Abstract
This study investigated the effect of coffee intake and exercise on the antioxidative activity and plasma cholesterol profile of physically trained rats while they were exercising. Forty eight rats were under either the control diet with water (C) or control diet with coffee (CF) and at the same time they were given physical training for 4 weeks. In terms of physical training, the rats were exercised on a treadmill for 30 minutes everyday. At the end of 4 weeks, animals in each dietary group were subdivided into 3 groups: before-exercise (BE); during-exercise (DE); after-exercise (AE). Animals in the DE group were exercised on a treadmill for one hour, immediately before being sacrificed. Animals in the AE group were allowed to take a rest for one hour after exercise. TG levels were significantly high in coffee intake group than in control group. Also TG level of AE group was significantly higher than that of BE group. Exercise and coffee-exercise interaction effects were significant in total cholesterol (P = 0.0004, 0.0170). The AE of coffee intake group showed highest total cholesterol levels. HDL-cholesterol was significantly lower in coffee intake group than in control group. Coffee, exercise, and coffee-exercise interaction effects were significant in SOD (P = 0.0001, 0.0001, and 0.0001). The AE and BE of coffee intake group showed higher SOD levels than the other four groups. Catalase activities were significantly higher in coffee intake group than control group. No significant main effect was found in GSH/GSSG. Coffee, exercise, and coffee-exercise interaction effects were significant in MDA levels (P = 0.0464, 0.0016, and 0.0353). The DE and AE of coffee intake group and the DE of control group showed higher MDA levels than the BE of control group. Therefore, coffee intake can promote activities of antioxidant enzyme but it also increases MDA and decreases HDL-cholesterol in physically trained rats.
Many studies have sought to determine that aerobic exercise is associated with production of ROS and tissue damage in the body. These ROS are eliminated by an elaborate antioxidant defense system consisting of enzymes such as catalase, superoxide dismutase, glutathione peroxidase, and numerous non-enzymatic antioxidants, including vitamins A, C and E, glutathione, ubiquinone, and flavonoids [1]. Depletion of each of the antioxidant systems increases the vulnerability of various tissues and cellular components to reactive oxygen species. Antioxidant supplements are commonly used by athletes as a means to counteract the oxidative stress of exercise. Whether strenuous exercise does, in fact, increase the need for additional antioxidants in the diet is not clear [2]. On the other hand, ROS generated during exercise act as signals to increase the production of antioxidant enzymes relevant to the adaptation of muscle cells to exercise as an adaptive response [3]. Indeed, when rats were trained, the expression of antioxidant enzymes was increased [4]. Moreover, other research reported that interfering with free radical metabolism with antioxidant supplements may prevent useful adaptations to training [5]. But, lots of studies have sought to determine that antioxidant supplements would benefit those who exercise regularly [6,7]. Coffee is one of the most widely consumed beverages in the world. Habitual coffee drinking has been associated with the prevention of diseases including cancer [8], cardiovascular disorders [9], obesity and diabetes [10] as well as neurodegenerative disorders [11,12]. Recently, coffee has aroused scientific interests because it is a rich source of a number of phenol compounds with antioxidant effects in vitro [13], stimulation of NO production and vasodilation [14], and regulation of xenobiotic-metabolizing enzymes [15]. Main polyphenols in coffee are phenolic acids such as chlorogenic and caffeic acid. Caffeine and its metabolites, di-and monomethylxanthines, have also been shown to have antioxidative properties [16]. But lots of studies also reported that caffeine, obtained from coffee, may decrease insulin sensitivity [17], increase serum homocysteine [18] and blood pressure [19]. Also it can be an important factor that increases the CHD risk. Moreover, coffee was demonstrated to act in vitro as pro-oxidant [1,14]. In our previous study, freeze dried instant coffee intake increased activities of antioxidant enzymes but it also increased MDA and total cholesterol in rats [20]. In this study, coffee was fed as a solution of freeze dried coffee (Dongseo Co., Korea) and the average amount of coffee intake per rat was 0.12 g freeze dried instant coffee/100 g body weight/day. This concentration was based on the rationale as follows: to get the visible effects of coffee intake, we maximized the amount of coffee intake, but did not exceed the upper limit of caffeine consumption, which can be approximately 895 mg/60 kg/day of caffeine because there was a report that the caffeine intake of physically active individuals went up to 895 mg/day [21]. Also, there were reports that caffeine content of food source was 106 mg × [1 serving] in espresso, 58 mg × [mL.sup.-100] in coffee, 25 mg × [mL.sup.-100] in black tea, and 15 mg × [mL.sup.-100] in green tea [22,23]. Thus, on the basis that a cup of coffee contains 87 mg of caffeine per 150 ml of coffee, 10 cups of coffee (7.2 g of freeze dried instant coffee per one cup) contains 870 mg of caffeine which would not exceed the upper limit of daily caffeine consumption for 60 kg men. If this concentration were applied to rats by weight-based approach, the concentration would be 0.12 g/100 g BW/day of coffee which contains 1.45 mg/100 g/day of caffeine. Therefore, we investigated the effect of instant coffee intake on the antioxidative activity and plasma cholesterol profiles in rats with this concentration of coffee.
