Abstract
It has been reported that dietary polyunsaturated fats (PUFA) increase liver injury in response to ethanol feeding. We tested the hypothesis that diets rich in linoleic acid (18:2n-6) would affect acute liver injury after acetaminophen injection and that protein restriction might exacerbate the liver injury. We examined effects of feeding diets with either 15% (wt/wt) corn oil or 14% beef tallow and 1% corn oil for six weeks with either 6 or 20 g/100 g protein on acute hepatotoxicity. After the feeding period, liver injury was induced by injecting either with 600 mg/kg body weight acetaminophen suspended in gum arabic-based vehicle, or with vehicle alone during fasting status. Samples of liver and plasma were taken for analyses of hepatic glutathione (GSH) levels and liver-specific enzymes [(Glutamate-pyruvate transaminase (GPT) and glutamate-oxaloacetate transaminase (GOT)], respectively. Whereas GSH level was significantly lower in only group fed 15% corn oil with 6 g/100 g protein among acetaminophen-treated groups, activities of GPT and GOT were significantly elevated in all groups except the one fed beef tallow with 20 g/100 g protein, suggesting low protein might exacerbate drug-induced hepatotoxicity. The feeding regimens changed the ratio of 18:2n-6 to oleic acid (18:1n-9) in total liver lipids approximately five-fold, and produced modest changes in arachidonic acid (20:4n-6). We conclude that diets with high 18:2n-6 promote acetaminophen-induced liver injury compared to diets with more saturated fatty acids (SFA). In addition, protein restriction appeared to exacerbate the liver injury.
Unsaturated fats such as corn oil, soybean oil, and fish oil influence health and several disease processes. The average diet in United States contains 33% of total calories as fat (Ervin et al., 2004). In addition to a lowering of saturated fat intake an increase in unsaturated fats, such as linoleic acid (18:2n-6), generally has been recommended due to the ability of polyunsaturated fatty acids (PUFA) to reduce serum cholesterol, a risk factor for heart disease. However, PUFA are the most peroxidizable fatty acids and may contribute to diseases related to inflammation in which eicosanoids are released from tissues and contribute to pathologies.
Alcoholic liver disease is thought to be related to reduced antioxidant defenses and increased formation of free radicals. Dietary saturated fatty acids (SFA) such as those present in beef and pork are protective against alcohol-induced liver disease in man and animals (Nanji et al., 1989 & 1994a; Ronis et al., 2004; Roskams et al., 2003), whereas PUFA from corn oil and fish oil augment hepatic fibrosis and necrosis in rats fed a liquid diet containing alcohol (Frederiks et al., 1984). It has been suggested that the type of dietary fats can influence the extent of the formation of lipid radicals and hence lipid peroxidation products in response to oxidative injury because any change in dietary fats is reflected in membrane composition (Ronis et al., 2004). For example, PUFA are more prone to oxidation than SFA and monounsaturated fatty acids (MUFA) (Alexander-North et al., 1994) and increase susceptibility to oxidative injury by affecting antioxidant defense systems (Slim et al., 1996).
Hepatotoxicity caused by acetaminophen is due to the formation of a toxic metabolite by the cytochrome P-450 system, which is known to be susceptible to dietary manipulation (Baumgardner et al., 2008; Gonzalez, 2005; Porubsky et al., 2008). Some evidence (Nanji et al., 1995; Speck & Lauterburg, 1991) have shown that increased cytochrome P-450 levels, CYP 2E1, and microsomal mixed function oxidase (MFO) activity in animals fed PUFA compared to SFA might account, in part, for the liver injury. Specifically, linoleic acid as well as the highly unsaturated, long chain fatty acids found in the fish oils (EPA and DHA) have been shown to be important in this respect (Speck & Lauterburg, 1991). Also, acetaminophen administration as well as high PUFA intake increase the activity of hepatic enzymes such as glutamate-pyruvate transaminase (GPT) and glutamate-oxaloacetate transaminase (GOT) and cause cell damage that increases the excretion of these enzymes into blood (Jaya et al., 1993).
