Abstract
The resistant dextrin NUTRIOSE®, developed from starch, is expected to act as a prebiotic. The aim of this study was to determine the effects of NUTRIOSE® on cecal parameters, short-chain fatty acid (SCFA) concentrations, and fecal excretion in rats. In an initial experiment, twenty-four male Fischer F344 rats were randomly assigned to one of the following four treatments for 14 days: G0 (control diet), G2.5 (control diet + 2.5% of dextrin), G5 (control diet + 5% of dextrin), and G10 (control diet + 10% of dextrin). After 14 days, total cecal weight, cecal content, and cecal wall weight were significantly increased in G5 and G10 compared to G0. At the same time, cecal pH was significantly lower in G10 compared to G0. Total SCFA concentration was significantly higher in G10 than in G5, G2.5, and G0, and significantly higher in G5 than in G0. Acetate, butyrate, and propionate concentrations were significantly increased in G5 and G10 compared to the controls. In a second trial based on a similar design, eighteen male Fischer F344 rats were treated with a control diet supplemented with 5% of dextrin or 5% of fructo-oligosaccharide. The results obtained with NUTRIOSE® were similar to those obtained with the fructo-oligosaccharide. In a third experiment, two groups of 5 Fischer F344 rats were orally treated with 100 and 1,000 mg/kg NUTRIOSE®, respectively, and from 18% to 25% of the dextrin was excreted in the feces. The results of these three studies show that the consumption of NUTRIOSE®, by its effects on total cecal weight, cecal content, cecal wall weight, pH, and SCFA production, could induce healthy benefits since these effects are reported to be prebiotic effects.
Prebiotics are defined as non-digestible food ingredients that beneficially affect the health of the host by selectively stimulating the growth and activity of specific species of bacteria in the gut [1]. Fibre-rich diets are considered to be protective against weight gain, cardiovascular disease, and colon cancer, and have beneficial effects on the human gastrointestinal tract [2-5]. In the second part of the last century, fibre intake decreased due to the evolution of food habits and is currently relatively low as compared to dietary recommendations (ANC "Apports Nutritionnels Conseillés" for the French population or recommended nutrient intakes). One way to increase fibre intake without increasing energy intake, is to replace some full-energy nutrients by low energy constituents, such as dietary fibres. Among them, and according to the new definition of dietary fibres published by Prosky 2001 [6], non digestible oligosaccharides (fructooligosaccharides, galactosaccharides, etc.) and resistant dextrins are two different types of fibres. The presence of their specific linkages (β, α1-2, α1-3...) does not permit the hydrolysis of these indigestible dextrins in the small intestine of mammals, and their energy content can be available for colonic flora. In the colon, they are fermented by intestinal bacteria. This bacterial fermentation in the colon leads to the production of short chain fatty acids (SCFA), mainly acetate, propionate, butyrate, and lactate [7-9]. A great proportion (80-85%) of these SCFA is absorbed by the mucosa of the colon and the other part is either evacuated in the feces or is used for growth and multiplication of bacteria. These indigestible dextrins can, as dietary fibres, have an action on fat and carbohydrate metabolism [7,10], on transit time [11], on mineral bioavailability [12], and on the densities of bifidobacteria and lactobacilli in the gastrointestinal tract [13]. Among the soluble fibres, some have already been largely studied, like fructo-oligosaccharides (FOS), oligofructoses, and xylooligosaccharides (XOS) [13-18]. The major disadvantage of these oligosaccharides is that as other indigestible carbohydrates, they induce digestible complaints often "incompatible" with their low energy substituent doses. There is a need to develop new dietary fibres that can be well tolerated at high doses. This is the case of the branched NUTRIOSE® dextrin, which is well-tolerated up to 45 grams per day [19,20]. Contrary to other dextrins, it is rich in α1-6, α1-2, and α1-3 linkages. Therefore, this dextrin is partly hydrolyzed and absorbed in the small intestine (15%). Large amounts of the ingested product reach the colon and are fermented by the gut microflora (75%).
The purpose of this rat study was to evaluate the nutritional effects of different doses of NUTRIOSE®. The results are focused on cecal parameters like cecal weight, cecal wet content, wall weight, pH, and SCFA concentrations. Moreover, the proportion of excreted NUTRIOSE® in the feces was also determined in the present study.