Forty eight male Sprague-Dawley rats (Daehanbiolink Co., Korea) weighing 65-75 g were fed either the control diet with water (C) or control diet with coffee (0.12 g/100 g body weight/day freeze dried instant coffee, Dongseo Co., Korea) (CF), and they were given physical training for 4 weeks. For physical training, animals were exercised on a treadmill for 30 minutes everyday. The control diet was vitamin-free casein based semisynthetic diet which met AIN-93 recommendation.
At the end of 4 weeks, animals in each dietary group were subdivided into 3 exercise groups: before-exercise (BE); during-exercise (DE); after-exercise (AE). Animals in BE group were sacrificed without exercise at the end of week 4. Animals in DE group were exercised on a treadmill (15° incline, 0.5-0.8 km/h) for 1 hour; animals in the AE group were allowed to take a rest for 1 hour after exercise. At the respective time points, animals were sacrificed by decapitation under the light ether anesthesia. Immediately following decapitation, plasma, muscle and liver were rapidly removed and stored at -40℃ until analyzed. Blood samples were collected in heparinized tubes. All blood samples were immediately centrifuged (3,000 rpm, 20 min, 4℃) for the separation of plasma and erythrocytes.
The activity of plasma catalase was determined with a commercial kit based on the method of Zamocky (Bioxytech Catalase-520). The activity of superoxide dismutase, the ratio of reduced glutathione and oxidized glutathione, and the levels of malondialdehyde were determined in liver cytosol. Liver was homogenized in cold Tris-KCl buffer (0.1 M) and the homogenized solution was centrifuged (8,000×g, 4℃, 30 min). The supernatant was then centrifuged (10,000×g, 4℃, 30 min). Again the supernatant was ultra-centrifuged (105,000×g, 4℃, 90 min) and separated the cytosol. Superoxide dismutase (SOD) activity was determined with a commercial kit based on the method of Nebot (Bioxytech SOD-525). The ratio of reduced glutathione/oxidized glutathione (GSH/GSSG) was determined with a commercial kit based on the method of Anderson (Bioxytech GSH/GSSG-412). The levels of malondialdehyde (MDA) were determined with a commercial kit based on the method of Gerard-Monnier (Bioxytech MDA-586). Plasma Triglyceride (TG) was analyzed with a commercial kit based on the Trinder method (Youngdong Pharmaceutical Co., Korea). Total cholesterol (TC) was analyzed with a commercial kit based on enzymatic method (Youngdong Pharmaceutical Co., Korea). High-density lipoprotein-cholesterol (HDL-C) was analyzed with a commercial kit based on the same analytical method as total cholesterol after the precipitation.
The data were expressed as mean values and their standard deviation (SD). The significance of difference between control group and coffee intake group was analyzed using t-test at P value < 0.05. The variables were analyzed by two-way analysis of variance (ANOVA) with coffee intake and exercise as factors. When a significant interaction effect was found, Scheffe post hoc tests were performed. When a significant coffee intake or exercise effect was found and the interaction effect was insignificant, one-way ANOVA was used to compare the means of the groups. All analyses were performed using SAS 9.1 for Windows (SAS Institute, Inc., Cary, NC,). The statistical significance level was set at P < 0.05.
Table 1 shows the effect of coffee intake on body weight and food efficiency ratio in physically trained rats. There were no significant differences in final body weight, food intake and food efficiency ratio of the groups. Coffee effect was not shown on body weight and food efficiency ratio.
The differences in plasma TG, TC and HDL-C are shown in Table 2 and Fig. 1. Significant coffee and exercise effects on TG levels were present (P = 0.0085, 0.004). Individual coffee and exercise effects were analyzed. TG levels were significantly higher in coffee intake group than in control group. TG level of AE group was significantly higher than those of BE and DE groups. Exercise and coffee-exercise interaction effects were significant on total cholesterol (P = 0.0004, 0.0170, respectively, Fig. 1). The AE of coffee intake group showed highest TC levels and the DE of control group showed lowest TC levels. Coffee intake increased TC levels in DE and AE, but in BE group, significant increase was not shown. In control group, TC levels of DE were lower than those of BE and AE. In coffee intake group, TC levels of AE were higher than those of BE and DE. Coffee effect was significant on HDL-C, which was significantly lower in coffee intake group than in control group.
Table 3 and Fig. 2 show the effect of coffee intake and exercise on SOD activity. Coffee, exercise, and coffee-exercise interaction effects were significant on SOD (P = 0.0001, 0.0001, and 0.0001, respectively, Fig. 2). The AE and BE of coffee intake group showed higher SOD levels than the other four groups. Coffee intake increased SOD activities in BE and AE, but in DE group, significant increase was not shown. In coffee intake group, SOD activities of DE were lower than those of BE and AE.