Protein deficiency causes impairment of antioxidant defenses, thereby increasing vulnerability to oxidative stress and sensitizing target tissues to necrosis (Huang & Fwu, 1992). Dietary protein restriction also affects hepatic lipid metabolism, lipoprotein formation, transport and secretion. It is well known that protein insufficiency contributes to liver steatosis and kwashiorkor is associated with fatty liver syndrome. Diets with 6% protein would not be expected to support optimal antioxidant defenses, glutathione synthesis or transmethylation. For example, rats fed low protein diets fail to maintain hepatic glutathione levels and have lower levels of antioxidant enzymes (Huang & Fwu, 1992). Therefore, it would be expected that protein malnutrition would interact with dietary fat sources to produce a very pro-inflammatory condition in liver, making it less able to defend against many hepatotoxins.
Since relationships between types and amounts of dietary fats and protein in acetaminophen-induced hepatotoxicity are not fully understood, we chose a rat model of hepatotoxicity to test the hypothesis that diets with SFA (beef tallow) prevent liver injury after acetaminophen challenge when compared to diets with PUFA (corn oil-rich diet) by changing the fatty acid composition of liver lipids. We also tested whether protein restriction might exacerbate the liver injury.
Forty-eight male Sprague-Dawley rats (Charles River, NC, USA) weighing 150 g to 190 g were housed individually in hanging wire-mesh cages with feeding cups. The room temperature (21 ± 1℃), lighting (on at 06:00, off at 18:00), and humidity (40-50%) were controlled. Rats had free access to restricted experimental diets (15 g per day) and tap water. Animals were randomly assigned to four diet groups and fed the modified AIN 93G diet (Reeves et al., 1993) for 6 weeks. The experimental protocol is shown in Table 1. One percent corn oil was included to prevent essential fatty acid deficiency and all diets contained 0.014 g/kg tert-butylhydroquinone to prevent peroxidization of lipids. All diets also contained: sucrose (Sigma Chemical Company, USA). 100 g/kg, celufil (Solka-Floc; International Fiber Co., USA), 50 g/kg; mineral mix (AIN-93G-MX, Dyets Inc. USA), 35 g/kg; vitamin mix (AIN-93-VX, Dyets), 10 g/kg; choline bitartrate (Sigma), 2.5 g/kg; L-cysteine (Sigma), 3 g/kg; and tert-butylhydroquinone (Sigma), 0.014 g/kg. The low protein diets were identical except that the protein level was reduced to 60 g/kg and diets were made isocaloric by increasing the cornstarch by 140 g/kg. The semipurified control diet was fed for one week to acclimate the animals to feed cups and individual caging, and then each group (n=8) was fed the indicated diets for the next six weeks to enable fatty acid composition to equilibrate with the diet pattern.
Each dietary group was subdivided into case and control groups. Each case group was unfed for 20 h prior to acetaminophen injection, and for 2 h afterwards, and was given food again before killing (Lim et al., 1994). Acetaminophen was given intraperitoneally in a vehicle of gum arabic (2% in saline) (Sigma) at a dose of 600 mg/kg body weight. After 18 h, animals were anesthetized with ketamine : acepromazine : xylazine (3:2:1, Sigma) at a dose of 0.1 mL/100 g body weight and blood samples were collected by cardiac puncture in tubes containing 3.2% sodium citrate (Sigma). Tubes were centrifuged at 2,000 rpm for 20 minutes to obtain plasma for liver function tests. The animals then were perfused transcardially with 0.9% NaCl and livers were snap-frozen. All frozen samples were stored at -80℃ for later experiments.
Glutamate-pyruvate transaminase (GPT) and glutamate-oxaloacetate transaminase (GOT) were measured with the Sigma transaminase [ALT/GPT and AST/GOT] Diagnostic kit. According to manufacturer's instruction, a standard curve was prepared using the Calibration Standard Solution with six levels of pyruvic acid. In brief, 0.2 mL plasma was added to 1.0 mL Sigma Prepared Substrate for GOT or 1.0 mL Alanine-α-KG Substrate for GPT in a 37℃ water bath for 1 h and for 30 min, respectively. Then 1.0 mL Sigma Color Reagent was added and incubated at room temperature (18-26℃) for 20 min, followed by adding 10.0 mL 0.40 N sodium hydroxide solution with vigorous mixing. Absorbance at 505 nm was recorded and the enzyme activities were determined in Sigma-Frankel (SF) Units/mL from the calibration curves.