Rats were given free access to feed and fresh potable water. Prior to the experiment, the rats were fed a standard non-purified AO4C diet (SAFE, Villemoisson sur Orge, France, see composition in Table 1) and housed two rats per cage in Makrolon cages (37.5×23.5×16 cm) in an environmentally controlled room (21℃ ± 2, 50% ± 10) with a 12 h light/dark cycle. The animal use and experiments were performed according to the procedures described in the Guide for the Care and Use of Laboratory Animals (NIH, 1996) and in-house Standard Operating Procedures. The centre had a statement from the National Ministry of Agriculture to conduct preclinical research.
NUTRIOSE® (ROQUETTE Frères, Lestrem, France) is a glucose polysaccharide produced from maize, wheat, or other edible starch heated at high temperature and adjusted to a low moisture level in the presence of an acid catalyst. The dextrin obtained is purified with activated carbon and demineralised by exchange resins. Afterwards, the product is subjected to chromatographic partitioning that removes glucose and lower molecular weight oligosaccharides. As described in Table 2, the final product, NUTRIOSE®, is a mixture of glucose polymers with a fairly narrow range of molecular weight (number average Mol. Wt., Mw = 2,600 g/Mol; weight average Mol. Wt., Mw = 5,000 g/Mol). The degree of polymerization is 12-25. In comparison, starch may contain up to a million glucose units. During the heating step, hydrolysis and repolymerization occur. In addition to the typical starch α-1,4 and α-1,6 glucosidic linkages, the recombination can result in other specific glucosidic linkages that are not found in starch. Mass spectrometry is used following methylation of NUTRIOSE® to determine the bond distribution, which can include both linear and branched linkages: (α-1,6 and / or β-1,6), (α-1,2 and / or β-1,2), (α-1,3 and / or β -1,2), and β-1,4. This point confers to the resistance of NUTRIOSE® against the action of endogenous glucidolytic enzymes and permits classification of the product among soluble dietary fibres with a total fibre content of nearly 85.0%.
ACTILIGHT® (BEGHIN-MEIJI, Marckolsheim, France) is a fructo-oligosaccharide (FOS) obtained industrially from the action of fructosyl furanosidase (from Aspergillus niger) on sucrose. ACTILIGHT® is considered a soluble dietary fibre with prebiotic properties [4]. It was therefore chosen in this study as the reference product.
In the first trial, 24 male Fischer F344 rats, aged 5 weeks and weighing approximately 120 g at receipt, were supplied by CHARLES RIVER (L'Arbresle, France). They were randomly assigned to one of four dietary treatments (6 rats / treatment) and housed two rats per cage in Makrolon cages. The dextrin was added to the non-purified AO4C diet (composition in Table 1) as follows: G0: control diet; G2.5: control diet + 2.5% (w/w) dextrin; G5: control diet + 5% (w/w) dextrin; G10: control diet + 10% (w/w) dextrin. The duration of the experiment was 14 days. Food and water consumption and body weights were recorded weekly. The animals were individually observed each day. Stool consistency was recorded daily. After this 14-day period, cecal effects were evaluated.
In a second trial, 18 male Fischer F344 rats, aged 5 weeks and weighing approximately 120 g at receipt were supplied by CHARLES RIVER (L'Arbresle, France). They were randomly assigned to one of three dietary treatments (6 rats / treatment) and housed two rats per cage in Makrolon cages. Dextrin was added to the non-purified AO4C diet (composition in Table 1) as follows: G0: control diet; GD5: control diet + 5% (w/w) dextrin; GA5: control diet + 5% (w/w) ACTILIGHT®. The dietary supplementation duration was 14 days. Food and water consumption, and body weights were recorded weekly. The animals were individually observed each day. Stool consistency was recorded daily. After this 14-day period, cecal effects were evaluated.
In the third experiment, 15 male Fischer F344 rats, aged 5 weeks and weighing approximately 150 g at reception, were supplied by CHARLES RIVER (L'Arbresle, France). They were randomly assigned to one of three dietary treatments (5 rats / treatment) and individually housed in metabolic cages. The control group (GO) was treated orally by gastric intubation with a single dose of water at 10 mL/kg of body weight. The second group (G1) was treated orally with a single dose of a 10 mg per mL dextrin solution at 10 mL/kg of body weight, which corresponds to 100 mg/kg of body weight. The third group (G2) was treated orally with a single dose of a 100 mg per mL dextrin solution at 10 mL/kg of body weight, which corresponds to 1,000 mg/kg of body weight. The rat feces was collected for 48 hours to determine the fecal excretion of NUTRIOSE®.
