Abstract
Catecholamines are among the first molecules that displayed a kind of response to prolonged or repeated stress. It is well established that long-term stress leads to the induction of catecholamine biosynthetic enzymes such as tyrosine hydroxylase (TH) and dopamine β-hydroxylase (DBH) in adrenal medulla. The aim of the present study was to evaluate the effects of ginseng on TH and DBH mRNA expression. Repeated (2 h daily, 14 days) immobilization stress resulted in a significant increase of TH and DBH mRNA levels in rat adrenal medulla. However, ginseng treatment reversed the stress-induced increase of TH and DBH mRNA expression in the immobilization-stressed rats. Nicotine as a ligand of the nicotinic acetylcholine receptor (nAChR) in adrenal medulla stimulates catecholamine secretion and activates TH and DBH gene expression. Nicotine treatment increased mRNA levels of TH and DBH by 3.3- and 3.1-fold in PC12 cells. The ginseng total saponin exhibited a significant reversal in the nicotine-induced increase of TH and DBH mRNA expression, decreasing the mRNA levels of TH and DBH by 57.2% and 48.9%, respectively in PC12 cells. In conclusion, immobilization stress induced catecholamine biosynthetic enzymes gene expression, while ginseng appeared to restore homeostasis via suppression of TH and DBH gene expression. In part, the regulatory activity in the TH and DBH gene expression of ginseng may account for the anti-stress action produced by ginseng.
Stress is a stimulus which affects the body in various ways. The body works to restore homeostasis and suppress physical changes that occur from stress. Excessive stress, when above tolerable limits, can influence body systems, that is, the nervous system [1], immune system [2], and digestive system [3]. In consequence, this disturbs homeostasis, resulting in the development of serious diseases. If the stress continues chronically, it can result in general adaptive symptoms which induce several nonspecific diseases including susceptibility to infection, autoimmune diseases, and chronic fatigue syndrome [4].
When stress continues, it causes reactions through the hypothalamic-pituitary-adrenal (HPA) axis [5]. Corticotrophin releasing hormone (CRH) is secreted at the hypothalamus and the CRH releases adrenocorticotropic hormone (ACTH) from the pituitary gland. The ACTH stimulates the adrenal gland to synthesize and release catecholamines such as corticosterone, epinephrine, dopamine, and norepinephrine which are characteristic stress hormones. Catecholamines are among the first molecules that showed some form of response to stress, and are crucial in stimulating action in response to a perceived threat. Increased level of catecholamines due to the stress stimuli may reflect an increase in the expression of genes encoding catecholamine synthesizing enzymes [6]. The mRNA levels of tyrosine hydroxylase (TH) and dopamine β-hydroxylase (DBH), the major rate-limiting enzymes in catecholamine biosynthesis, were markedly elevated in adrenal medulla of stressed mice [7,8].
Nicotine is a ligand of nicotinic acetylcholine receptor (nAChR) in adrenal medulla and was reported to stimulate catecholamines secretion via increase of expression of catecholamine synthesizing enzymes, TH and DBH, in rat adrenal medulla [9]. PC12 cells, rat pheochromocytoma cells, express TH and DBH genes. The expression of TH and DBH genes was also up-regulated by nicotine treatment in PC12 cells [10].
Recently, the distinct bioactive components in foods have been reported to regulate the protective system of human through affecting the circulatory, endocrine, and immune systems and nerve function. Food research has increasingly focused on analyzing functional properties of foods and their bioactive components. Ginseng (Panax ginseng) has a long history of usage as a traditional medicine in Korea, China, and Japan. Recent studies have presented that ginseng increases resistance to stress, decreases blood pressure, benefits on immune function and exerts antidepressant effects [11-14]. Researches on the anti-stress effects of ginseng suggested ginseng total saponin (GTS) as the active components involved in the alleviation of mental and physical stress and the normalization of work capacity [13-15]. GTS, ginsenosides Rg3 and Rb1 treatment represented an anti-stress effect by attenuating the brain polyamine, putrescine, level in immobilization-stressed mice [15].
