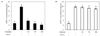
Fig. 4
Effects of genistein on mRNA gene expression in tumor necrosis factor (TNF)-α-stimulated human umbilical vascular endothelial cells (HUVECs). Cells (1 × 106 cells) in 100 mm dishes were preincubated with and without the indicated concentrations of genistein for 2 hr and then incubated with TNFα (10 ng/mL) for 20 hr. Untreated represents the negative control without TNF-α treatment. (A) Levels of mRNA were determined by RT-PCR analysis. GAPDH was used as an internal control. (B) All signals were normalized to GAPDH mRNA levels and expressed as ratios. Data represent means ± SD of triplicate experiments. Values sharing the same superscript are not significantly different at P < 0.05.
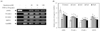
Fig. 5
Effects of daidzein on mRNA gene expression in tumor necrosis factor (TNF)-α-stimulated human umbilical vascular endothelial cells (HUVECs). Cells (1 × 106 cells) in 100 mm dishes were preincubated with and without the indicated concentrations of daidzein for 2 hr and then incubated with TNFα (10 ng/mL) for 20 hr. Untreated represents the negative control without TNF-α treatment. (A) Levels of mRNA expression were determined by RT-PCR analysis. GAPDH was used as an internal control. (B) All signals were normalized to GAPDH mRNA levels and expressed as ratios. Data represent the means ± SD of triplicate experiments. Values sharing the same superscript are not significantly different at P < 0.05.
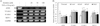
Fig. 6
Effects of genistein and daidzein on nitric oxide (NO) production in tumor necrosis factor (TNF)-α-stimulated human umbilical vascular endothelial cells (HUVECs). Cells (4 × 105 cells) in 24-well plates were preincubated with and without the indicated concentrations of genistein or daidzein for 2 hr and then incubated with TNF-α (10 ng/mL) for 20 hr. Data represent the means ± SD of triplicate experiments. Values sharing the same superscript are not significantly different at P < 0.05.
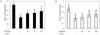
Fig. 7
Effects of genistein and daidzein on nuclear factor (NF)κB activity in tumor necrosis factor (TNF)-α-stimulated human umbilical vascular endothelial cells (HUVECs). Cells (1 × 106 cells) in 100 mm dishes were preincubated with and without the indicated concentrations of genistein or daidzein for 2 hr and then incubated with TNF-α (10 ng/mL) for 20 hr. (A) DNA binding activity of NFκB was assessed by electrophoretic mobility shift assay (EMSA)(B) Values are expressed as relative intensity of radioactivity. Data represent the means ± SD of triplicate experiments. Values sharing the same superscript are not significantly different at P < 0.05.
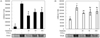
References
1. Anthony MS, Clarkson TB, Williams JK. Effects of soy isoflavones on atherosclerosis: potential mechanisms. Am J Clin Nutr. 1998. 68:1390S–1393S.


2. Anthony MS, Clarkson TB, Hughes CL Jr, Morgan TM, Burke GL. Soybean isoflavones improve cardiovascular risk factors without affecting the reproductive system of peripubertal rhesus monkeys. J Nutr. 1996. 126:43–50.


3. Lee YM, Jung MH, Lee YS, Song J. Effect of genistein and soy protein on lipids metabolism in ovariectomized rats. Korean J Nutr. 2005. 38:267–278.
4. Kirk EA, Sutherland P, Wang SA, Chait A, LeBoeuf RC. Dietary isoflavones reduce plasma cholesterol and atherosclerosis in C57BL/6 mice but not LDL receptor-deficient mice. J Nutr. 1998. 128:954–959.


5. Vera R, Sánchez M, Galisteo M, Villar IC, Jimenez R, Zarzuelo A, Pérez-Vizcaíno F, Duarte J. Chronic administration of genistein improves endothelial dysfunction in spontaneously hypertensive rats: involvement of eNOS, caveolin and calmodulin expression and NADPH oxidase activity. Clin Sci (Lond). 2007. 112:183–191.