The effect of coffee intake and exercise on catalase activities is shown in Table 4. A significant coffee effect (P = 0.0008) was found in catalase activities. Since the interaction effect was absent, the individual effect of coffee could be analyzed. Catalse activities were significantly higher in coffee intake group than in control group.
Table 5 shows the effect of coffee intake and exercise on GSH/GSSG. No significant main effect was found in GSH/GSSG. Coffee intake and exercise did not affect GSH/GSSG.
The effect of coffee intake and exercise on MDA levels is shown in Table 6 and Fig. 3. Coffee, exercise, and coffee-exercise interaction effects were significant on MDA levels (P = 0.0464, 0.0016, and 0.0353, respectively, Fig. 3). The DE and AE of coffee intake group and the DE of control group showed higher MDA levels than the BE of control group. Coffee intake increased MDA levels in BE and AE, but in DE group, significant increase was not shown. In control group, MDA levels of DE were higher than those of BE and AE.
This study showed that freeze dried instant coffee consumption induced a favorable turn on of the activities of antioxidant enzymes but also increased MDA and decreased HDL-cholesterol in physically trained rats. The hypothesis was that coffee consumption, as an antioxidant, can react effectively to exercise-induced oxidative stress, increase the activity of antioxidant enzymes and decrease cholesterol level in physically trained rats. The evidence of an alteration in the antioxidative status with oxidative stress is based on the change of antioxidant enzyme activities and lipid peroxidation under exercise induced oxidative stress. At various points during the study, the antioxidative status in rats was evaluated using the ratios of GSH/GSSG and the activities of catalase and SOD as a direct measure, and the level of MDA and lipid profile as an indirect, long-term measure. Coffee intake increased catalase and SOD activities in other study [24]. Consistently in this study, SOD activities of the CF group were significantly higher than those of the C group in BE and AE. But in DE, there were no significant differences in the SOD activities. In SOD activities of the C group, there were no differences regardless of exercise. However in the CF group, SOD activities of AE were significantly higher than those of BE and DE, and especially those of DE were significantly lower than those of BE and AE. It can be supposed that coffee consumption can increase the activities of SOD and help recuperation of antioxidant defense system in physically trained rats, but coffee intake did not react effectively in DE. Catalase activities of the CF group were significantly higher than those of the C group. No significant main effect was found in GSH/GSSG. It means that coffee intake and exercise induced oxidative stress did not induce the change of GSH/GSSG in trained rats. Other previous research showed considerable evidence that training adaptation of antioxidant activity is due to altered gene expression, with both mRNA and enzyme protein levels being up regulated [25]. Training adaptation of antioxidant enzymes is influenced heavily by a number of physiological and environmental factors, such as gender, age, diet, and medication [26]. Exercise training in animals fed an antioxidant-deficient diet, such as vitamin E and selenium, has been shown to elicit greater levels of antioxidant adaptation than those fed normal diet [27,28]. Moreover, those researches recommend us to reconsider the taking of antioxidant supplements during training. In all probability, antioxidant supplements should not be recommended before training as they hinder with muscle cell adaptation [29]. When antioxidants were given, these adaptations were, however, not permitted [5]. On the other hand, antioxidants may be administered before competition, when exercise is likely to be exhaustive and result in the generation of ROS that overwhelm the defensive mechanisms [30]. Consistently, in this study, CF group freely received coffee in tap water with exercise training during four weeks, and coffee intake could increase the activities of catalase and SOD in BE and AE but did not react efficiently in DE. Considerable numbers of studies have demonstrated that oxidative stress can occur during exercise [31]. Oxygen consumption can be increased several-fold with exercise [32]. Xanthine dehydrogenase oxidizes hypoxanthine to xanthine and xanthine to uric acid using NAD+ as the electron acceptor forming NADH and superoxide [33]. Tissue damage resulting from exercise may induce the activation of inflammatory cells such as neutrophils, with the subsequent production of ROS by NADPH oxidase [34]. Catecholamine concentrations are increased during exercise, and ROS can result from their auto-oxidation [35]. Muscle mitochondria undergo increased uncoupling and superoxide generation with increasing temperatures. Therefore, exercise-induced hyperthermia may cause oxidative stress [36]. An increase in temperature and relative humidity from 20℃ and 40%, respectively, to 30℃ and 80%, respectively, was associated with a greater increase in the oxidation of glutathione in red blood cell hemolysate and lipid peroxidation in plasma following a submaximal exercise test in horses [37]. Auto-oxidation of oxyhemoglobin to methemoglobin results in the production of superoxide and the rate of formation of methemoglobin can be increased