Abstract
There is an increasing interest in curcumin (Curcuma longa L.) as a cardiovascular disease (CVD) protective agent via decreased blood total cholesterol and low-density lipoprotein-cholesterol (LDL-cholesterol) level. The aim of this study was to investigate further the potential mechanism in the hypocholesterolemic effect of curcumin by measuring cholesterol 7a-hydroxylase (CYP7A1), a rate limiting enzyme in the biosynthesis of bile acid from cholesterol, at the mRNA level. Male Sprague-Dawley rats were fed a 45% high fat diet or same diet supplemented with curcumin (0.1% wt/wt) for 8 weeks. The curcumin diet significantly decreased serum triglyceride (TG) by 27%, total cholesterol (TC) by 33.8%, and LDL-cholesterol by 56%, respectively as compared to control group. The curcumin-supplemented diet also significantly lowered the atherogenic index (AI) by 48% as compared to control group. Hepatic TG level was significantly reduced by 41% in rats fed with curcumin-supplemented diet in comparison with control group (P < 0.05). Conversely, the curcumin diet significantly increased fecal TG and TC. The curcumin diet up-regulated hepatic CYP7A1 mRNA level by 2.16-fold, compared to control group p (P < 0.05). These findings suggested that the increases in the CYP7A1 gene expression may partially account for the hypocholesterolemic effect of curcumin.
Cardiovascular disease (CVD) is one of the main causes of mortality globally. The World Health Organization (WHO) has predicted an increase in prevalence of CVD, with projected combined death toll of 24 million by 2030 [1]. An increased blood cholesterol level, which is governed by cholesterol absorption, synthesis, storage, and excretion, is one of the major risk factors leading to the development of CVD [2]. The conversion of cholesterol to bile acids in the liver is an important pathway for the elimination of cholesterol from the body [3]. Cholesterol 7a-hydroxylase (CYP7A1) is a liver-specific enzyme that catalyzes the rate-limiting step in the biosynthesis of bile acid from cholesterol.
The need to decrease plasma lipids has been recognized and dietary control of blood cholesterol has been explored [4]. Plants and phytochemicals have been shown to exert CVD protective effects in epidemiological analysis, animal experiments, and clinical trials [4-7]. These effects could be due to plant polyphenolic compounds that possess an aromatic ring bearing one or more hydroxyl groups [8].
Curcuma longa L. (turmeric), which belongs to the Zingiberacae family [9], is a perennial plant that has been widely used as spice, coloring adjuncts and also as a major ingredient of curry powder [10]. Tumeric has a long history of use as traditional medicines of China and India [11], reflecting the compound's diverse and beneficial health effects. Curcumin is a phenolic compound present in the turmeric roots. Commercially available turmeric powders contain 2.71-5.18g of curcumin per 100g with 100 g curry powder typically containing 0.34-0.47g curcumin [12]. Several intervention studies using animal models have showed that curcumin has a hypocholesterolemic effect [13,14]. A plausible explanation for curcumin-mediated lowered concentration of blood cholesterol involves stimulated conversion of cholesterol to bile acids via increase of cholesterol 7a-hydroxylase (CYP7A1) enzyme activity [15].
The conversion of cholesterol to bile acids in the liver is an important pathway for the elimination of cholesterol from the body [3]. Cholesterol 7a-hydroxylase (CYP7A1) is a liver-specific enzyme that catalyzes the rate-limiting step in the biosynthesis of bile acid from cholesterol in liver. The objective of this study was to elucidate the molecular details of the hypocholesterolemic effect of curcumin. This regulation could be explained by alterations of the expression of gene that is involved in cholesterol homeostasis in the body. The previous studies mainly indicated the hypocholesterolemic role of curcumin at the enzyme activity level [15]. We hypothesized that curcumin reduces concentration of blood cholesterol via induction of the CYP7A1 gene expression.
Curcumin was obtained from Sigma-Aldrich (St Louis, MO, USA). All other chemicals were of the highest pure grade available.
Four-week-old male Sprague-Dawley rats (SLC, Japan) were randomly assigned to two groups (n = 6) after 1 week of adaption for metabolic stabilization. The animals were all individually housed in stainless steel cages in a room kept at 22 ± 1℃ with a 12 h -hour light/dark cycle. Experimental diets employed were the modified AIN93 diet (Dyets, Bethlehem, PA). All diets contained 45% high fat in order to induce hypercholesterolemia. Rats were maintained on a curcumin-free diet and a diet with 0.1% (w/w) curcumin for 8 weeks. The body weight and energy intake were measured twice a week. All animal procedures conformed to NIH guidelines as stated in the "Principles of Laboratory Animal Care" [16].
At the end of the experiment, animals were anesthetized and a central longitudinal incision was made into the abdominal wall. Blood samples were collected by cardiac puncture. Liver samples were excised, immediately frozen in liquid nitrogen and stored at -70℃. The feces were collected on week 8 for 3 days and dried.
Serum concentrations of triglyceride (TG), total cholesterol (TC), high-density lipoprotein cholesterol (HDL-C), and activities of glutamic-oxaloacetic transaminase (GOT) and glutamate-pyruvate transaminase (GPT) were measured by an enzymatic colorimetric method [17] using a commercial enzymatic kit (Asan Pharmaceutical, Seoul, Korea). Low density lipoprotein cholesterol (LDL-C) was calculated as: LDL-C = TC-[(HDL-C-TG)/2] [18]. The atherogenic index (AI) was calculated as: AI = (TC-HDL-C)/HDL-C [19].
Total RNA was isolated from liver tissue using Trizol reagent (Invitrogen, Calsbad, USA). The cDNAs were synthesized from 4 µg total RNA using M-MLV reverse transcriptase (Invitrogen). After cDNA synthesis, quantitative real-time PCR was performed in 25 µL QuantiTect SYBR Green PCR Kit solution (Qiagen, Valencia, CA, USA) using a Rotor gene 3000 (Corbett Research, Mortlake, Australia). The reaction mixture was incubated for an initial denaturation at 95℃ for 15 min, followed by 40 cycles of 95℃ for 15 s, 60℃ for 60 s and 72℃ for 30 s. Primers were designed using an on-line program (Primer3; http://frodo.wi.mit.edu/) [21]. Primers for rat CYP7A1 were forward: 5'-TGC TCT GTG TTC ACT TTC TG-3', reverse: 5'-ACT CGG TAA CAG AAG GCA TA-3' and for rat β-actin were forward: 5'-GGC ACC ACA CTT TCT ACA AT-3', reverse: 5'-AGG TCT CAA ACA TGA TCT GG-3'. The ΔCT method was used to measure relative quantification. The ΔCT value for each sample was determined by calculating the difference between the CT value of the target gene and the CT value of the β-actin reference gene. Values were expressed as fold-change over control and expressed as the mean ± SE (n = 6). All PCR products were separated by electrophoresis using 1.5% agarose gel stained with ethidium bromide and visualized under ultraviolet light.
All data are expressed as mean ± SE. The statistical significance of difference between the control and curcumin-treated groups was analyzed by Student's t-test using the SPSS package program version 17.0 (SPSS, Chicago, IL, USA). The results were considered significant at P < 0.05.