Reduced glutathione (GSH) was measured by the modified method of Sedlak and Lindsay (1968). A standard curve was prepared using reduced GSH (Sigma) in 0.02 M EDTA (Biorad, USA). The curve was linear between 3.0 and 100.0 nmols GSH and data were expressed as µmole GSH per g tissue. Briefly, 600 µL aliquots of liver homogenate were treated with 60 µL 55% TCA (trichloroacetic acid, Sigma), shaken well and allowed to stand on ice for 10 min, followed by centrifugation at 4,000 g for 12 min. Four hundred µL of the supernatant was mixed with 1.0 mL of 1.2 M TRIS (Biorad) in 0.06 M EDTA (pH 8.9) and 100 µL of Ellman's reagent (DTNB, Sigma). The samples were allowed to develop at room temperature for 15 minutes and absorbance at 412 nm was recorded.
Extraction of fatty acids from liver homogenates was performed using the modified method of Folch et al. (1957). Extracted fatty acids were refluxed with 95% methanol and 5% sulfuric acid to form methyl esters of fatty acids. Fatty acid analysis was performed by gas-liquid chromatography using an Omegawax 320 column by the method described in Supelco GC bulletin 855 (Official methods of analysis, 1984). Fatty acid values are presented as µmole %, which represents the area under the chromatograph identified as a particular fatty acid based on its retention time when compared to the external standard mixture.
Fatty acid analysis was performed with a Hewlett-Packard 5890 gas chromatograph using a flexible fused capillary column (30 m × 0.25 mm I.D.: thickness 0.25 µm, J & W Scientific, Folsom, CA). The column temperature was programmed to begin at 205℃ for 20 min. and then increase at a rate of 3℃/min to a final temperature of 220℃, which held for 3 min. The injection port temperature was 250℃ and the detector was 260℃. Fatty acid values were presented as µmole %, which represents the area under the chromatograph identified as a particular fatty acid based on its retention time when compared to the internal standard.
All data were presented as mean ± SD. The data were analyzed with nonparametric method due to small sample sizes using SAS computer-based statistics programs. Kruskal-Wallis tests and the Wilcoxon rank sum tests were performed for one-way analysis of variance and statistical differences between two groups, respectively. Differences with p<0.05 were considered statistically significant.
Final body weights of animals were not significantly different. Liver weights per 100 g body weight were not significantly different between different groups (Table 3).
Plasma GOT and GPT levels increased significantly after acute intoxication with acetaminophen. Blood samples were collected after 18 h of exposure. Animals fed corn oil and/or low protein levels showed significant increases in both GOT and GPT levels (Fig. 1). In contrast, animals of only group 4 fed beef tallow with 20 g/100 g protein showed no significant differences after acetaminophen challenge (APAP groups in Fig. 1). Regardless of types of dietary fats, low protein aggravated acetaminophen-induced GOT and GPT levels. Therefore, beef tallow with 20 g/100 g protein protected against liver injury as judged by the increase in plasma activity of liver-specific marker enzymes after acetaminophen-induced intoxication.
Within same diet groups, liver GSH concentrations of acetaminophen-injected groups were significantly lower by 45% than those in vehicle group of corn oil and low protein-fed group (Fig. 2). Compared with gum arabic-treated groups, amounts and types of dietary fats and protein did not show any significant difference among groups. However, only GSH levels of group 1 (15% CO and 6% protein) were significantly lower than those of the other acetaminophen-treated groups (Fig. 2), suggesting low protein might exacerbate drug-induced hepatotoxicity.