Though reports have discussed the anti-stress effects of ginseng or GTS, the effects of ginseng on the TH and DBH gene expression have yet to be clearly elucidated. In the present study, we investigated the anti-stress effects that ginseng produces against immobilization stress in rats. Furthermore, to investigate the anti-stress action of ginseng, we examined the effect of ginseng on the regulation of gene expression of catecholamine biosynthesizing enzymes, TH and DBH, in immobilization stressed rats in vivo. In addition, we utilized PC 12 cells to identify the direct effect of GTS on TH and DBH gene expression.
The ginseng for animal treatment was powdered using an extruding machine and supplied by Korea Food Research Institute [16]. The ginseng total saponin (GTS) was used for PC12 cell treatment and generously provided by Korea Food Research Institute [16]. The GTS was extracted from 4-year-old ginseng (Panax ginseng) roots. It contained major glycosides (Rg3 40%, Rf1 52.7%) and other glycosides and components (7.3%) as determined by high performance liquid chromatography methods [16].
Male Sprague-Dawley rats, 7 weeks of age, were purchased from Charles River (Hino, Japan) and housed in an environmentally controlled animal laboratory and maintained at 22 ± 1℃ with a 12 hr light: dark cycle (light period: 08:00-20:00 hr) throughout the experimental period. After acclimatization for 1 week in the laboratory environment, they were randomly divided into three groups of 6 animals: control (C), immobilization stress (S), and ginseng with immobilization stress (GS). For immobilization stress experiments, rats were immobilized in hemi-cylindrical plastic tube for 2 hr (09:00-11:00 hr). Repeated stress was achieved by immobilizing the animals for 2 consecutive weeks, 2 hr daily. Controls were unhandled. Ginseng (200 mg/kg BW) was administrated after the application of immobilization stress. Ginseng was dissolved in 3 ml of water for oral administration. All animal procedures conformed to NRC guidelines as stated in the 'Principles of Laboratory Animal Care' [17].
At the end of the experiment, animals were anesthetized and a central longitudinal incision was made into the abdominal wall, and blood samples were collected by cardiac puncture. Blood samples were centrifuged at 1500 ×g for 20 min at 4℃ and the serum was separated and stored at -20℃. The adrenal glands were dissected, rinsed with saline, frozen immediately in liquid nitrogen, and stored at -70℃ until analyzed. The serum corticosterone level was measured using a commercially available RIA kit (Coat-A-Count TKRC1, USA).
PC12 cells were cultured in RPMI-1640 medium (Gibco, USA) supplemented with 10% (v/v) horse serum (Gibco, USA), 5% (v/v) fetal bovine serum (Gibco, USA), 10 mM glutamine, 100 U/ml penicillin, and 100 µg/ml streptomycin at 37℃ under the condition of 5% CO2. The cells were divided into three groups; control (C), nicotine (N), and GTS plus nicotine (GN). PC12 cells were treated with 10 µM nicotine in the presence or absence of GTS for 12 hr. The GTS was dissolved with distilled water and the final concentration in culture medium was 10 µg/ml. The control cells were cultured without nicotine and GTS. All treatments were performed three times in triplicate.
Total RNA was extracted from adrenal glands and PC12 cells using TRIzol Reagent (Promega, Madison, USA). The cDNAs were synthesized from 4 µg of RNA using M-MLV reverse transcriptase (Promega). After cDNA synthesis, quantitative real-time PCR was performed in 25 µl of Universal SYBR Green PCR Master Mix (Qiagen, Chatsworth, USA) using a fluorometric thermal cycler (Rotor-GeneTM 2000, Corbett Research, Australia). Reaction mixtures were incubated for an initial denaturation at 95℃ for 10 min, which was followed by 50 PCR cycles. Each cycle was performed under temperatures of 95℃ for 10 seconds, 55℃ for 20 seconds, and 72℃ for 20 seconds, respectively. Primers were designed using an on-line program (primer3; http://frodo.wi.mit.edu/) [18]. The sequences of the sense and antisense primers were as listed below: TH, 5'-TAAGTGGTGAATTTTGGCTT-3' and 5'-GCTGTGGTATTTGAGGAGAG-3'; DBH, 5'-CCAGGATCCCATACACTAGA-3' and 5'-CTGGATACCCATCAGGACTA-3'; β-actin, 5'-GTTGCCAATAGTGATGACCT-3' and 5'-GGACCTGACAGACTACCTCA-3'. The Δ CT method was used to measure relative quantification. Values were expressed as the fold-change over control.
Values were expressed as mean ± SE. Significant differences among groups were analyzed by one-way ANOVA using the SPSS version 12.0 (SPSS Inc., Chicago, USA). Differences were considered statistically significant at P < 0.05. Where ANOVA showed significance, differences among groups were evaluated by Tukey's multiple range test.
Body weight was significantly decreased in the immobilization-stressed group by 15.5% compared to control group (P < 0.05) (Fig. 1). The percentage of rats with immobilization stress that gained weight accounted up to only 37.7% of control group. The ginseng administrated group had a significant reversal in the stress-induced decrease in body weight compared to immobilization stressed group (P < 0.05) (Fig. 1).
Fig. 2 depicts hormonal responses to immobilization stress. Immobilization stress for 2 wks resulted in 9-fold increase in serum corticosterone concentration compared to the hormone level in non-stressed control group (Fig. 2). The ginseng-administered group exhibited a significant reversal in the stress-stimulated serum corticosterone level, decreasing by 50% compared to immobilization stressed group (P < 0.05) (Fig. 2).
Immobilization stress for 2 wks produced 3.3- and 3.1-fold stimulation of adrenal TH and DBH mRNA levels compared to control group (Fig. 3A, 3B). The ginseng administered group exhibited a significant reversal in the stress-induced gene expression of TH and DBH, decreasing the mRNA levels of TH and DBH by 35.6 and 48.2%, respectively, compared to the immobilization stressed group (P < 0.05) (Fig. 3A, 3B).
Nicotine treatment increased mRNA levels of TH and DBH by 3.3- and 3.1-fold, respectively, compared to control cells (Fig. 4A, 4B). GTS exhibited a significant reversal in the nicotine-induced gene expression of TH and DBH, decreasing the mRNA levels of TH and DBH by 57.2% and 48.9%, respectively, compared to cells treated with nicotine.
Ginseng is one of the most popular medicinal plants throughout the world because of its beneficial effects. Ginseng is a well-known adaptogen, but the mechanism of anti-stress activity has yet to be clearly elucidated. In the previous study [11], we reported the decrease in body weight, food intake, and tissue weights of spleen and thymus in immobilization-stressed rats, and that ginseng administration reversed stress-induced changes in body weight, food intake, and tissue weights in stressed rats, suggesting anti-stress potential of ginseng. In this study, we investigated the plausible mechanism of anti-stress action of ginseng using immobilization-stressed rats and PC 12 cells.
Stress stimulated the secretion of catecholamines from the adrenal gland [5]. The catecholamines speeded up the catabolism of protein and degradation of triglyceride from adipose tissue and decreased appetite, resulted in the decrease of body weight [19,20]. In our experiment, the body weight and food intake in the immobilization stressed group were decreased as reported by previous studies [11,20,21]. Administration of ginseng significantly reversed stress-induced body weight loss in this study. Kim [22] reported that the rats treated with ginseng had less weight loss compared to the control group, when they were exposed to the external stress.
Increased serum corticostrone level in immobilization stress is due to the activation of the hypothalamic-pituitary-adrenal (HPA) axis, which is highly responsive to stress and is one of the principal mechanism by which an organism mobilizes its defense against stress events [5]. In our study, the serum cortocostrone level in immobilization stressed group was markedly increased, as reported by previous studies [11,23]. Ginseng administration significantly reduced stress-increased serum corticosterone level, clearly demonstrating its anti-stress potential.
It is generally accepted that TH, catalyzing tyrosine to dihydroxyphenylacetic acid, is the rate limiting enzyme in the biosynthesis of catecholamines. Dihydroxyphenylacetic acid is immediately decarboxylated to the neurotransmitter dopamine. Addition of a hydroxyl group to the beta-carbon of the aliphatic chain by dopamine b-hydroxylase (DBH) generates norepinephrine, and finally, norepinephrine is converted to epinephrine by PNMT [24]. It has been revealed that several catecholaminergic regions respond to a variety of stressors with increased levels of mRNAs that encode catecholamine-synthesizing enzymes such as TH and DBH [6]. In the present study, the mRNA levels of TH and DBH were markedly increased by immobilization stress in rat adrenal glands. Ginseng administration suppressed the stress-induced TH and DBH gene expression in immobilization stressed rats. These results support the hypothesis that ginseng inhibited catecholamine biosynthesis against immobilization stress via down-regulation of TH and DBH gene expression.
We also investigated the direct effects of ginseng on TH and DBH gene expression using in vitro model, PC12 cells. PC12 pheochromocytoma cell line is a clone of cells derived from rat adrenal medullary tumor. PC12 cells were derived exhibiting many properties of adrenal medullary chromaffin cells, including the synthesis, storage, and secretion of catecholamines. These cells are therefore useful for studies of environmental factors that modulate catecholaminergic functions [25]. It was demonstrated that the expressions of TH and DBH genes was increased by nicotine treatment in PC12 cells [10]. In the present study, induction of both TH and DBH mRNAs was observed after treatment with nicotine in PC12 cells. GTS down-regulated the nicotine-induced increase of TH and DBH mRNA levels in PC12 cells. These results suggested that direct inhibitory effects on catecholamine biosynthesis may be involved in the anti-stress effects of ginseng.
In peripheral systems, catecholamines act as hormones and neurotransmitters in the adrenal medulla and sympathetic nerve terminals respectively, and are secreted in large quantities under stressful conditions [24]. The organs that are responsive to those transmitters are stimulated to cope with stress. Long-term or excessive stress results in the over-secretion of catecholamines, leading to over-reaction and exhaustion of the organs. For example, a persistent high level of corticosterone during chronic stress causes apoptosis and necrosis in immature T and B cells, resulting in the decline of immune function [26]. Furthermore, in autonomic nervous systems, the stress affects sympathetic nervous systems, resulting in metabolic disorders [27]. It is possible that ginseng suppressed catecholamine synthesis induced under stressful conditions via the reduction of catecholamine, synthesizing enzymes gene expression and leading to the recovery of homeostasis. In part, these effects may account for the anti-stress mechanism of ginseng.
On the basis of the above results, it is suggested that the extract of ginseng had potent anti-stress activity that can normalize the stress-induced changes in body weight gain, blood hormone, and catecholamine synthesizing enzymes gene expression, thus helping the restoration of the body's homeostasis. Furthermore, the present study suggests that GTS, one of the major ginseng components, can be postulated as a potential mediator, showing the regulation of catecholamine synthesizing gene expression in PC12 cells.
Figures and Tables
Fig. 1
Effect of ginseng on body weights in immobilization stressed rats. The groups are control (C), immobilization stress (S), and ginseng with immobilization stress (GS). The immobilization stress was induced daily for 2 hr of each day for 2 weeks. The ginseng with immobilization stress group was treated with ginseng (200 mg/kg BW) dissolved in water after the application of immobilization stress. Results are represented as mean ± SE (n = 6). Values with different superscript letter are significantly different, P < 0.05.
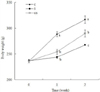
Fig. 2
Effect of ginseng on serum corticosterone levels in immobilization stressed rats. The serum corticosterone levels were measured as described in Materials and Methods. Each bar represents the mean ± SE in each group; control (C), immobilization stress (S), and ginseng with immobilization stress (GS). Results are represented as mean ± SE (n = 6). Values with different superscript letter are significantly different, P < 0.05.
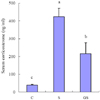
Fig. 3
Effect of ginseng on the expression of genes involved catecholamine biosynthesis in adrenal gland. Total RNA extracted from adrenal gland was used for mRNA expression analysis of TH (A) and DBH (B) by quantitative real-time PCR. The groups are control (C), immobilization stress (S), and ginseng with immobilization stress (GS). The immobilization stress was induced daily for 2 hr of each day for 2 weeks. The ginseng with immobilization stress group was treated with ginseng (200 mg/kg BW) dissolved in water after the application of immobilization stress. Values are expressed as the fold-change over control as mean ± SE (n = 4). Values with different superscript letter are significantly different, P < 0.05.
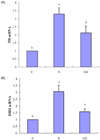
Fig. 4
Effect of GTS on the expression of genes involved catecholamine biosynthesis in PC12 cells. The groups are control (C), nicotine (N), GTS with nicotine (GN). PC12 cells were treated with 10 uM nicotine in the presence or absence of 10 µg/ml GTS for 12h. The mRNAs of TH (A) and DBH (B) were measured by quantitative real-time RT-PCR. Values are expressed as the fold-change over control and as the mean ± SE (n = 3). Values with different superscript letter are significantly different, P < 0.05.
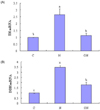
References
1. Chrousos GP. The role of stress and the hypothalamic-pituitary-adrenal axis in the pathogenesis of the metabolic syndrome: neuro-endocrine and target tissue-related causes. Int J Obes Relat Metab Disord. 2000. 24:S50–S55.