6. Mahn K, Borrás C, Knock GA, Taylor P, Khan IY, Sugden D, Poston L, Ward JP, Sharpe RM, Viña J, Aaronson PI, Mann GE. Dietary soy isoflavone induced increases in antioxidant and eNOS gene expression lead to improved endothelial function and reduced blood pressure in vivo. FASEB J. 2005. 19:1755–1757.


7. Si H, Liu D. Genistein, a soy phytoestrogen, upregulates the expression of human endothelial nitric oxide synthase and lowers blood pressure in spontaneously hypertensive rats. J Nutr. 2008. 138:297–304.


8. Rimbach G, Boesch-Saadatmandi C, Frank J, Fuchs D, Wenzel U, Daniel H, Hall WL, Weinberg PD. Dietary isoflavones in the prevention of cardiovascular disease--a molecular perspective. Food Chem Toxicol. 2008. 46:1308–1319.


9. Berliner JA, Navab M, Fogelman AM, Frank JS, Demer LL, Edwards PA, Watson AD, Lusis AJ. Atherosclerosis: basic mechanisms. Oxidation, inflammation, and genetics. Circulation. 1995. 91:2488–2496.
10. Bowie AG, Moynagh PN, O'Neill LA. Lipid peroxidation is involved in the activation of NF-κB by tumor necrosis factor but not interleukin-1 in the human endothelial cell line ECV304. Lack of involvement of H2O2 in NF-κB activation by either cytokine in both primary and transformed endothelial cells. J Biol Chem. 1997. 272:25941–25950.


11. May MJ, Wheeler-Jones CP, Pearson JD. Effects of protein tyrosine kinase inhibitors on cytokine-induced adhesion molecule expression by human umbilical vein endothelial cells. Br J Pharmacol. 1996. 118:1761–1771.


12. Adamson P, Tighe M, Pearson JD. Protein tyrosine kinase inhibitors act downstream of IL-1 alpha and LPS stimulated MAP-kinase phosphorylation to inhibit expression of E-selectin on human umbilical vein endothelial cells. Cell Adhes Commun. 1996. 3:511–525.


13. Majewska E, Paleolog E, Baj Z, Kralisz U, Feldmann M, Tchórzewski H. Role of tyrosine kinase enzymes in TNF-alpha and IL-1 induced expression of ICAM-1 and VCAM-1 on human umbilical vein endothelial cells. Scand J Immunol. 1997. 45:385–392.


14. Lee YS, Jang SY, Kim KO. Effects of soy isoflavone intake on nitrite content and antioxidant enzyme activities in male rats fed high-fat diet. Korean J Nutr. 2005. 38:89–95.
15. Liu D, Homan LL, Dillon JS. Genistein acutely stimulates nitric oxide synthesis in vascular endothelial cells by a cyclic adenosine 5'-monophosphate-dependent mechanism. Endocrinology. 2004. 145:5532–5539.


16. Yen GC, Lai HH. Inhibitory effects of isoflavones on nitric oxide- or peroxynitrite-mediated DNA damage in RAW 264.7 cells and phiX174 DNA. Food Chem Toxicol. 2002. 40:1433–1440.


17. Sheu F, Lai HH, Yen GC. Suppression effect of soy isoflavones on nitric oxide production in RAW 264.7 macrophages. J Agric Food Chem. 2001. 49:1767–1772.


18. Mizutani K, Ikeda K, Nishikata T, Yamori Y. Phytoestrogens attenuate oxidative DNA damage in vascular smooth muscle cells from stroke-prone spontaneously hypertensive rats. J Hypertens. 2000. 18:1833–1840.


19. Ruiz-Larrea MB, Mohan AR, Paganga G, Miller NJ, Bolwell GP, Rice-Evans CA. Antioxidant activity of phytoestrogenic isoflavones. Free Radic Res. 1997. 26:63–70.