Because the type and amount of dietary fat are reflected in tissue fatty acid profiles, fatty acid compositions were determined for liver lipids (Table 4). Corn oil-fed rats showed much higher 18:2n-6 and much lower 18:1n-9 compared with beef tallow-fed animals. Beef tallow-fed rats showed approximately 1.3- to 2-fold higher levels of 18:1n-9 and 2- to 3-fold lower levels of 18:2n-6 compared with corn oil-fed animals.
Interestingly, even in groups fed the same amount of beef tallow, PUFA levels (18:2n-6 and 20:4n-6) were significantly elevated in 6% protein (group 3) than in 20% protein (group 4), whereas SFA (except for 18:0) and MUFA showed the opposite patterns in groups 3 and 4. Thus, the feeding regimens changed the ratio of 18:2n-6 to oleic acid in total liver lipids approximately five-fold and produced modest changes in 20:4n-6.
This present study hypothesized that diets with SFA (beef tallow) prevent liver injury after acetaminophen challenge when compared to diets with PUFA by changing the fatty acid composition of liver lipids profile. We also tested whether these effects are dependant on the quantity of dietary protein. The results indicates that acetaminophen hepatotoxicity is significantly increased in rats fed diets containing high PUFA compared to rats fed low PUFA. This protective effect was not observed in rats fed a low protein diet and manifested in the concentrations of GPT and GOT in plasma and GSH levels in liver. Animals fed PUFA and/or low protein diet had significantly elevated plasma GPT and GOT levels and decreased GSH levels after acetaminophen challenge. Although the mechanism of these effects is unclear, these effects may be related to the observed changes in tissue fatty acid composition. The feeding of corn oil changed the ratio of linoleic to oleic acids in total liver lipids approximately five-fold higher than beef tallow diet. These observations are in agreement with Nanji et al. (1989, 1994a & 1994b), who reported that linoleic acid content of dietary fats was related to ethanol-induced liver injury and this injury was reversed with a diet rich in SFA (palm oil) but not unsaturated fats (fish oil). These effects were highly correlated with the changes in fatty acids composition of liver lipids.
Fatty acid composition of cellular membranes and in particular the MUFA and PUFA content are highly dependent on the fat composition of the diet by de novo synthesis or by elongation from dietary precursors (Camara et al., 1996). This was particularly significant with 18:1n-9 and 18:2n-6 in our data. In liver from rats fed corn oil, levels of 18:2n-6 were two- to three-fold those found in liver homogenates from beef tallow fed rats and 18:1n-9 showed opposite trends. These differences in fatty acid composition may cause alterations in enzyme activity and subsequent differences in drug metabolism and toxicity by altering membrane conformation and fluidity (Kuralay et al., 1998; McDanell et al., 1992; Nanji et al., 1994a). Moussa et al. (2000) suggests that lymphocyte phospholipid preferentially stimulated the synthesis of MUFA, especially 18:1n-9, by increasing Δ9-desaturase activity rather than SFA uptake in SFA-rich coconut-oil diets. Some evidence have supported that suppressive synthesis of oleic acid by n-6 PUFA rich diet (Momchilova et al., 1985) and inhibitory activity of Δ9-desaturase by an excess of linolenyl-CoA, which is metabolized from linoleic acid (Buller et al., 1986).
In contrast, the changes in fatty acid composition in rats fed SFA diet, which is low in 18:2n-6 may be related to the hepatoprotective effect of this diet. French et al. (1997) supported this hypothesis by showing that a diet low in 18:2n-6 prevented the fatty acid changes (e.g., reduction in 20:4n-6) seen with ethanol feeding and protected against mitochondrial fragility caused by ethanol ingestion (McDanell et al., 1992; Ronis et al., 2004). Several workers have suggested, in part, possible mechanisms that dietary 18:2n-6 levels might be a major factor in influencing oxidative stress. Slim et al. (1996) have suggested that 18:2n-6 might easily oxidize and generate cytotoxic products, such as linoleic acid hydroperoxides (Hennig et al., 1987) or 4-hydroxy-2-(E)-nonenal (Tamura & Shibamoto, 1991) by showing that feeding corn oil caused significantly elevated 18:2n-6 in liver and a marked increase in lipid peroxidation products of rabbit livers when compared to beef tallow which had the lowest 18:2n-6 in liver. In addition, elevated oleic acid may exert antioxidant effects on lipid peroxidation, acting as a competitive inhibitor of oxidation of PUFA (Lee et al., 1998). Although oleic acid can undergo oxidation with one double bond, they can not amplify propagative reactions due to their lack of multiple double bonds and thus their rate of oxidation is much less than that of PUFA. PUFA are excellent targets for lipid peroxidation because the formation of free radical is propagated to amplify the reaction.
The effects of changing the level of 20:4n-6 are controversial. Reduction of 20:4n-6 may decrease the formation of eicosanoids and promote less free radical generation (French et al., 1997; Nanji et al., 1989). Nanji et al. (1995) also suggested that this hepatoprotective effect might be caused by diminishing the availability of substrate for lipid peroxidation in the liver with substitution of PUFA with SFA. Therefore, decreased levels of this peroxidizable fatty acid may account, in part, for the absence of liver injury. Moreover, 20:4n-6 might contribute to increased oxidation in animals fed corn oil rich in 18:2n-6 (Slim et al., 1996). They also suggested that corn oil-fed animals have low scavenging capacity to quench free radicals and the low resistance to peroxidation when compared to animals fed more SFA diets. Nanji et al. (1994b & 1997) showed that both prostaglandin E2 (PGE2) and 6-keto-prostaglandin F1α were reduced in liver nonparenchymal cell, which were related to pathological liver injury in rats fed corn oil and ethanol. Acetaminophen-induced liver injury in vivo was significantly reduced by prostacyclin which is considered to be cytoprotective (Guarner et al., 1988; Ito et al., 2006; Nanji et al., 1994b & 1997).
GSH depletion can markedly compromise the overall antioxidant system because glutathione metabolism appears to be a crucial component of the overall defense against oxidative stress within a cell (Hennig & Toborek, 1994; Kuralay et al., 1998; Németh et al., 2004). Our results showed that hepatic GSH, which was measured after intoxication with acetaminophen, was significantly lower in corn oil and low protein fed animals than the counterpart of that group (Fig. 2), suggesting that low protein might exacerbate GSH depletion due to lack of substrates for GSH synthesis.
Protein deficiency also causes decreased GSH and additional depletion of antioxidant defenses, thereby increasing susceptibility to oxidative stress. Deneke et al. (1985) reported a significant reduction in superoxide dismutase (SOD) activity in lungs from rats fed a 3% protein (casein) diet and decreased catalase activity and GSH concentrations in lungs from rats fed a protein free diet. Huang and Fwu (1992) reported that gradually increasing the soybean oil level in the low protein diet (8% lactalbumin) resulted in further augmentation of thiobarbituric acid reactive substances (TBARS) concentrations in all tissues. Compared with rats fed a normal protein diet, the reduction in the activities of SOD and GSH peroxidase in liver in response to the increase of dietary soybean oil was more severe when these animals were fed a low protein diet. These data suggested that rats fed a low protein diet might be more susceptible to peroxidative tissue damage, in part, due to lowered antioxidative enzyme activities under the challenge of oxidative stress such as a high soybean oil intake.
Interestingly, our data showed that PUFA levels (18:2n-6 and 20:4n-6) were significantly elevated in the group fed beef tallow with 6% protein (group 3) than in the group fed the same amount of beef tallow with 20% protein (group 4). However, SFA (except for 18:0) and MUFA showed the opposite patterns in groups 3 and 4. This result implies that protein restriction affects hepatic lipid metabolism and would interact with dietary fats to produce a pro-inflammatory condition in liver by unclear mechanisms, making it less able to defend against many hepatotoxins.
Another possible explanation for hepatoprotective effect of beef tallow is due to changes in triglyceride levels. Nakamura et al. (1994) suggested that a decrease in hepatic phospholipid 20:4n-6 would allow enhanced triglyceride synthesis, because 20:4n-6 reduces hepatic triglyceride synthesis. The increase in 20:4n-6 in beef tallow fed groups may be one possible mechanism for reducing fat accumulation and less inducing fatty liver in these groups. Nanji et al. (1993) supported this evidence by showing that 20:4n-6 could not be synthesized to act as a target for free radical attack because beef tallow had very low 18:2n-6.
In conclusion, data from the present study confirm that high PUFA and/or low protein diet can lead to increased in vivo susceptibility to hepatotoxicity, compared to rich SFA and protein diet. Furthermore, our data suggested that this high SFA and protein diet had marked protection against the hepatotoxicity of acetaminophen by changing the fatty acid composition of liver lipids and protein insufficiency aggravated the enhanced liver injury resulting from feeding diets high in PUFA. Other types of dietary fats such as soybean and olive oils and/or chronic drug-induced hepatotoxicity need to be investigated for future study.
Figures and Tables
Fig. 1
The effect of acetaminophen on plasma levels of the liver enzymes, GPT (panel A) and GOT (panel B). Abbreviations: Group 1, 15% corn oil + 6% protein; Group 2, 15% corn oil + 20% protein; Group 3, 14% beef tallow + 1% corn oil + 6% protein; Group 4, 14% beef tallow + 1% corn oil + 20% protein; GA, gum arabic vehicle injected to control group; APAP, acetaminophen injected to case group. Each bar represents the mean ± SE (n=8). Different letters (a, b & c) indicate significant difference among APAP groups (*p<0.05, **p<0.01).
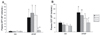
Fig. 2
The effect of acetaminophen on GSH levels. Abbreviations: Group 1, 15% corn oil + 6% protein; Group 2, 15% corn oil + 20% protein; Group 3, 14% beef tallow + 1% corn oil + 6% protein; Group 4, 14% beef tallow + 1% corn oil + 20% protein; GA, gum arabic vehicle injected to control group; APAP, acetaminophen injected to case group. Each bar represents the mean ± SE (n=8). **p<0.01 compared with GA and APAP groups in the same group. Different letters (a, b & c) indicate significant difference among APAP groups (*p<0.05).
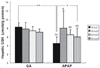
Acknowledgment
I give special thanks to Kathie L. Wickwire for technical support and Dr. James L. Hargrove for careful guidance.
References
1. Alexander-North LS, North JA, Kiminyo KP, Buettner GR, Spector AA. Polyunsaturated fatty acids increase lipid radical formation induced by oxidant stress in endothelial cells. J Lipid Res. 1994. 35:1773–1785.


2. AOAC. Official Methods of Analysis. 1984. 14th Edition. Maryland. USA: Association of Official Analytical Chemists.
3. Baumgardner JN, Shankar K, Hennings L, Badger TM, Ronis MJ. A new model for nonalcoholic steatohepatitis in the rat utilizing total enteral nutrition to overfeed a high-polyunsaturated fat diet. Am J Physiol Gastrointest Liver Physiol. 2008. 294:G27–G38.


4. Buller KL, Enser M. The effects of food intake and dietary fatty acids on the activity of stearoyl-CoA Δ9-desaturase in pig adipose tissue. J Agri Sci. 1986. 106:601–609.


5. Camara M, Mourot J, Février C. Influence of two dietary fats on lipid synthesis in the pig: Comparative study of liver, muscle and two back fat layers. Ann Nutr Metab. 1996. 40:287–295.


6. Deneke SM, Lynch BA, Fanburg BL. Effects of low protein diets or feed restriction on rat lung glutathione and oxygen toxicity. J Nutr. 1985. 115:726–732.


7. Ervin RB, Wright JD, Wang C, Kennedy-Stephenson J. Dietary intake of fats and fatty acids for the United states population:1999-2000. Adv Data. 2004. 348:1–6.
8. Folch J, Lees M, Sloane-Stanley GH. Extraction of fatty acid. J Biol Chem. 1957. 226:497–509.
9. Frederiks WM, Fronik GM, Hesseling JMG. A method for quantitative analysis of the extent of necrosis ischemic rat liver. Exp Mol Pathol. 1984. 41:119–125.


10. French SM, