Abstract
We investigated the effect of paternal folate status on folate content and expression of the folate transporter folate receptor α (FRα) in rat placental tissues. Rats were mated after males were fed a diet containing 0 mg of folic acid/kg of diet (paternal folate-deficient, PD) or 8 mg folic acid/kg of diet (paternal folate-supplemented, PS) for 4 weeks. At 20 days of gestation, the litter size, placental weight, and fetal weight were measured, and placental folate content (n = 8/group) and expression of FRα (n = 10/group) were analyzed by microbiological assay and Western blot analysis, respectively. Although there was no difference observed in litter size or fetal weight, but significant reduction (10%) in the weight of the placenta was observed in the PD group compared to that in the PS group. In the PD group, placental folate content was significantly lower (by 35%), whereas FRα expression was higher (by 130%) compared to the PS group. Our results suggest that paternal folate status plays a critical role in regulating placental folate metabolism and transport.
The placenta, a tissue of fetal origin, plays distinct roles in the maternal-fetal transfer of oxygen and nutrients as well as the synthesis of hormones and various proteins [1]. Proper functioning of the placenta, therefore, is important for normal fetal growth and development. Restrictions of nutrient intake during the critical period of rapid placental growth may restrict the degree of growth and development of the placenta, leading to low birth weight [2].
Folate plays a role in various one-carbon transfer reactions and is important for normal placental and fetal growth and development [3]. Placental folate transporters play a critical role in mediating placental transport of maternal folate to the fetus [4]. They act by transferring 5-methyltetrahydrofolate against a concentration gradient, achieving about a 3-fold higher folate concentration in the fetal circulation than in the maternal plasma [3]. Among several transporters, placental folate receptor α (FRα) is responsible for the initial step in placental folate transfer by mediating apical folate uptake at physiological pH as well as the entry of folate from maternal blood into the syncytiotrophoblast [5-8]. Maternal folate deficiency causes changes in placental folate content, expression of folate transporters, and global DNA methylation [8-11], and is known to result in placental complications such as placental abruption, vascular thrombosis, and infarction, as well as adverse birth outcomes [12].
The placenta has recently received a great deal of attention due to its importance in imprinting and epigenetic regulation during the development of offspring [13]. Research on the association of parental folate status with placental folate metabolism and birth outcome has mainly focused on maternal folate status. The association of paternal folate status to reproduction has largely been limited to sperm quality [14-16]. In our preliminary study, lower contents of brain folate in fetuses of folate-supplemented dams mated with folate-deficient males were observed compared to those of folate-supplemented male rats [17]. We therefore hypothesized that paternal folate deficiency influences placental folate transfer, and in turn, resulting in unfavorable fetal outcomes similar to maternal folate deficiency. In the study presented here, we investigated the effect of paternal folate status on placental folate content and FRα expression.
Six-week-old male Sprague-Dawley rats (n = 14) and female rats (n = 14) were purchased from Central Lab., Animal Inc. (Seoul, Korea) and fed a non-purified diet (Central Lab, Animal Inc.) during a one-week acclimation period. After this, male rats were randomly divided into two groups and fed ad libitum casein-based AIN-93G diets (Central Lab, Animal Inc.) containing 0 mg of folic acid [pteroylglutamic acid]/kg of diet (paternal folate-deficient group, PD) or 8.0 mg of folic acid/kg of diet (paternal folate-supplemented group, PS) for 4 weeks [11]. In contrast, all female rats were fed a diet containing 8.0 mg of folic acid/kg of diet for 4 weeks before mating and thereafter. Body weight and food intake were monitored throughout the experimental period once and thrice per week, respectively. On day 20 of gestation, rats were killed by exsanguination under ether anesthesia, and litter size, placental weight, and fetal weight were measured. The isolated fetal liver and placenta were stored at -80℃ until analyses. This study was approved by the Institutional Animal Care and Use Committee of Ewha Womans University.
Folate content was measured with Lactobacillus rhamnosus (ATCC 7469) in a 96-well microplate using randomly selected fetal liver (n = 10) and placental tissues (n = 8) [18]. After washing with phosphate-buffered saline (pH 7.0), the whole placenta was homogenized with 0.1 M potassium phosphate buffer containing ascorbic acid (58.8 mmol/l) and centrifuged (240 × g for 10 min, 4℃). The supernatant was incubated with rat serum folate conjugase (8 h, 37℃, pH 7.0) to hydrolyze polyglutamyl folates to monoglutamyl folates, which are readily utilized by the bacteria. After heating (10 min, 100℃) and subsequent centrifugation (2,450 × g, 10 min), the supernatant was directly used for folate assay.
Randomly selected whole placental tissues (n = 10) were homogenized in RIPA Cell Lysis Buffer (pH 7.5, GenDepot, Barker, TX, USA) with ProteoBlock Protease Inhibitor Cocktail (Fermentas, Burlington, Canada) and centrifuged (16,600 × g for 15 min, 4℃). The protein content of the supernatant was determined using a bicinchoninic acid (BCA) Protein Assay Kit (Thermo Scientific, Rockford, IL, USA). Then, 15 µg of protein was applied onto 10% SDS-polyacrylamide gel and electro-transferred onto a nitrocellulose transfer membrane (Whatman, Dassel, Germany), which was probed with primary antibodies against either FRα or β-actin (Santa Cruz Biotechnology, Santa Cruz, CA, USA); horseradish-peroxidase-linked antibody was used as a secondary antibody (Santa Cruz Biotechnology). The blotted membranes were visualized with SuperSignal West Pico Chemiluminescent Substrate (Thermo Scientific), and the density of each band was quantified using LAS-3000 and MultiGauge V3.0 densitometer (Fujifilm, Tokyo, Japan).
The mean body weight and food intake of male rats during mating and female rats on day 20 of gestation did not differ significantly between the PS and PD groups (Table 1). There were no differences in litter size between the two groups (14.1 ± 1.01 and 13.3 ± 0.75 in the PS and PD groups, respectively). The mean placental weight in the PD group (0.36 ± 0.01 g) was lower than that in the PS group (0.40 ± 0.01 g, P < 0.05, Fig. 1B). On the other hand, no difference was observed in mean fetal weight between the two groups (PS; 1.37 ± 0.01 g and PD; 1.35 ± 0.02 g, P = 0.38, Fig. 1A).
The mean fetal liver folate content of the PD group (30.70 ± 2.70 nmol/g of wet tissue) was significantly lower than that of the PS group (46.17 ± 5.73 nmol/g of wet tissue, P < 0.05, Fig. 2A), and the mean placental folate content of the PD group (5.03 ± 0.57 nmol/g of wet tissue) was significantly lower than that of the PS group (7.70 ± 0.36 nmol/g of wet tissue, P < 0.01, Fig. 2B). The placental FRα expression of the PD group was about 2.3 times higher than that of the PS group (P < 0.01, Fig. 2C).
We found that mean placental weight was lower in the PD group than in the PS group, although fetal weights were similar between the two groups. We also found that paternal folate deficiency affected folate transport in the placenta. Folate deficiency also reduced the folate content with an increase in the expression of FRα in the placenta, and we do not know the mechanism of these seemingly contradictory findings. The placenta is a tissue of fetal origin. Therefore, both paternal and maternal factors (genetic and nutritional) should play equally critical roles in regulating placental development and other functions, including folate metabolism and transport. Although such regulations can be controlled by epigenetic regulation of genes from both parents, little attention has been given to paternal factors. In our study, paternal folate deficiency alone, despite the maternal folate sufficiency, caused a decrease in fetal liver and placental folate contents along with FRα upregulation and a decrease in placental weight. These results clearly suggest that paternal folate status was critical for placental folate metabolism and development, and this effect was independent from the effect of maternal folate status. To our knowledge, this is the first study to demonstrate the effect of paternal folate status on placental folate content and FRα expression.
Fetal growth has been positively associated with maternal folate nutrition in animal and human studies [19-22]. In some experiments, maternal folate intake was found to be associated with fetal weight in a dose-dependent manner [23,24]. As an explanation of such findings, it was demonstrated that maternal deficiency is associated with folate receptor upregulation accompanied by multiple aberrations in fetal tissues as well as severe placental defects in mice [24]. It was also reported that placental weights are not affected by maternal folate deficiency despite markedly low placental folate content. In our study, however, a higher mean placental weight was found in the PS than PD group, whereas no difference in the fetal weight was observed. We do not know the reasons for these discrepancies with other investigations.
At this point, we are unable to offer underlying mechanisms for our observed changes in the folate content, FRα expression, and placental weight caused by paternal folate status. However, the following explanation may be plausible: changes in paternal folate status could affect sperm DNA methylation, which would disrupt gene expression. We and several other investigators have reported reduced levels of global [11,25] and gene-specific DNA methylation [26] in the placenta during maternal folate deficiency as well as in sperm in folate-deficient males with genetic polymorphisms [27,28]. Although we did not measure DNA methylation in the placenta in this study, fetuses in the PD group may have been affected by folate-deficiency-induced paternal epigenetic modification in addition to sperm DNA alteration, such as DNA hypomethylation during spermatogenesis [29,30]. We speculate that the reduction in placental weight in the PD group was due to epigenetic changes in sperm DNA, or imprinting of genes, and possibly in the placental DNA as well. Although some alleles are expressed from only one of the parent-derived ones affecting genomic imprinting, both parents can provide DNA sequences to the fertilized egg at the time of fertilization. Therefore, it is possible that paternal folate deficiencies cause epigenetic changes in genes, including the FRα gene, thus altering the expression of FRα in the placenta and liver as well.
The weakness of our present study is that it is difficult to explain the physiological significance of our data or even a mechanistic explanation of our findings. Further determinations of folate contents and the expression of other folate transporters, as well as folate distribution in maternal and fetal tissues, may elucidate these issues. Furthermore, studies should investigate whether or not the changes in other placental transporters are caused by paternal folate deficiency in combination with maternal folate sufficiency or deficiency in this animal model. The role of the placenta has recently received great attention because of its importance in imprinting and epigenetic regulation during the development of offspring [13].
Figures and Tables
Fig. 1
Fetal weights and placental weights in the paternal folate-supplemented and folate-deficient groups. (A) Fetal weight and (B) placental weight. The vertical lines represent the SEM. Numbers below the group names represent the number of animals. P values represent the significance of the differences among the groups (Student's t-test).
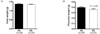
Fig. 2
Fetal liver folate contents, placental folate contents, and protein expression levels of FRα in the paternal folate-supplemented and folate-deficient groups. (A) Fetal liver folate content, (B) placental folate content, and (C) expression of FRα in the placenta. The vertical lines represent the SEM. P values represent the significance of the differences among the groups (Student's t-test).
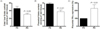
References
1. Belkacemi L, Nelson DM, Desai M, Ross MG. Maternal undernutrition influences placental-fetal development. Biol Reprod. 2010. 83:325–331.
2. Wallace JM, Luther JS, Milne JS, Aitken RP, Redmer DA, Reynolds LP, Hay WW Jr. Nutritional modulation of adolescent pregnancy outcome - a review. Placenta. 2006. 27:Suppl A. S61–S68.