Regarding the first and second experiments, after a 14-day treatment and an 18-h starvation period, the rats were sacrificed by CO2 asphyxia. A ventral midline incision was made and the caecum was removed. Immediately after removal, the caeca with contents were weighed. The cecal wall was weighed and the cecal contents were collected. The pH value was measured using a CG-825 Schott pH-meter, equipped with a microelectrode. Immediately there after, the caecum contents were mixed with a preservative solution (2.5 ml per g caecum content) consisting of phosphoric acid (0.63% v/v) and mercury chloride (0.091% w/v). One gram of cecal content was adjusted to 25 mL and centrifuged at 25,000 × g for 20 min. The supernatants were colorimetrically analyzed for acetate and lactate (Roche, Meylan, France), and for propionate and butyrate via gas chromatography using a VARIAN 3400 gas chromatograph and a capillary column for free fatty acids (DBFFAP, J&W Scientific FOLSOM, CA; 30 m × 0.32 mm i.d. film thickness: 0.25 micron). Helium was used as the carrier gas with a pressure of 10 psi with a split flow rate of 50 mL/minute. The oven temperature was 140℃ and increased by 2℃/minute up to 170℃. The detector temperature was 240℃ and injector temperature was 245℃. The employed detector was a flame ionization detector.
At the end of the third experiment, rat feces was collected for 24 and 48 h and weighed (wet weight). It were freeze-dried for 48 h (dry weight) and finally ground. The NUTRIOSE® residue was assessed by determining the fecal branched residual glucose. To do so, soluble carbohydrates were extracted from the feces using a 0.1% (w/v) chloramphenicol solution. Free glucose was determined enzymatically in the supernatant using a glucose oxidase kit (Roche, Meylan, France). Total glucose (polymerized plus free) was determined after acid hydrolysis (HCl 4N) for 45 minutes at 100℃ using the same reagent kit as the one used for free glucose. The NUTRIOSE® residue was estimated by deducting the fecal free glucose from the total fecal glucose.
The data were analysed using Kruskall Wallis tests for non parametric data to test the dose effect. When global analysis was significant (P < 0.05), the Kolmogorov Smirnov test was used to compare the different treated groups to the control group. The tests were carried out on SAS® software version 8.
From experiment 1, the mean dietary preparation intakes ranged from 19.4 ± 0.6 to 20.3 ± 1.0 g/animal/day during week 1 (Table 3). During week 2, the animals consumed from 21.4 ± 0.4 to 23.4 ± 1.3 g/animal/day. There were no differences between the groups (Table 3). The mean tested product consumptions were 0.51, 1.08, and 2.10 g/animal/day for groups G2.5, G5, and G10, respectively. Slightly softer feces was observed in the 10% treated animals, but at this level, as in the other group, no diarrhea was observed. Animal behaviour and external aspects were similar in the treated animals compared to the controls.
There was no difference in body weight evolution (Table 3). At the beginning of the experiment the animals weighed from 149 ± 8 g (G10) to 152 ± 9 g (G0), and 14 days later from 231 ± 3 g (G2.5) to 240 ± 9 g (G5). No significant differences were noted between the groups. There was also no difference in water consumption (from 19.3 ± 0.6 to 21.5 ± 1.4 g/animal/d; data not shown).
The results for cecal weight, cecal contents, cecal walls, and cecal pH, obtained from experiment 1, are presented in Table 4. There was a dose-effect relationship between the dose of the product consumed and the cecal effects. There were significant differences at the doses of 5% and 10% in the feed for total cecal weight, cecal content, and cecal wall weight. In group G10, these 3 parameters were, on average, 65%, 72%, and 31% higher, respectively, than in G0 group (Table 4).
The cecal pH fell 2.9% in the 5% group compared to the G0 group (6.39 ± 0.19 versus 6.58 ± 0.13). This difference was not significant, whereas at 10% dextrin in the feed (Table 4), the decrease was significantly different (8.7% decrease; P < 0.01).