with exercise [38]. Therefore, during exercise, increased ROS need more actions of antioxidants. But coffee intake group of DE could not defend ROS effectively. MDA levels of the CF group were significantly higher than those of the C group in BE and AE. In the CF group, there were no differences in MDA levels regardless of exercise, but in the C group, MDA levels of DE were significantly higher than those of BE and AE. It can be supposed that coffee can be pro-oxidant or its metabolites can increase lipid peroxidation, but the levels of MDA were equilibrium under exercise induced antioxidative stress. Other investigation showed that generation of H2O2 accounts for the genotoxic effects of coffee [39] and another reported that chlorogenic and caffeic acids and their pyrolysis products which are contained in coffee cause formation of ROS [40], and also caffeine was shown to possess pro oxidant properties under specific conditions [41]. Moreover, coffee was demonstrated to act in vitro as pro-oxidant [14,42]. There is considerable evidence that increased antioxidant defense system is due to altered gene expression, with both mRNA and enzyme protein levels, but data are limited and sometimes controversial [25,43]. For those points of view, some coffee contents can help increase antioxidant defense system, but the other contents or the decomposed contents of coffee which can come with roasting degree, type of coffee bean, the amount of consumption and preparation method can be pro oxidant [14,21], those can induce increased MDA levels. In addition, TC levels of the CF group were not significantly different from those of the C group regardless of exercise. Whereas HDL-C levels of the CF group were significantly lower than those of the C group. Moderate exercise-training has been reported to increase HDL-cholesterol [4,44], but in this study, coffee intake decreased HDL-cholesterol in trained rats. The cholesterol-raising effect of coffee intake has long been controversial [45]. But other research reported that the effect of coffee diterpenes on total cholesterol is due to raised LDL and VLDL cholesterol and decreased HDL cholesterol [46]. The consumption of 450-1,000 ml of coffee has increased the plasma total homocysteine (tHcy) concentration by 19% at 4 h and 11-22% after 2 to 4 weeks [18,47]. Elevated plasma tHcy concentrations have been associated with an increased risk of cardiovascular diseases in many case-control studies and in some, but not all, prospective cohort studies [48]. On the other side, some studies have investigated the role of homocysteine in promoting oxidative stress and have obtained conflicting results. There was a significant correlation between homocysteine and MDA but it is also conflicted [49]. Even though coffee consumption would increase the tHcy concentration, there is no convincing evidence that coffee consumption would increase the risk of CVD [50]. By all accounts, this study observed coffee intake induced significant increase in MDA levels and significant decrease in HDL-cholesterol, and other studies reported that coffee intake increased tHcy. It can be supposed that coffee intake can be associated with risk factors of CVD. Therefore, despite the many uncertainties regarding the mode of action, these results suggest that coffee intake can promote activities of antioxidant enzyme but also increase MDA and decrease HDL-cholesterol in physically trained rats. Further researches are required to demonstrate effects of appropriate time and amount of coffee intake on physically trained rats under exercise induced antioxidative stress.
Figures and Tables
Fig. 1
The effect of coffee intake and exercise on total cholesterol (Tcol) in physically trained rats. Which are determined by two-way ANOVA. Data were means and letters in the same figure were significantly different at P < 0.05. Control: control diet + physical training, coffee: coffee intake + physical training. BE: before exercise, DE: during exercise, AE: after exercise
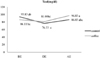
Fig. 2
The effect of coffee intake and exercise on superoxide dismutase (SOD) in physically trained rats. Which are determined by two-way ANOVA. Data were means, and letters in the same figure were significantly different at P < 0.05. Control: control diet + physical training, coffee: coffee intake + physical training. BE: before exercise, DE: during exercise, AE: after exercise
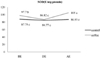
Fig. 3
The effect of coffee intake and exercise on malondialdehide (MDA) in physically trained rats. Which are determined by two-way ANOVA. Data were means, and letters in the same figure were significantly different at P < 0.05. Control: control diet + physical training, coffee: coffee intake + physical training. BE: before exercise, DE: during exercise, AE: after exercise
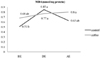
Table 2
The effect of coffee intake and exercise on the triglyceride, total cholesterol and HDL-cholesterol in trained rats
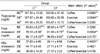
Table 3
The effect of coffee intake and exercise on superoxide dismutase (SOD) activity in trained rats
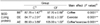
Table 5
The effect of coffee intake and exercise on reduced glutathione/oxidized glutathione (GSH/GSSG) in trained rats
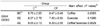
References
1. Urso ML, Clarkson PM. Oxidative stress, exercise, and antioxidant supplementation. Toxicology. 2003. 189:41–54.


2. Jackson MJ. Hanninen O, Packer L, Sen CK, editors. Handbook of Oxidants and Antioxidants in Exercise. 2000. Amsterdam: Elsevier;57–68.
3. Hollander J, Fiebig R, Gore M, Ookawara T, Ohno H, Ji LL. Superoxide dismutase gene expression is activated by a single bout of exercise in rat skeletal muscle. Pflugers Arch. 2001. 442:426–434.


4. Choi EY, Cho YO. The effects of physical training on antioxidative status under exercise-induced oxidative stress. Nutr Res Pract. 2007. 1:14–18.


5. Gomez-Cabrera MC, Borras C, Pallardo FV, Sastre J, Ji LL, Vina J. Decreasing xanthine oxidase-mediated oxidative stress prevents useful cellular adaptations to exercise in rats. J Physiol. 2005. 567:113–120.


6. Choi EY, Cho YO. Allium vegetable diet can reduce the exercise-induced oxidative stress but does not alter plasma cholesterol profile in rats. Ann Nutr Metab. 2006. 50:132–138.


7. Morillas-Ruiz JM, Villegas García JA, López FJ, Vidal-Guevara ML, Zafrilla P. Effects of polyphenolic antioxidants on exercise-induced oxidative stress. Clin Nutr. 2006. 25:444–453.


8. Ogunleye AA, Xue F, Michels KB. Green tea consumption and breast cancer risk or recurrence: a meta-analysis. Breast Cancer Res Treat. 2010. 119:477–484.


9. Kuriyama S, Shimazu T, Ohmori K, Kikuchi N, Nakaya N, Nishino Y, Tsubono Y, Tsuji I. Green tea consumption and mortality due to cardiovascular disease, cancer, and all causes in Japan: the Ohsaki study. JAMA. 2006. 296:1255–1265.


10. Thielecke F, Boschmann M. The potential role of green tea catechins in the prevention of the metabolic syndrome-a review. Phytochemistry. 2009. 70:11–24.


11. Hu G, Bidel S, Jousilahti P, Antikainen R, Tuomilehto J. Coffee and tea consumption and the risk of Parkinson's disease. Mov Disord. 2007. 22:2242–2248.


12. Eskelinen MK, Ngandu T, Tuomilehto J, Soininen H, Kivipelto M. Midlife coffee and tea drinking and the risk of late-life dementia: a population-based CAIDE study. J Alzheimer's Dis. 2009. 16:85–91.


13. Scalbert A, Williamson G. Dietary intake and bioavailability of polyphenols. J Nutr. 2000. 130:2073–2085.


14. López-Galilea I, Andueza S, Leonardo Id, Paz de Peña M, Cid C. Influence of torrefacto roast on antioxidant and pro-oxidant activity of coffee. Food Chem. 2006. 94:75–80.


15. Artali R, Beretta G, Morazzoni P, Bombardelli E, Meneghetti F. Green tea catechins in chemoprevention of cancer: a molecular docking investigation into their interaction with glutathione S-transferase (GST P1-1). J Enzyme Inhib Med Chem. 2009. 24:287–295.


16. Lee C. Antioxidant ability of caffeine and its metabolites based on the study of oxygen radical absorbing capacity and inhibition of LDL peroxidation. Clin Chim Acta. 2000. 295:141–154.


17. Thong FSL, Derave W, Kiens B, Graham TE, Ursø B, Wojtaszewski JFP, Hansen BF, Richter EA. Caffeine-induced impairment of insulin action but not insulin signaling in human skeletal muscle is reduced by exercise. Diabetes. 2002. 51:583–590.


18. Verhoef P, Pasman WJ, Van Vliet T, Urgert R, Katan MB. Contribution of caffeine to the homocysteine-raising effect of coffee: a randomized controlled trial in humans. Am J Clin Nutr. 2002. 76:1244–1248.


19. Noordzij M, Uiterwaal CSPM, Arends LR, Kok FJ, Grobbee DE, Geleijnse JM. Blood pressure response to chronic intake of coffee and caffeine: a meta-analysis of randomized controlled trials. J Hypertens. 2005. 23:921–928.


20. Park SY. The effect of coffee intake on antioxidative activity and fuel utilization under excised induced oxidative stress in non exercised rats [master's thesis]. 2010. Seoul: Duksung Women's University.
21. Tunnicliffe JM, Erdman KA, Reimer RA, Lun V, Shearer J. Consumption of dietary caffeine and coffee in physically active populations: physiological interactions. Appl Physiol Nutr Metab. 2008. 33:1301–1310.


22. Desbrow B, Hughes R, Leveritt M, Scheelings P. An examination of consumer exposure to caffeine from retail coffee outlets. Food Chem Toxicol. 2007. 45:1588–1592.


23. Lopez-Garcia E, Rodriguez-Artalejo F, Rexrode KM, Logroscino G, Hu FB, van Dam RM. Coffee Consumption and Risk of Stroke in Women. Circulation. 2009. 119:1116–1123.


24. Montavon P, Kukic KR, Bortlik K. A simple method to measure effective catalase activities: Optimization, validation, and application in green coffee. Anal Biochem. 2007. 360:207–215.


25. Gore M, Fiebig R, Hollander J, Griffiths M, Leeuwenburgh C, Ohno H, Ji LL. Exercise training alters antioxidant enzyme gene expression in rat skeletal muscle. Can J Physiol Pharmacol. 1998. 76:1139–1145.


26. McArdle A, Vasilaki A, Jackson M. Exercise and skeletal muscle ageing: cellular and molecular mechanisms. Ageing Res Rev. 2002. 1:79–93.


27. Chang CK, Huang HK, Tseng HF, Hsuuw YD, Tso TK. Interaction of vitamin E and exercise training on oxidative stress and antioxidant enzyme activities in rat skeletal muscle. J Nutr Biochem. 2007. 18:39–45.


28. Ji LL, Stratman FW, Lardy HA. Antioxidant enzyme systems in rat liver, and skeletal muscle: influences of selenium deficiency, chronic training and acute exercise. Arch Biochem Biophys. 1998. 263:150–160.
29. Gomez-Cabrera MC, Domenech E, Viña J. Moderate exercise is an antioxidant: Upregulation of antioxidant genes by training. Free Radic Biol Med. 2008. 44:126–131.


30. Gomez-Cabrera MC, Pallardo FV, Sastre J, Vina J, Garciadel-Moral L. Allopurinol and markers of muscle damage among participants in the Tour de France. JAMA. 2003. 289:2503–2504.


31. Leeuwenburgh C, Heinecke JW. Oxidative stress and antioxidants in exercise. Curr Med Chem. 2001. 8:829–838.


32. Butler PJ, Woakes AJ, Smale K, Roberts CA, Hillidge CJ, Snow DH, Marlin DJ. Respiratory and cardiovascular adjustments during exercise of increasing intensity and during recovery in thoroughbred racehorses. J Exp Biol. 1993. 179:159–180.


34. Kanter MM. Free radicals, exercise and antioxidant supplementation. Proc Nutr Soc. 1998. 57:9–13.


35. Sealy RC, Puzyna W, Kalyanaraman B, Felix CC. Identification by electron spin resonance spectroscopy of free radicals produced during autoxidative melanogenesis. Biochim Biophys Act. 1984. 800:269–276.


36. Sanchez-Quesada JL, Jorba O, Payes A, Otal C, Serra-Grima R, González-Sastre F, Ordóñez-Llanos J. Ascorbic acid inhibits the increase in low-density lipoprotein (LDL) susceptibility to oxidation and the proportion of electronegative LDL induced by intense aerobic exercise. Coron Artery Dis. 1998. 9:249–255.


37. Mills PC, Smith NC, Casas I, Harris P, Harris RC, Marlin DJ. Effects of exercise intensity and environmental stress on indices of oxidative stress and iron homeostasis during exercise in the horse. Eur J Appl Physiol Occup Physiol. 1996. 74:60–66.


38. Gohil K, Viguie C, Stanley WC, Brooks GA, Packer L. Blood glutathione oxidation during human exercise. J Appl Physiol. 1988. 64:115–119.


39. Nagao M, Fujita Y, Wakabayashi K, Nukaya H, Kosuge T, Sugimura T. Mutagens in coffee and other beverages. Environ Health Perspect. 1986. 67:89–91.


40. Yamanaka N, Oda O, Nagao S. Prooxidant activity of caffeic acid, dietary non-flavonoid phenolic acid, on Cu2+ induced low density lipoprotein oxidation. FEBS Lett. 1997. 405:186–190.


41. Azam S, Hadi N, Khan NU, Hadi M. Antioxidant and prooxidant properties of caffeine, theobromine and xanthine. Med Sci Monit. 2003. 9:BR325–BR330.
42. Andueza S, Cid C, Cristina Nicoli M. Comparison of antioxidant and pro-oxidant activity in coffee beverages prepared with conventional and "Torrefacto" coffee. Lebenson Wiss Technol. 2004. 37:893–897.


43. Oh-ishi S, Kizaki T, Nagasawa J, Izawa T, Komabayashi T, Nagata N, Suzuki K, Taniguchi N, Ohno H. Effects of endurance training on superoxide dismutase activity, content and mRNA expression in rat muscle. Clin Exp Pharmacol Physiol. 1997. 24:326–332.


44. Schwartz RS. The independent effects of dietary weight loss and aerobic training on high density lipoproteins and apolipoprotein A-I concentrations in obese men. Metabolism. 1987. 36:165–171.


45. Thelle DS, Arnesen E, Forde OH. The Tromsö Heart Study. Does coffee raise serum cholesterol? N Engl J Med. 1983. 308:1454–1457.
46. Mensink RP, Lebbink WJ, Lobbezoo IE, Weusten-van der Wouw MPME, Zock PL, Katan MB. Diterpene composition of oils from Arabica and Robusta coffee beans and their effects on serum lipids in man. J Intern Med. 1995. 237:543–550.


47. Urgert R, Van Vliet T, Zock PL, Katan MB. Heavy coffee consumption and plasma homocysteine: a randomized controlled trial in healthy volunteers. Am J Clin Nutr. 2000. 72:1107–1110.


48. Wald DS, Law M, Morris JK. Homocysteine and cardiovascular disease: evidence on causality from a meta-analysis. BMJ. 2002. 325:1202–1206.