4. Henderson GI, Perez T, Schenker S, Mackins J, Antony AC. Maternal-to-fetal transfer of 5-methyltetrahydrofolate by the perfused human placental cotyledon: evidence for a concentrative role by placental folate receptors in fetal folate delivery. J Lab Clin Med. 1995. 126:184–203.
5. Bisseling TM, Steegers EA, van den Heuvel JJ, Siero HL, van de Water FM, Walker AJ, Steegers-Theunissen RP, Smits P, Russel FG. Placental folate transport and binding are not impaired in pregnancies complicated by fetal growth restriction. Placenta. 2004. 25:588–593.


6. Luhrs CA, Slomiany BL. A human membrane-associated folate binding protein is anchored by a glycosyl-phosphatidylinositol tail. J Biol Chem. 1989. 264:21446–21449.


7. Verma RS, Gullapalli S, Antony AC. Evidence that the hydrophobicity of isolated, in situ, and de novo-synthesized native human placental folate receptors is a function of glycosyl-phosphatidylinositol anchoring to membranes. J Biol Chem. 1992. 267:4119–4127.


9. Baker H, Frank O, Deangelis B, Feingold S, Kaminetzky HA. Role of placenta in maternal-fetal vitamin transfer in humans. Am J Obstet Gynecol. 1981. 141:792–796.


10. Solanky N, Requena Jimenez A, D'Souza SW, Sibley CP, Glazier JD. Expression of folate transporters in human placenta and implications for homocysteine metabolism. Placenta. 2010. 31:134–143.


11. Kim JM, Hong K, Lee JH, Lee S, Chang N. Effect of folate deficiency on placental DNA methylation in hyperhomocysteinemic rats. J Nutr Biochem. 2009. 20:172–176.


12. Ray JG, Laskin CA. Folic acid and homocyst(e)ine metabolic defects and the risk of placental abruption, pre-eclampsia and spontaneous pregnancy loss: A systematic review. Placenta. 1999. 20:519–529.


13. Fowden AL, Coan PM, Angiolini E, Burton GJ, Constancia M. Imprinted genes and the epigenetic regulation of placental phenotype. Prog Biophys Mol Biol. 2010. 11. 23. [Epub ahead of print].


14. Wallock LM, Tamura T, Mayr CA, Johnston KE, Ames BN, Jacob RA. Low seminal plasma folate concentrations are associated with low sperm density and count in male smokers and nonsmokers. Fertil Steril. 2001. 75:252–259.


15. Young SS, Eskenazi B, Marchetti FM, Block G, Wyrobek AJ. The association of folate, zinc and antioxidant intake with sperm aneuploidy in healthy non-smoking men. Hum Reprod. 2008. 23:1014–1022.


16. Boxmeer JC, Smit M, Utomo E, Romijn JC, Eijkemans MJ, Lindemans J, Laven JS, Macklon NS, Steegers EA, Steegers-Theunissen RP. Low folate in seminal plasma is associated with increased sperm DNA damage. Fertil Steril. 2009. 92:548–556.


17. Kim HW, Lee YA, Kim HH, Lee S, Chang N. Paternal folate deficiency during reproduction influences folate concentrations in the brain of the offspring. FASEB J. 2010. 24:915.7.


18. Tamura T. Picciano MF, Stokstad ELR, Gregory JF, editors. Microbiological assay of folates. Folic Acid Metabolism in Health and Disease. 1990. New York: Wiley-Liss;121–137.
19. Mooij PN, Thomas CM, Doesburg WH, Eskes TK. The effects of periconceptional folic acid and vitamin supplementation on maternal folate levels and on neurulating hamster embryos in vivo. Int J Vitam Nutr Res. 1993. 63:212–216.
20. Tamura T, Goldenberg RL, Freeberg LE, Cliver SP, Cutter GR, Hoffman HJ. Maternal serum folate and zinc concentrations and their relationships to pregnancy outcome. Am J Clin Nutr. 1992. 56:365–370.


21. Timmermans S, Jaddoe VW, Hofman A, Steegers-Theunissen RP, Steegers EA. Periconception folic acid supplementation, fetal growth and the risks of low birth weight and preterm birth: the Generation R Study. Br J Nutr. 2009. 102:777–785.


22. Lindzon G, O'Connor DL. Folate during reproduction: the Canadian experience with folic acid for fortification. Nutr Res Pract. 2007. 1:163–174.


23. van Eijsden M, Smits LJ, van der Wal MF, Bonsel GJ. Association between short interpregnancy intervals and term birth weight: the role of folate depletion. Am J Clin Nutr. 2008. 88:147–153.


24. Xiao S, Hansen DK, Horsley ET, Tang YS, Khan RA, Stabler SP, Jayaram HN, Antony AC. Maternal folate deficiency results in selective upregulation of folate receptors and heterogeneous nuclear ribonucleoprotein-E1 associated with multiple subtle aberrations in fetal tissues. Birth Defects Res A Clin Mol Teratol. 2005. 73:6–28.


25. Engeham SF, Haase A, Langley-Evans SC. Supplementation of a maternal low-protein diet in rat pregnancy with folic acid ameliorates programming effects upon feeding behaviour in the absence of disturbances to the methionine-homocysteine cycle. Br J Nutr. 2010. 103:996–1007.


26. Chan D, Cushnie DW, Neaga OR, Lawrance AK, Rozen R, Trasler JM. Strain-specific defects in testicular development and sperm epigenetic patterns in 5,10-methylenetetrahydrofolate reductase-deficient mice. Endocrinology. 2010. 151:3363–3373.


27. Dhillon VS, Shahid M, Husain SA. Associations of MTHFR DNMT3b 4977 bp deletion in mtDNA and GSTM1 deletion, and aberrant CpG island hypermethylation of GSTM1 in non-obstructive infertility in Indian men. Mol Hum Reprod. 2007. 13:213–222.


28. Park BH, Kim YJ, Park JS, Lee HY, Ha EH, Min JW, Park HS. Folate and homocysteine levels during pregnancy affect DNA methylation in human placenta. J Prev Med Public Health. 2005. 38:437–442.
29. Jenkins TG, Carrell DT. The paternal epigenome and embryogenesis: poising mechanisms for development. Asian J Androl. 2011. 13:76–80.


30. Nafee TM, Farrell WE, Carroll WD, Fryer AA, Ismail KMK. Epigenetic control of fetal gene expression. BJOG. 2008. 115:158–168.