The cecal SCFA concentrations are presented in Table 4 (experiment 1). The cecal concentrations of acetate were 42% and 88% higher in the groups fed additions of 5% and 10% of NUTRIOSE®, respectively, compared to rats fed the control diet [G5: 24.45 mg/caecum content ± 4.72; G10: 32.36 mg/caecum content ± 3.42 with P < 0.01 and P < 0.001, respectively, compared to the control group (G0: 17.19 mg/caecum content ± 1.65)]. For propionate, the smallest dose of 2.5% NUTRIOSE® induced a 57% significant increase in propionate concentration compared to the control group (3.37 mg/caecum ± 0.77 versus 2.15 mg/caecum ± 0.53; P < 0.05). At 5% and 10%, the dextrin led to increases of 94% (4.17 mg/caecum ± 1.45 versus 2.15 mg/caecum ± 0.53; P < 0.05) and 155% (5.48 mg/caecum ± 0.84 versus 2.15 mg/caecum ± 0.53; P < 0.001), respectively. Finally, in the 5% group, there was a statistically significant increase of 35% in butyrate concentration (22.48 mg/caecum ± 3.68 versus 16.70 mg/caecum ± 3.03; P < 0.05). This was confirmed in the 10% group with a 47% increase (24.55 mg/caecum ± 2.61 versus 16.70 mg/caecum ± 3.03; P < 0.001) in butyrate concentration (Table 4).
When expressed in mg/caecum (Table 4), total short chain fatty acids were increased from the 5% dose. This augmentation was from 42% (P < 0.01) to 73% (P < 0.001) for the 5% and 10% groups, respectively. The results for lactic acid are not presented because there was no production of this acid by any group.
The results for cecal weights, cecal contents, cecal wall, and cecal pH in experiment 2 are presented in Table 5. Both products induced significant increases of cecal weight, cecal contents, and wall weight (Table 5). Inversely, pH values were decreased in both groups treated by 5% NUTRIOSE® and 5% ACTILIGHT®.
For SCFA, propionate and total SCFA were significantly increased in both groups. Acetate and butyrate were significantly different after treatment with 5% NUTRIOSE®. After treatment with 5% ACTILIGHT®, acetate and butyrate increased but the differences were not significantly different.
After treatment with 5% NUTRIOSE®, fecal branched residual glucose values were increased (Table 6). The variations in fecal glucose between the control and treated groups were 6.45 mg and 44.96 mg for 100 mg/kg bwt and 1,000 mg/kg bwt NUTRIOSE® intake, respectively. The percentages of glucose excretion in the rats for both tested doses were rather similar: 25% and 18% for 100 mg/kg bwt and 1,000 mg/kg bwt NUTRIOSE®, respectively.
Carbohydrates that reach the large intestine, so-called low digestible carbohydrates (LDC), induce laxative effects essentially due to the stimulation of peristalsis [9] and to the osmotic power of LDC. FOS and inulin exhibit laxative effects at relatively small dosages in animals and in human [11,21]. In our study, animals in group G10 consumed up to 10 g/kg animal/day of dextrin and only soft feces was observed. At this level, as for the other groups, no sign of diarrhea was observed. NUTRIOSE® at all tested doses did not modify the appearance of the feces compared to the control group, suggesting no effects on peristalsis and secretion in the colon. There was no difference in body weight evolution. At the beginning of the experiment, the animals ranged from 149 g (G10) to 152 g (G0) and 14 days later from 231 g (G2.5) to 240 g (G5). No significant differences were noted between the groups. Thus, this dextrin did not increase dietary intake. Several studies have demonstrated that low digestible carbohydrates were fermented by bacteria and fecal recovery was found to be zero [1]. Such is the case for fructo-oligosaccharides. In contrast, for our study, fecal recovery was about 20% of the ingested dose, demonstrating the incomplete digestion of this dextrin in rats and resulting in a low caloric value. After 2 weeks of NUTRIOSE® consumption, no physiopathologic damage was observed, whatever the dose as compared to the control group. Thus, the tolerance of the product was excellent. Similar levels of excretion were found in humans fed this dextrin [22]. Taken together, the results indicate that the product was well tolerated, and more particularly in the gastrointestinal tract. These results are in good accordance with the digestive tolerance and fecal excretion of this dextrin in humans [20,22,23].
In our study, the dextrin tested is a very good substrate for cecal fermentation. The highly fermentable product leads to an increase in cecal weight due to the production of bacterial mass in the caecum [13]. The minimum dose to induce cecal enlargement seems to be between 2.5% and 5%. Effectively, there was a high increase in absolute cecal weight (more than 35%) in the 5% treated animals, whereas the increase observed in the 2.5% group (3%) was not statistically significant. The increase of cecal wall may be attributed to an increase in intestinal mucosa thickness as a consequence of the large amount of SCFA produced and as an adaptive process to highly fermentable carbohydrates. Indeed, SCFA production induces the proliferation of cecal cells. It was demonstrated that FOS and XOS increased cecal weight and cecal wall weight compared to a diet without fibres [13]. All the three major short chain fatty acids (acetate, propionate, and butyrate) were increased by NUTRIOSE®. Based on the data, it also appears that the minimal dose to induce a positive effect is between 2.5 and 5%. High levels of SCFA, particularly of butyrate, in the lower part of the gastrointestinal tract are important for a healthy large intestine. Butyrate, known to be the preferred energy substrate for colonocytes, was increased to a large extent in this study and may be correlated with cecal wall augmentation. Indeed, in addition to the trophic effect of butyrate on the mucosa, it is an important source of energy for the colonic epithelium and can regulate both the growth and the differentiation of colonic cells. The fact that at the 2.5% dose there was a statistically significant increase in propionate production suggests that this dextrin is a high propiogenic oligosaccharide. The effect of a diet containing propionate was found to be associated with lower fasting glycemia in rats [10]. An in vitro study on rat hepatocytes showed that propionate can decrease gluconeogenesis, while on the other hand stimulate glycolysis [7]. Another study in humans [24] showed that the addition of propionate to bread inhibited the activity of amylase. Thus, in the present study, the observed increase of propionate production allows one to expect an affect of this low digestible carbohydrate on glycemia. The acetate increase observed in this type of experiment is often related to fermentable carbohydrates [13,25-27]. Lactic acid accumulation was not noted during the study. This would indicate that the process of fermentation was not overdosed even at the highest dose of 10%.
Similar effects on cecal parameters and SCFA production were observed with the reference FOS ACTILIGHT®. In conclusion, NUTRIOSE® was as efficient as ACTILIGHT® to promote the production of cecal SCFA.
In rats, fermentation is essentially localized in the caecum. The caecum has the highest level of SCFA available for absorption/utilization. As a result of fermentation, pH decreases. This phenomenon was indicated as a statistical decrease in the 5% treated group in the second experiment and in the 10% treated group in the first experiment. An acidic cecal pH is probably caused by greater levels of total SCFA production, but it can also be caused by a decrease of nitrogen compounds, which are quite basic [20]. This increased acid production can prevent the colonization of potentially pathogenic microorganisms [28]. This phenomenon can also be associated with the proliferation of bifidobacteria and lactobacilli in the GI tract. Indeed, the lowering of pH and the production of metabolites stimulate the growth of prebiotic bacteria and the promotion of bacteriostatic effects against potentially harmful bacteria like enterobacteria and clostridia [3]. Vermorel et al. [29] showed that NUTRIOSE® can improve magnesium and calcium absorption, and their retention, in humans. The authors indicated that this phenomenon could be linked to the decrease of pH of gut content as encountered in our study. Prebiotics are not only nutrients for bacteria, but can also induce environmental changes such as changes in luminal pH, mineral absorption [30], trophicity, and improvement of the immune system [31].
In conclusion, NUTRIOSE® dextrin increased SCFA production in the caecum of rats. Butyrate can provide a source of energy for the large bowel; suggesting that this indirect energy source may play a role in mucosal cell differentiation. An increase in SCFA may be due to an increase in the colonic bacteria that are able to ferment NUTRIOSE® residues. This possible effect on microflora may prevent the colonization of potential pathogens and enhance the immune system. Further studies of the effects of this dextrin on intestinal microflora composition and the immune system are necessary to sustain their beneficial health effects.
Figures and Tables
Table 4
Effects of NUTRIOSE® on cecal parameters and SCFA production
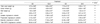
The values are the mean ± SEM, n = 6. Data were analysed using Kruskall Wallis test for non parametric data to test the dose effect. When global analysis was significant (P < 0.05), the Kolmogorov Smirnov test was used to compare the different treated groups to the control group.
a,b,c,d significantly different from G0 (a : NS; b : P < 0.05; c : P < 0.01; d : P < 0.001)
1) global P-value (Kruskal-Wallis) *< 0.05, **< 0.01, ***< 0.001.
References
1. Gibson GR, Probert HM, Loo JV, Rastall RA, Roberfroid MB. Dietary modulation of the human colonic microbiota: updating the concept of prebiotics. Nutr Res Rev. 2004. 17:259–275.


3. Duggan C, Gannon J, Walker WA. Protective nutrients and functional foods for the gastrointestinal tract. Am J Clin Nutr. 2002. 75:789–808.


5. Geier MS, Butler RN, Howarth GS. Probiotics, prebiotics and synbiotics: a role in chemoprevention for colorectal cancer? Cancer Biol Ther. 2006. 5:1265–1269.


6. Prosky L. AOAC International. Committee on Food Nutrition. Dietary fiber. J AOAC Int. 2001. 84:222–224.


7. Anderson JW, Bridges SR. Short-chain fatty acid fermentation products of plant fiber affect glucose metabolism of isolated rat hepatocytes. Proc Soc Exp Biol Med. 1984. 177:372–376.


8. Berggren AM, Björck IM, Nyman EM, Eggum BO. Short-chain fatty acid content and pH in caecum of rats given various sources of carbohydrates. J Sci Food Agric. 1993. 63:397–406.


9. May T, Mackie RI, Fahey GC Jr, Cremin JC, Garleb KA. Effect of fiber source on short-chain fatty acid production and on the growth and toxin production by Clostridium difficile. Scand J Gastroenterol. 1994. 29:916–922.


10. Boillot J, Alamowitch C, Berger AM, Luo J, Bruzzo F, Bornet FR, Slama G. Effects of dietary propionate on hepatic glucose production, whole-body glucose utilization, carbohydrate and lipid metabolism in normal rats. Br J Nutr. 1995. 73:241–251.


11. Cummings JH, Macfarlane GT, Englyst HN. Prebiotic digestion and fermentation. Am J Clin Nutr. 2001. 73:415S–420S.


12. Demigné C, Rémésy C, Rayssiguier Y. Effect of fermentable carbohydrates on volatile fatty acids, ammonia and mineral absorption in the rat caecum. Reprod Nutr Dev. 1980. 20:1351–1359.


13. Campbell JM, Fahey GC Jr, Wolf BW. Selected indigestible oligosaccharides affect large bowel mass, cecal and fecal short-chain fatty acids, pH and microflora in rats. J Nutr. 1997. 127:130–136.


14. Djouzi Z, Andrieux C. Compared effects of three oligosaccharides on metabolism of intestinal microflora in rats inoculated with a human faecal flora. Br J Nutr. 1997. 78:313–324.


15. Levrat MA, Rémésy C, Demigné C. High propionic acid fermentations and mineral accumulation in the cecum of rats adapted to different levels of inulin. J Nutr. 1991. 121:1730–1737.


16. Roberfroid MB. Concepts in functional foods: the case of inulin and oligofructose. J Nutr. 1999. 129:1398S–1401S.


17. Roberfroid M. Dietary fiber, inulin, and oligofructose: a review comparing their physiological effects. Crit Rev Food Sci Nutr. 1993. 33:103–148.


18. Roberfroid MB. Health benefits of non-digestible oligosaccharides. Adv Exp Med Biol. 1997. 427:211–219.


19. Lefranc-Millot C. NUTRIOSE® 06: a useful soluble dietary fibre for added nutritional value. Nutrition Bulletin. 2008. 33:234–239.
20. van den Heuvel EG, Wils D, Pasman WJ, Bakker M, Saniez MH, Kardinaal AF. Short-term digestive tolerance of different doses of NUTRIOSE FB, a food dextrin, in adult men. Eur J Clin Nutr. 2004. 58:1046–1055.


21. Gibson GR, Beatty ER, Wang X, Cummings JH. Selective stimulation of bifidobacteria in the human colon by oligofructose and inulin. Gastroenterology. 1995. 108:975–982.


22. van den Heuvel EG, Wils D, Pasman WJ, Saniez MH, Kardinaal AF. Dietary supplementation of different doses of NUTRIOSE FB, a fermentable dextrin, alters the activity of faecal enzymes in healthy men. Eur J Nutr. 2005. 44:445–451.


23. Pasman W, Wils D, Saniez MH, Kardinaal A. Long-term gastrointestinal tolerance of NUTRIOSE FB in healthy men. Eur J Clin Nutr. 2006. 60:1024–1034.


24. Todesco T, Rao AV, Bosello O, Jenkins DJ. Propionate lowers blood glucose and alters lipid metabolism in healthy subjects. Am J Clin Nutr. 1991. 54:860–865.


25. Propst EL, Flickinger EA, Bauer LL, Merchen NR, Fahey GC Jr. A dose-response experiment evaluating the effects of oligofructose and inulin on nutrient digestibility, stool quality, and fecal protein catabolites in healthy adult dogs. J Anim Sci. 2003. 81:3057–3066.


26. Smiricky-Tjardes MR, Grieshop CM, Flickinger EA, Bauer LL, Fahey GC Jr. Dietary galactooligosaccharides affect ileal and total-tract nutrient digestibility, ileal and fecal bacterial concentrations, and ileal fermentative characteristics of growing pigs. J Anim Sci. 2003. 81:2535–2545.


27. Wong JM, de Souza R, Kendall CW, Emam A, Jenkins DJ. Colonic health: fermentation and short chain fatty acids. J Clin Gastroenterol. 2006. 40:235–243.


28. Gibson GR, Roberfroid MB. Dietary modulation of the human colonic microbiota: introducing the concept of prebiotics. J Nutr. 1995. 125:1401–1412.


29. Vermorel M, Coudray C, Wils D, Sinaud S, Tressol JC, Montaurier C, Vernet J, Brandolini M, Bouteloup-Demange C, Rayssiguier Y. Energy value of a low-digestible carbohydrate, NUTRIOSE FB, and its impact on magnesium, calcium and zinc apparent absorption and retention in healthy young men. Eur J Nutr. 2004. 43:344–352.