Rats fed the curcumin-supplemented diet showed a significantly decreased serum TG level by 27% in comparison with control group (P < 0.05) (Table 1). The curcumin diet also significantly lowered serum TC and LDL-C by 34% and 68%, respectively, compared to the control group (P < 0.05) (Table 1). Serum HDL-C level was not affected by curcumin supplementation (Table 1). In addition, AI was significantly decreased in dietary curcumin supplemented rats by 48% in comparison with control group (P < 0.05) (Fig. 2A). The serum activities of glutamic-oxaloacetic transaminase (GOT) and glutamate-pyruvate transaminase (GPT) were within the normal range for rats, showing 67-72 IU/l and 16-21 IU/l, respectively (Fig. 2B). No significant diet-related differences were shown between groups.
Hepatic TG level was significantly reduced by 40% in rats fed with curcumin-supplemented diet in comparison with control group (P < 0.05) (Table 2). There was no difference in hepatic TC level between groups. Fecal TG and cholesterol concentrations were significantly increased by 34% and 17%, respectively in rats fed with curcumin-supplemented diet as compared with the control group (P < 0.05) (Table 2).
Real-time RT-PCR determined that consumption of curcumin resulted in a significantly elevated mRNA level of hepatic CYP7A1 by 2.16-fold compared to the control group (P < 0.05) (Fig. 3).
The observations in human [7] and animal studies [22] showed an inverse association between curcumin supplementation and the concentrations of serum cholesterol and triglyceride. Administration of 500 mg curcumin to human volunteers for 1 week did produce significant change in serum cholesterol level, with curcumin tending to lower serum cholesterol and TG levels [7]. Contrary to these results, consumption of curcumin over 1 month or 6 months was reported not to affect blood concentrations of TG, or TC, LDL, and HDL-cholesterol [23]. Thus, we investigated the hypochlestrolemic effect of curcumin in high-fat diet treated rats. Furthermore, to investigate the mechanism of the hypochlestrolemic action of curcumin, we examined the effect of curcumin on the regulation of gene expression of cholesterol 7a-hydroxylase (CYP7A1), a rate limiting enzyme in bile acid biosynthesis from cholesterol.
The induction of hypercholesterolemia in experimental rats or mice by feeding a high-cholesterol or high-fat diet has been widely suggested as a reliable model to study hypochlestrolemic effect of curcumin [14,15,22]. The 0.1% (w/w) dose of curcumin used in animal studies, including the present study, roughly corresponds to twice the calculated dietary intake of curcumin in a study conducted in India [24]. This is likely not a concern, since studies indicate that consumption of up to 12 g of curcumin daily is not harmful or toxic to human and animals [24]. In our study, a diet enriched with curcumin showed a significant decrease in the levels of serum TG, TC, and LDL-C as reported by previous studies [14,15,22]. In addition, curcumin significantly decreased the AI, which is an important risk index for CVD, clearly demonstrating hypocholesterolemic potential of curcumin.
In this study, curcumin significantly decreased hepatic TG level while there was no reduction in cholesterol level. Similarly, another study reported that 0.2% curcumin treatment significantly reduced hepatic TG but not cholesterol level [25]. Our experiments showed that fecal TG and cholesterol levels were greater in rats fed with the curcumin-supplemented diet than the control diet, reflecting that cholesterol may bind to curcumin and be excreted. These results are consistent with the previous study reporting the curcumin-mediated increase in fecal excretion of cholesterol and bile acid [26]. It was suggested that curcumin may prevent the absorption of cholesterol and lipids by disrupting micelle formation and promote fecal excretion of total steroids and bile acids [27].
When excretion of bile acids increases, conversion of cholesterol to bile acids in the liver will be enhanced in order to replenish the loss in bile acids. Conversion of cholesterol to bile acids is the major pathway of cholesterol elimination and accounts for about 50% of daily cholesterol excretion [28]. Curcumin was reported to decrease the serum cholesterol via enhanced CYP7A1 enzyme activity, which controls cholesterol homeostasis [15]. In our study, rats consuming the curcumin diet showed a significant increase in hepatic CYP7A1 mRNA level. Curcumin treatment was reported to up-regulate the LDL-receptor mRNA in human hepatoma HepG2 cells [29] suggesting that curcumin regulates expression of genes involved in cholesterol homeostasis. Overall, the increase in hepatic CYP7A1 gene expression may be a mechanism that partially accounts for the hypocholesterolemic effect of curcumin. Curcumin stimulated the activation and expression of AMP-activated protein kinase and peroxisome proliferator-activated receptor involved in adipocyte energy metabolism in subcutaneous adipose tissue of mice fed a curcumin-supplemented high fat diet [22]. It would be of interest to further investigate the signal pathway related CYP7A1 gene expression that curcumin might involve. In conclusion, curcumin significantly decreased serum total- and LDL-cholesterol level and triglyceride level. Also, curcumin enhanced hepatic CYP7A1 gene expression. These results suggested that increased CYP7A1 gene expression may partially account for the role in the cholesterol lowering effect of curcumin.
Figures and Tables
Fig. 1
Effect of dietary curcumin supplementation on body weight. Rats were fed a 45% high-fat diet with or without curcumin (0.1% w/w) for 8 weeks. Body weight (A) and food intake were measured twice per week. EER (B) was calculated as; body weight gain (g)/food intake (g). Data are expressed as mean ± SEM (n = 6).
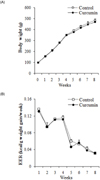
Fig. 2
Effect of dietary curcumin supplementation on atherpgenic index and serum activities of GOT and GPT. Rats were fed a 45% high-fat diet with or without curcumin (0.1% w/w) for 8 weeks. Data are expressed as mean ± SEM (n = 6). Serum lipids were measured by an enzymatic colorimetric method. The atherogenic index (A) was calculated as: AI = (TC-HDL-C) / HDL-C. The serum activities of glutamic-oxaloacetic transaminase (GOT) and glutamate-pyruvate transaminase (GPT) (B) were measured by an enzymatic colorimetric method. *Means significantly different from control group at P < 0.05.
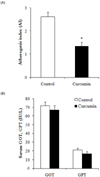
Fig. 3
Effects of dietary curcumin supplementation on hepatic CYP7A1 mRNA expression. Rats were fed a 45% high-fat diet with or without curcumin (0.1% w/w) for 8 weeks. Total RNA was extracted from liver and the CYP7A1 mRNA was measured by quantitative real-time PCR. Values are expressed as the fold-change over control as mean ± SEM (n = 6). *Means significantly different from control group at P < 0.05.
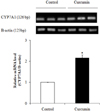
References
1. Rosamond W, Flegal K, Furie K, Go A, Greenlund K, Haase N, Hailpern SM, Ho M, Howard V, Kissela B, Kittner S, Lloyd-Jones D, McDermott M, Meigs J, Moy C, Nichol G, O'Donnell C, Roger V, Sorlie P, Steinberger J, Thom T, Wilson M, Hong Y. American Heart Association Statistics Committee and Stroke Statistics Subcommittee. Heart disease and stroke statistics-2008 update: a report from the American Heart Association Statistics Committee and Stroke Statistics Subcommittee. Circulation. 2008. 117:e25–e146.
2. Assamann G, Cullen P, Jossa F, Lewis B, Macini M. Coronary heart disease: reducing the risk: the scientific background to primary and secondary prevention of coronary heart disease. Arterioscler Thromb Vasc Biol. 1999. 19:1819–1824.
3. Zhang Z, Wang H, Jiao R, Peng C, Wong YM, Yeung VS, Huang Y, Chen ZY. Choosing hamsters but not rats as a model for studying plasma cholesterol-lowering activity of functional foods. Mol Nutr Food Res. 2009. 53:921–930.


4. Yang L, Kadowaki M. Effects of rice proteins from two cultivars, Koshihikari and Shunyo, on hepatic cholesterol secretion by isolated perfused livers of rats fed cholesterol-enriched diets. Ann Nutr Metab. 2009. 54:283–290.


5. Tucker KL. Dietary intake and coronary heart disease: a variety of nutrients and phytochemicals are important. Curr Treat Options Cardiovasc Med. 2004. 6:291–302.


6. Vincent HK, Bourguignon CM, Taylor AG. Relationship of the dietary phytochemical index to weight gain, oxidative stress and inflammation in overweight young adults. J Hum Nutr Diet. 2010. 23:20–29.


7. Soni KB, Kuttan R. Effect of oral curcumin administration on serum peroxides and cholesterol levels in human volunteers. Indian J Physiol Pharmacol. 1992. 36:273–275.
8. Halliwell B. Dietary polyphenols: Good, bad, or indifferent for your health? Cardiovas Res. 2007. 73:341–347.


9. Araújo CC, Leon LL. Biological activities of Curcuma longa L. Mem Inst Oswaldo Cruz. 2001. 96:723–728.


10. Asai A, Miyazawa T. Dietary curcuminoids prevent high-fat diet-induced lipid accumulation in rat liver and epididymal adipose tissue. J Nutr. 2001. 131:2932–2935.


11. Beynen AC, Visser JJ, Schouten JA. Inhibitory effect on lithogenesis by ingestion of a Curcuma mixture (Temoe Lawak Singer). Journal of Food Science and Technology. 1987. 24:253–256.
12. Hiserod R, Hartman TG, Ho CT, Rosen RT. Characterization of powdered turmeric by liquid chromatography-mass spectrometry and gas chromatography-mass spectrometry. J Chromatogr. 1996. 740:51–63.


13. Manjunatha H, Srinivasan K. Hypolipidemic and antioxidant effects of curcumin and capsaicin in high-fat-fed rats. Can J Physiol Pharmacol. 2007. 85:588–596.


14. Asai A, Miyazawa T. Dietary curcuminoids prevent high-fat diet-induced lipid accumulation in rat liver and epididymal adipose tissue. J Nutr. 2001. 131:2932–2935.


15. Babu PS, Srinivasan K. Hypolipidemic action of curcumin, the active principle of turmeric (Curcuma longa) in streptozotocin induced diabetic rats. Mol Cell Biochem. 1997. 166:169–175.
16. National Institute of Health. Guide for the care and use of laboratory animals. 1996. Washington DC: National Academic Press;21–55.
17. Sale FO, Marchesini S, Fishman PH, Berra B. A sensitive enzymatic assay for determination of cholesterol in lipid extracts. Anal Biochem. 1984. 142:347–350.


18. Friedewald WT, Levey RI, Fredrickson DS. Estimation of the concentration of low-density lipoprotein cholesterol in plasma, without use of the preparative ultracentrifuge. Clin Chem. 1972. 18:499–502.


19. Rosenfeld L. Lipoprotein analysis. Early methods in the diagnosis of atherosclerosis. Arch Pathol Lab Med. 1989. 113:1101–1110.
20. Bligh EG, Dyer WJ. A rapid method of total lipid extraction and purification. Can J Physiol Pharmacol. 1959. 37:911–917.


21. Rozen S, Skaletsky HJ. Krawetz S, Misener S, editors. Primer3 on the WWW for general users and for biologist programmers. Bioinformatics methods and protocols: Methods in molecular biology. 2000. New Jersey: Humana Press;365–386.


22. Ejaz A, Wu D, Kwan P, Meydani M. Curcumin inhibits adipogenesis in 3T3-L1 adipocytes and angiogenesis and obesity in C57/BL Mice. J Nutr. 2009. 139:919–925.


23. Baum L, Cheung SK, Mok VC, Lam LC, Leung VP, Hui E, Ng CC, Chow M, Ho PC, Lam S, Woo J, Chiu HF, Goggins W, Zee B, Wong A, Mok H, Cheng WK, Fong C, Lee JS, Chan MH, Szeto SS, Lui VW, Tsoh J, Kwok TC, Chan IH, Lam CW. Curcumin effects on blood lipid profile in a 6-month human study. Pharmacol Res. 2007. 56:509–514.


24. Thimmayamma BVS, Rau P, Radhaiah G. Use of spices and condiments in the dietaries of urban and rural families. Indian J Nurt Diet. 1983. 20:153–162.
25. Manjunatha H, Srinivasan K. Protective effect of dietary curcumin and capsaicin on induced oxidation of low-density lipoprotein, iron-induced hepatotoxicity and carrageenan-induced inflammation in experimental rats. FEBS J. 2006. 273:4528–4537.


26. Rao DS, Sekhara NC, Satyanarayana MN, Srinivasan M. Effect of curcumin on serum and liver cholesterol levels in the rat. J Nutr. 1970. 100:1307–1316.


27. Srinivasan K, Sambaiah K. The effect of spices on cholesterol 7 alpha-hydroxylase activity and on serum and hepatic cholesterol levels in the rat. Int J Vitam Nutr Res. 1991. 61:364–369.