Abstract
The dietary intake of whole grains is known to reduce the incidence of chronic diseases such as obesity, diabetes, cardiovascular disease, and cancer. To investigate whether there are anti-adipogenic activities in various Korean cereals, we assessed water extracts of nine cereals. The results showed that treatment of 3T3-L1 adipocytes with Sorghum bicolor L. Moench, Setaria italica Beauvois, or Panicum miliaceum L. extract significantly inhibited adipocyte differentiation, as determined by measuring oil red-O staining, triglyceride accumulation, and glycerol 3-phosphate dehydrogenase activity. Among the nine cereals, P. miliaceum L. showed the highest anti-adipogenic activity. The effects of P. miliaceum L. on mRNA expression of peroxisome proliferator-activated receptor-γ, sterol regulatory element-binding protein 1, and the CCAAT/enhancer binding protein-α were evaluated, revealing that the extract significantly decreased the expression of these genes in a dose-dependent manner. Moreover, P. miliaceum L. extract changed the ratio of monounsaturated fatty acids to saturated fatty acids in adipocytes, which is related to biological activity and cell characteristics. These results suggest that some cereals efficiently suppress adipogenesis in 3T3-L1 adipocytes. In particular, the effect of P. miliaceum L. on adipocyte differentiation is associated with the downregulation of adipogenic genes and fatty acid accumulation in adipocytes.
Obesity is a major contributor to various chronic diseases, such as type 2 diabetes, hypertension, cardiovascular disease, and certain types of cancer. Diet is closely related to the development of obesity, although genetic factors including gene mutations also regulate its development [1]. Several epidemiological studies have clearly shown that dietary whole grains are associated with lower body mass index and a reduced risk of being overweight [2-4]. However, the effects of cereals on the cellular and molecular mechanisms responsible for differentiation of adipocytes have not been reported.
Mouse fibroblast 3T3-L1 cells are well-established and commonly used as an in vitro model for assessing adipocyte differentiation [5]. During adipocyte differentiation, transcriptional factors such as peroxisome proliferator-activated receptor-γ (PPARγ), sterol regulatory element-binding protein1 (SREBP1), and CCAAT/enhancer binding protein-α (C/EBPα) promote the expression of adipocyte-specific genes for adipogenesis and to maintain differentiated states [6]. There is evidence that saturated fatty acid (SFA) intake is positively correlated with obesity [7,8]. However, monounsaturated fatty acid (MUFA) intake reduces the impact of a high fat diet on obesity [9,10]. It has also been suggested that fatty acids and their composition are related to the biological characteristics of adipocytes.
To investigate whether cereals are capable of anti-adipogenic activities, we examined water extracts of nine cereals using 3T3-L1 cell differentiation assays. Additionally, the expressions of adipogenic transcriptional factors (PPARγ, SREBP1, and C/EBPα) as well as fatty acid accumulation amounts were evaluated, as these are key regulators of adipocyte differentiation.
Dulbecco's modified Eagles medium (DMEM), bovine calf serum (BCS), antibiotic-anti-mycotic solution, and trypsin-EDTA were purchased from Gibco Co. (Grand Island, NY, USA). Dexamethasone (DEX), 3-isobutyl-1-methylxanthine (IBMX), insulin, and oil red O were purchased from Sigma-Aldrich (St. Louis, MO, USA). Fatty acid methyl esters (FAME) standards were purchased from Supelco (Bellefonte, PA, USA). All solvents and reagents used were of analytical grade. Boron trifluoride methanol solution (14% BF3), sodium hydroxide, and sodium chloride were purchased from Sigma-Aldrich (St. Louis, MO, USA). Chloroform, normal hexane, and methanol were purchased from J. T. Baker (Phillipsburg, NJ, USA).
All cereals were obtained from the National Agricultural Cooperative Federation (Shinlim, Wonju, Korea) and ground into a fine powder. The powdered material (50 g) was ultrasonically extracted with 500 ml of 80℃ water twice for 3 hr each time at room temperature and concentrated at 80℃ in a rotatory vacuum evaporator (EYELA N-1000, Riakikai Co., Ltd., Tokyo, Japan). The extracts were then freeze-dried and stored at -70℃. The common names and extract yields of the cereals are listed in Table 1.
Mouse 3T3-L1 fibroblast cells, obtained from the Korean Cell Line Bank (Seoul, Korea), were cultured in six-well tissue culture plates. The cells were maintained in DMEM supplemented with 10% heat-inactivated BCS, 100 units/ml of penicillin, and 100 µg/ml of streptomycin. One day after confluence (designated as day 0), cell differentiation was induced by adipogenic agents (0.5 mM IBMX, 2 µM DEX, and 0.7 µM insulin), which were added to the culture medium [11]. Cells were randomly treated with each cereal sample at 10 µg/ml concentrations (n = 4) in different plates upon initiation of differentiation and with every medium change for 8 days.
After 8 days of adipogenic differentiation, cultured adipocytes that had reached confluence were fixed with 3.7% formalin for 1 hr, and stained with oil red O (three parts 0.5% oil red O dye in isopropanol to two parts water) for 40 min. The stained cells were washed three times with water. The oil red O was then eluted with DMSO and quantified by measuring optical density (OD) at 510 nm.
3T3-L1 adipocytes were harvested 8 days after initiating differentiation. The cells were washed twice with cold PBS, collected, and lysed in a lysis buffer (1% Triton X-100 in PBS). Total TG content in the cells was determined using a TG kit (Wako Chemical, Osaka, Japan). The protein concentration was determined with a BioRed DC Protein Assay kit (Bio-Rad Laboratories, Hercules, CA, USA). Inhibition was expressed as the percent (%) decrease in TG content against a control (0%).