20. Wei H, Wei L, Frenkel K, Bowen R, Barnes S. Inhibition of tumor promoter-induced hydrogen peroxide formation in vitro and in vivo by genistein. Nutr Cancer. 1993. 20:1–12.


21. Kapiotis S, Hermann M, Held I, Seelos C, Ehringer H, Gmeiner BM. Genistein, the dietary-derived angiogenesis inhibitor, prevents LDL oxidation and protects endothelial cells from damage by atherogenic LDL. Arterioscler Thromb Vasc Biol. 1997. 17:2868–2874.


22. Sadowska-Krowicka H, Mannick EE, Oliver PD, Sandoval M, Zhang XJ, Eloby-Childess S, Clark DA, Miller MJ. Genistein and gut inflammation: role of nitric oxide. Proc Soc Exp Biol Med. 1998. 217:351–357.


23. Gottstein N, Ewins BA, Eccleston C, Hubbard GP, Kavanagh IC, Minihane AM, Weinberg PD, Rimbach G. Effect of genistein and daidzein on platelet aggregation and monocyte and endothelial function. Br J Nutr. 2003. 89:607–615.


24. Fautz R, Husein B, Hechenberger C. Application of the neutral red assay (NR assay) to monolayer cultures of primary hepatocytes: rapid colorimetric viability determination for the unscheduled DNA synthesis test (UDS). Mutat Res. 1991. 253:173–179.


25. D'Agostino P, Ferlazzo V, Milano S, La Rosa M, Di Bella G, Caruso R, Barbera C, Grimaudo S, Tolomeo M, Feo S, Cillari E. Anti-inflammatory effects of chemically modified tetracyclines by the inhibition of nitric oxide and interleukin-12 synthesis in J774 cell line. Int Immunopharmacol. 2001. 1:1765–1776.
26. Dignam JD, Lebovitz RM, Roeder RG. Accurate transcription initiation by RNA polymerase II in a soluble extract from isolated mammalian nuclei. Nucleic Acids Res. 1983. 11:1475–1489.


27. Bingham SA, Atkinson C, Liggins J, Bluck L, Coward A. Phyto-oestrogens: where are we now? Br J Nutr. 1998. 79:393–406.


28. Weber C, Negrescu E, Erl W, Pietsch A, Frankenberger M, Ziegler-Heitbrock HW, Siess W, Weber PC. Inhibitors of protein tyrosine kinase suppress TNF-stimulated induction of endothelial cell adhesion molecules. J Immunol. 1995. 155:445–451.
29. Libby P, Galis ZS. Cytokines regulate genes involved in atherogenesis. Ann N Y Acad Sci. 1994. 748:158–168.
30. Chen H, Chen Y, Tian W, Lei S, Peng R. Effects of estradiol and isoflavoid on the expression of adhesion molecules on neutrophils. Hua Xi Yi Ke Da Xue Xue Bao. 2001. 32:27–31.
31. Lund CO, Mortensen A, Nilas L, Breinholt VM, Larsen JJ, Ottesen B. Estrogen and phytoestrogens: Effect on eNOS expression and in vitro vasodilation in cerebral arteries in ovariectomized Watanabe heritable hyperlipidemic rabbits. Eur J Obstet Gynecol Reprod Biol. 2007. 130:84–92.


32. Woodman OL, Missen MA, Boujaoude M. Daidzein and 17 beta-estradiol enhance nitric oxide synthase activity associated with an increase in calmodulin and a decrease in caveolin-1. J Cardiovasc Pharmacol. 2004. 44:155–163.


33. Kim DS, Kwon HM, Choi JS, Kang SW, Ji GE, Kang YH. Resveratrol blunts tumor necrosis factor-α-induced monocyte adhesion and transmigration. Nutr Res Pract. 2007. 1:285–290.


34. Guo JM, Xiao BX, Liu DH, Grant M, Zhang S, Lai YF, Guo YB, Liu Q. Biphasic effect of daidzein on cell growth of human colon cancer cells. Food Chem Toxicol. 2004. 42:1641–1646.