2. Khansari DN, Murogo AJ, Faith RE. Effects of stress on the immune system. Immunol Today. 1990. 11:170–175.


3. Staratakis CA, Chrousos GP. Neuroendocrinology and pathophysiology of the stress system. Ann N Y Acad Sci. 1995. 771:1–18.


4. Serova LI, Gueorguiev V, Cheng SY, Sabban EL. Adrenocorticotropic hormone elevates gene expression for catecholamine biosynthesis in rat superior cervical ganglia and locus coeruleus by an adrenal independent mechanism. Neuroscience. 2008. 153:1380–1389.


5. Dunn AJ, Kramarcy NR. Iversen LL, Iversen SD, Snyder SH, editors. Neurochemical responses in stress: relationships between the hypothalamo-pituitary-adrenal and catecholamine system. Handbook of psychopharmacology. 1984. vol 18. New York: Plenum Press;455–479.
6. Sabban EL, Kvetnanský R. Stress-triggered activation of gene expression in catecholaminergic systems: dynamics of transcriptional events. Trends Neurosci. 2001. 24:91–98.


7. Kvetnansky R, Micutkova L, Rychkova N, Kubovcakova L, Mravec B, Filipenko M, Sabban EL, Krizanova O. Quantitative evaluation of catecholamine enzymes gene expression in adrenal medulla and sympathetic Ganglia of stressed rats. Ann N Y Acad Sci. 2004. 1018:356–369.


8. Kubovcakova L, Tybitanclova K, Sabban EL, Majzoub J, Zorad S, Vietor I, Wagner EF, Krizanova O, Kvetnansky R. Catecholamine synthesizing enzymes and their modulation by immobilization stress in knockout mice. Ann N Y Acad Sci. 2004. 1018:458–465.


9. Sterling CR, Tank WA. Adrenal tyrosine hydroxylase activity and gene expression are increased by intraventricular administration of nicotine. J Pharmacol Exp Ther. 2001. 296:15–21.
10. Hiremagalur B, Nankova B, Nitahara J, Zeman R, Sabban EL. Nicotine increases expression of tyrosine hydroxylase gene. J Biol Chem. 1993. 268:23704–23711.
11. Choi EH, Lee HJ, Kim CJ, Kim JT, Kwun IS, Kim Y. Anti-stress effects of ginseng in immobilization-stressed rats. Journal of Food Science and Nutrition. 2004. 9:253–258.


12. Jeon BH, Kim CS, Kim HS, Park JB, Nam KY, Chang SJ. Effect of Korean red ginseng on blood pressure and nitric oxide production. Acta Pharmacol Sin. 2000. 21:1095–1100.
13. Kim DH, Moon YS, Lee TH, Jung JS, Suh HW, Song DK. The inhibitory effect of ginseng saponins on the stress-induced plasma interleukin-6 level in mice. Neurosci Lett. 2003. 353:13–16.


14. Dang H, Chen Y, Liu X, Wang Q, Wang L, Jia W, Wang Y. Antidepressant effects of ginseng total saponins in the forced swimming test and chronic mild stress models of depression. Prog Neuropsychopharmacol Biol Psychiatry. 2009. 33:1417–1424.


15. Lee SH, Jung BH, Kim SY, Lee EH, Chung BC. The antistress effect of ginseng total saponin and ginsenoside Rg3 and Rb1 evaluated by brain polyamine level under immobilization stress. Pharmacol Res. 2006. 54:46–49.


16. Jee HK, Cho YJ, Kim CT, Jang YS, Kim CJ. Increase of solubility of ginseng radix by extrusion cooking. Korean Journal of Food Science and Technology. 2006. 38:361–368.
17. National Reseach Council. Guide for the care and use of laboratory animals. 1996. 7th ed. Washington, D.C.: National Academy press;128.
18. Rozen S, Skaletsky HJ. Biology KS, Misener S, editors. Primer3 on the WWW for general users and for biologist programmers. Bioinformatics Methods and Protocols: Methods in Molecular. 2000. New Jersey: Humana Press;365–386.


19. Weissman C. The metabolic response to stress: an overview and update. Anesthesiology. 1990. 73:308–327.
20. Rybkin II, Zhou Y, Volaufova J, Smagin GN, Ryan DH, Harris RB. Effect of restraint stress on food intake and body weight is determined by time of day. Am J Physiol. 1997. 273:R1612–R1622.
21. Harris RB, Zhou J, Youngblood BD, Rybkin II, Smagin GN, Ryan DH. Effect of repeated stress on body weight and body composition of rats fed low- and high-fat diets. Am J Physiol. 1998. 275:R1928–R1938.
22. Kim CC. Influence of Panax ginseng on the response of stressful stimuli in the experimental animal exposed to various stress. Korean Journal of Ginseng Science. 1979. 3:168–186.
23. Hauger RL, Lorang M, Irwin M, Aguilera G. CRF receptor regulation and sensitization of ACTH responses to acute ether stress during chronic intermittent immobilization stress. Brain Res. 1990. 532:34–40.


24. Wong DL, Tank AW. Stress-induced catecholaminergic function: transcriptional and post-transcriptional control. Stress. 2007. 10:121–130.


25. Tischler AS, Perlman RL, Morse GM, Sheard BE. Glucocorticoids increase catecholamine synthesis and storage in PC 12 pheochromocytoma cell cultures. J Neurochem. 1983. 40:364–370.


26. Dhabhar FS, Miller AH, McEwen BS, Spencer RL. Effects of stress on immune cell distribution. Dynamics and hormonal mechanisms. J Immunol. 1995. 154:5511–5527.
27. Nonogaki K, Iguchi A. Stress, acute hyperglycemia, and hyperlipidemia: Role of the autonomic nervous system and cytokines. Trends Endocrinol Metabol. 1997. 8:192–197.