3T3-L1 adipocytes were harvested 8 days after initiating differentiation. The cells were washed twice with cold PBS, scraped into 200 µl of an enzyme extract buffer (provided with the kit), and sonicated. GPDH activity was determined using a GPDH kit (Takara Bio, Shiga, Japan), according to the manufacturer's protocol.
To determine the time-course effect of P. miliaceum L. on adipocyte differentiation-related gene expression, cells were harvested at 2, 4, 6, and 8 days after initiating differentiation. Total RNA was isolated from 3T3-L1 cells using Trizol reagent (Invitrogen, Carlsbad, CA, USA), following the manufacturer's recommendations. Real-time quantitative PCR was performed with a SYBR Green™ kit (Quantitect™ SYBR Green PCR, Qiagen, Valencia, CA, USA). The cycling conditions were 15 min at 95℃, 40 cycles of 15 s at 94℃, 30 s at 51℃, and 30 s at 72℃. Relative quantification was determined using the delta-delta method [12]. The primer sequences of the genes examined are shown in Table 2.
Cellular lipids were extracted using a previously reported procedure [13]. The harvested cells were washed with 4 ml isopropanol, and 4 ml hexane was added. The cells were crushed using an ultrasonic reactor for 10 min, and 4 ml water was added to the mixture. The tubes were centrifuged at 2,000 × g at 5℃ for 5 min, and the upper layer was transferred to a new tube. After the hexane was evaporated under nitrogen in a 38℃ water bath, the residue was stored at -80℃ for further analysis.
A combined base/acid methylation method [14] with modifications was used. The methyl ester of TFA was obtained by a reaction in a Techne DB-3D block heater (Barloworld Scientific US Ltd., Burlington, NJ, USA), with BF3 as the catalyst. A portion of a 20 mg oil sample was boiled with 2 mL methanolic sodium hydroxide (0.5 M) at 100℃ for 5 min on a heated block. BF3 reagent (2 mL) was added and boiled for an additional 5 min. One mL of hexane and 2 mL of saturated sodium chloride solution were added and vortexed for 1 min. The hexane layer was transferred to a vial for subsequent gas chromatography analysis. FAME were determined by gas chromatography (Agilent 6890N GC; Agilent Technologies, Santa Clara, CA, USA) equipped with flame ionization detector (FID) and a HP-FFAP capillary column (30 m × 0.32 mm I.D. × 0.25 µm film thickness; Agilent Technologies). The injector temperature was 230℃ with a split ratio of 10:1, and the FID temperature was 250℃. The oven temperature program was 100℃ for 1 min, 100-230℃ at a rate of 3℃ per minute, and 230℃ for 10 min. Helium was used as the carrier gas at a constant flow rate of 1.0 mL/min with an injection volume of 1 µL. Hydrogen flow to the detector was 40 mL/min, air flow was 300 mL/min, and the helium make-up gas flow rate was 20 mL/min.
We tested the water extracts of nine cereals using 3T3-L1 cell assays to investigate whether anti-adipogenic activity was present in the cereals. The effects of the nine water cereal extracts on the accumulation of intracellular lipid droplets in 3T3-L1 adipocytes are shown in Fig. 1. The OD values of oil red O decreased from 87.4%, to 76.7%, and 76.4% in the S. bicolor L. Moench, S. italica Beauvois, and P. miliaceum L. groups, respectively. We also examined cellular TG accumulation. As shown Fig. 1B, treatment with the S. italica Beauvois and P. miliaceum L. samples reduced TG content of the differentiated adipocytes from 78.6% to 65.7% compared to that in control cells.
GPDH activity decreased from 81.34%, to 81.26%, and 69.57% in the S. bicolor L. Moench, S. italica Beauvois, and P. miliaceum L. groups, respectively (Fig. 2). P. miliaceum L. decreased GPDH activity most effectively among the nine cereal extracts.
Among the nine samples, P. miliaceum L. had the most effect on oil red O staining, TG content, and GPDH activity in 3T3-L1 adipocytes. To gain a better understanding of the molecular mechanism(s) underlying this anti-adipogenic effect, we examined the effect of P. miliaceum L. on gene expression of key adipogenic regulators. Moreover, to examine whether a lower concentration of P. miliaceum L. could alter gene expression, cells were treated with P. miliaceum L. at concentrations of 0, 1, or 10 µg/ml for 8 days during differentiation. Consistent with the decrease in TG accumulation and GPDH activity, P. miliaceum L. significantly decreased PPARγ and SREBP1 mRNA expression in a time- and dose-dependent manner compared to that in differentiated adipocytes (Fig. 3A and 3C). A lower concentration of P. miliaceum L. (1 µg/ml) did not significantly affect C/EBPα gene expression compared to that in differentiated adipocytes (Fig. 3C).
The total MUFA to SFA ratio as well as the individual ratio of C16:1/C16:0 and C18:1/C18:0 in adipocytes treated with P. miliaceum L. (1 and 10 µg/ml) were significantly higher compared to those in the control group (P < 0.05, Table 3). C16:1 fatty acid was not detected in differentiated 3T3-L1 cells (control group). Treatment with 10 µg/ml P. miliaceum L. significantly increased the ratios of C18:1/C18:0 and C16:1/C16:0 by 413.1% and 460.0% compared to those in control cells (P < 0.05).
There is abundant evidence for the beneficial effects of consuming whole grains on the risk for chronic diseases that are closely related to obesity. However, such evidence does not fully explain the mechanisms for preventing and managing obesity. We examined obesity-related markers in 3T3-L1 cells to investigate the anti-adipogenic efficacy of several cereals cultivated in Korea. In our preliminary oil red O staining assay, no significant difference was observed at 1 µg/ml for all of the cereals. We could not prepare samples at 100 µg/ml due to grain viscosity. Thus, 3T3-L1 cells were treated with samples at 10 µg/ml concentration. The results showed that some of the cereals, specifically S. bicolor L. Moench, S. italica Beauvois, and P. miliaceum L., had anti-adipogenic activity as measured by oil red O staining as well as TG content and GPDH activity. Oil red O staining and TG content are indicators of lipid accumulation. GPDH, a cytosolic enzyme, is the predominant substrate for TG synthesis in adipose tissue and is linked to the characteristic changes that occur during the conversion process [15]. These results indicate that the cereal samples inhibited lipid accumulation during adipocyte differentiation, and that this, at least in part, was mediated by a reduction in GPDH activity. P. miliaceum L. had the highest anti-adipogenic activity.
To gain a better understanding of the molecular mechanism(s) underlying this effect, we examined the effect of P. miliaceum L. on gene expression of key adipogenic regulators. Previous studies have shown that PPARγ and C/EBPα cross-regulate each other to maintain gene expression and that they regulate the expression of other adipogenic genes during adipogenic differentiation [16,17]. We found that PPARγ and C/EBPα mRNA expression increased at 4 days and that P. miliaceum L. effectively inhibited mRNA expression (Fig. 3). Several factors are participating in the underlying PPARγ and C/EBPα mechanism. Wnt signaling is known to block the PPARγ and C/EBPα expression, and β-catenin is commonly thought to function as a Wnt effector [18]. Phosphatidylinositol-3 kinase and AKT1/protein kinase B also facilitate PPARγ and C/EBPα expression [19]. P. miliaceum L. inhibited PPARγ mRNA expression more effectively than it did C/EBPα mRNA expression. P. miliaceum L. may regulate PPARγ mRNA expression by modulating C/EBPα and other factors. Oishi et al. [20] revealed that the Krüppel-like factor (KFL) family, particularly KFL5, is induced early during adipocyte differentiation and that it directly activates PPARγ expression.
SREBP1 has a role regulating genes associated with fatty acid synthesis, and its overexpression contributes to the generation of PPARγ ligands [21]. The downregulation of C/EBPα and SREBP1 by P. miliaceum L. may reduce fatty acid synthesis as well as PPARγ activity, which may result in the inhibition of adipogenesis by blocking adipocyte differentiation. These results suggest that the anti-adipogenic effect of P. miliaceum L. involves downregulation of both C/EBPα and SREBP1 and subsequent PPARγ downregulation.
Fatty acids play an essential role in cellular metabolism and in the physiological responses of cells by regulating membrane fluidity [22], signal transduction [23], and protein function [24]. MUFA synthesis increases cell membrane fluidity. Collins et al. [25] found that upregulation of desaturation pathways increases MUFA production and that is related to membrane fluidity and insulin sensitivity in human adipocytes. Scaglia and Igal [26] noted that depleting stearoyl-CoA desaturase 1 (SCD1), an enzyme that converts SFA into MUFA, decreased the MUFA/SFA ratio and inhibited incorporation of glucose into cells. Jaureguiberry et al. [27] also recently reported significant increases in intracellular lipid droplets in SCD overexpressing cells, suggesting that changes in the MUFA/SFA ratio are related to cell biological activities and that a high MUFA/SFA ratio is a key regulator for membrane fluidity and the biophysical characteristics of cells. In this study, P. miliaceum L. (1 and 10 µg/ml) significantly increased the MUFA/SFA ratio in adipocytes (Table 3). These changes may influence adipocyte membrane phospholipids, which modulate membrane fluidity and regulate several proteins associated with adipocyte differentiation. Some evidence exists for a significant role of fatty acids in adipocytes. For example, a negative correlation has been noted between GPDH activity and cellular fatty acids, particularly MUFA C16:1 and C18:1 [28]. Another in vitro study also showed that the SFAs laurate, myristate, and palmitate increase cellular TG accumulation in 3T3-L1 cell and SFA act as a ligand for toll-like receptor 4, which is required for PPARγ expression [29]. In our study, reduced TG content and GPDH activity by P. miliaceum L. corresponded with increased C16:1/C16:0, C18:1/C18:0, and MUFA/SFA ratios in adipocytes. Therefore, the anti-adipogenic effect of P. miliaceum L. on adipocytes is associated with cellular MUFA accumulation, and its action may be responsible for the downregulation of PPARγ mRNA expression.
Although the active principles responsible for the anti-adipogenic activities of the tested cereals were not identified, our results suggest that some cereals, particularly P. miliaceum L., provide useful information regarding the further discovery of cereals with chemopreventive effects.
Figures and Tables
Fig. 1
Effects of cereal extracts on adipocyte differentiation in 3T3-L1 cells. Preadipocytes were cultured in media containing different cereal extracts from day 0 to day 6, and lipid accumulation was measured by (A) oil red O staining and (B) TG content. Data are expressed as means ± SDs (n = 4). *P < 0.05 vs. C (untreated control adipocytes). The results represent three independent experiments.
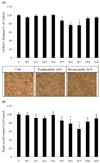
Fig. 2
Effects of cereal extracts on glycerol 3-phosphate dehydrogenase (GPDH) activity in 3T3-L1 cells. Preadipocytes were cultured in media containing different cereal extracts from day 0 to day 6, and GPDH activity was measured. Data are expressed as means ± SDs (n = 4). *P < 0.05 vs. C (untreated control adipocytes). The results represent three independent experiments.
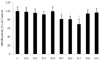
Fig. 3
Effect of the P. miliaceum L. extract on real-time PCR analysis in 3T3-L1 cells. Preadipocytes were cultured in media containing different concentrations of P. miliaceum L. from day 0 to day 6. After 2, 4, and 6 days, peroxisome proliferator-activated receptor-γ (PPARγ), sterol regulatory element-binding protein 1 (SREBP1), and CCAAT/enhancer binding protein-α (C/EBPα) mRNA expression was measured. A, PPARγ; B, C/EBPα; C, SREBP1. Data are expressed as means ± SDs (n = 4). *P < 0.05 vs. 0 µg/ml. The results represent three independent experiments.
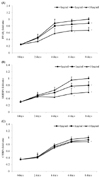
References
1. Barsh GS, Farooqi IS, O'Rahilly S. Genetics of body-weight regulation. Nature. 2000. 404:644–651.


2. Dugee O, Khor GL, Lye MS, Luvsannyam L, Janchiv O, Jamyan B, Esa N. Association of major dietary patterns with obesity risk among Mongolian men and women. Asia Pac J Clin Nutr. 2009. 18:433–440.
3. Misra A, Rastogi K, Joshi SR. Whole grains and health: perspective for Asian Indians. J Assoc Physicians India. 2009. 57:155–162.
4. Williams PG, Grafenauer SJ, O'Shea JE. Cereal grains, legumes, and weight management: a comprehensive review of the scientific evidence. Nutr Rev. 2008. 66:171–182.


5. Cho EJ, Rahman MA, Kim SW, Baek YM, Hwang HJ, Oh JY, Hwang HS, Lee SH, Yun JW. Chitosan oligosaccharides inhibit adipogenesis in 3T3-L1 adipocytes. J Microbiol Biotechnol. 2008. 18:80–87.
6. Rosen ED, MacDougald OA. Adipocyte differentiation from the inside out. Nat Rev Mol Cell Biol. 2006. 7:885–896.


7. van Dijk SJ, Feskens EJ, Bos MB, Hoelen DW, Heijligenberg R, Bromhaar MG, de Groot LC, de Vries JH, Müller M, Afman LA. A saturated fatty acid-rich diet induces an obesity-linked proinflammatory gene expression profile in adipose tissue of subjects at risk of metabolic syndrome. Am J Clin Nutr. 2009. 90:1656–1664.


8. Phillips CM, Goumidi L, Bertrais S, Field MR, Peloso GM, Shen J, McManus R, Hercberg S, Lairon D, Planells R, Roche HM. Dietary saturated fat modulates the association between STAT3 polymorphisms and abdominal obesity in adults. J Nutr. 2009. 139:2011–2017.


9. Bos MB, de Vries JH, Feskens EJ, van Dijk SJ, Hoelen DW, Siebelink E, Heijligenberg R, de Groot LC. Effect of a high monounsaturated fatty acids diet and a Mediterranean diet on serum lipids and insulin sensitivity in adults with mild abdominal obesity. Nutr Metab Cardiovasc Dis. 2010. 20:591–598.


10. Lai CQ, Parnell LD, Arnett DK, García-Bailo B, Tsai MY, Kabagambe EK, Straka RJ, Province MA, An P, Borecki IB, Tucker KL, Ordovás JM. WDTC1, the ortholog of Drosophila adipose gene, associates with human obesity, modulated by MUFA intake. Obesity (Silver Spring). 2009. 17:593–600.


11. Choi BH, Kim YH, Ahn IS, Ha JH, Byun JM, Do MS. The inhibition of inflammatory molecule expression on 3T3-L1 adipocytes by berberine is not mediated by leptin signaling. Nutr Res Pract. 2009. 3:84–88.


12. Livak KJ, Schmittgen TD. Analysis of relative gene expression data using real-time quantitative PCR and the 2(-Delta Delta C(T)) Method. Methods. 2001. 25:402–408.


13. He ML, Hnin TM, Kuwayama H, Mir PS, Okine EK, Hidari H. Effect of conjugated linoleic acid type, treatment period, and dosage on differentiation of 3T3 cells. Lipids. 2006. 41:937–949.


14. Kramer JK, Fellner V, Dugan ME, Sauer FD, Mossoba MM, Yurawecz MP. Evaluating acid and base catalysts in the methylation of milk and rumen fatty acids with special emphasis on conjugated dienes and total trans fatty acids. Lipids. 1997. 32:1219–1228.


15. Kozak LP, Jensen JT. Genetic and developmental control of multiple forms of L-glycerol 3-phosphate dehydrogenase. J Biol Chem. 1974. 249:7775–7781.


16. Gregoire FM, Smas CM, Sul HS. Understanding adipocyte differentiation. Physiol Rev. 1998. 78:783–809.


17. Rosen ED, Spiegelman BM. Molecular regulation of adipogenesis. Annu Rev Cell Dev Biol. 2000. 16:145–171.


18. Ross SE, Hemati N, Longo KA, Bennett CN, Lucas PC, Erickson RL, MacDougald OA. Inhibition of adipogenesis by Wnt signaling. Science. 2000. 289:950–953.


19. Tseng YH, Kriauciunas KM, Kokkotou E, Kahn CR. Differential roles of insulin receptor substrates in brown adipocyte differentiation. Mol Cell Biol. 2004. 24:1918–1929.


20. Oishi Y, Manabe I, Tobe K, Tsushima K, Shindo T, Fujiu K, Nishimura G, Maemura K, Yamauchi T, Kubota N, Suzuki R, Kitamura T, Akira S, Kadowaki T, Nagai R. Krüppel-like transcription factor KLF5 is a key regulator of adipocyte differentiation. Cell Metab. 2005. 1:27–39.


21. Kim JB, Wright HM, Wright M, Spiegelman BM. ADD1/SREBP1 activates PPARγ through the production of endogenous ligand. Proc Natl Acad Sci U S A. 1998. 95:4333–4337.


22. Siener R, Alteheld B, Terjung B, Junghans B, Bitterlich N, Stehle P, Metzner C. Change in the fatty acid pattern of erythrocyte membrane phospholipids after oral supplementation of specific fatty acids in patients with gastrointestinal diseases. Eur J Clin Nutr. 2010. 64:410–418.


23. Koenitzer JR, Freeman BA. Redox signaling in inflammation: interactions of endogenous electrophiles and mitochondria in cardiovascular disease. Ann N Y Acad Sci. 2010. 1203:45–52.


24. Søgaard R, Werge TM, Bertelsen C, Lundbye C, Madsen KL, Nielsen CH, Lundbaek JA. GABAA receptor function is regulated by lipid bilayer elasticity. Biochemistry. 2006. 45:13118–13129.


25. Collins JM, Neville MJ, Hoppa MB, Frayn KN. De novo lipogenesis and stearoyl-CoA desaturase are coordinately regulated in the human adipocyte and protect against palmitate-induced cell injury. J Biol Chem. 2010. 285:6044–6052.


26. Scaglia N, Igal RA. Inhibition of Stearoyl-CoA Desaturase 1 expression in human lung adenocarcinoma cells impairs tumorigenesis. Int J Oncol. 2008. 33:839–850.


27. Jaureguiberry MS, Tricerri MA, Sanchez SA, Garda HA, Finarelli GS, Gonzalez MC, Rimoldi OJ. Membrane organization and regulation of cellular cholesterol homeostasis. J Membr Biol. 2010. 234:183–194.


28. He ML, Wang Y, You JS, Mir PS, McAllister TA. Effect of a seaweed extract on fatty acid accumulation and glycerol-3-phosphate dehydrogenase activity in 3T3-L1 adipocytes. Lipids. 2009. 44:125–132.


29. Yeop Han C, Kargi AY, Omer M, Chan CK, Wabitsch M, O'Brien KD, Wight TN, Chait A. Differential effect of saturated and unsaturated free fatty acids on the generation of monocyte adhesion and chemotactic factors by adipocytes: dissociation of adipocyte hypertrophy from inflammation. Diabetes. 2010. 59:386–396.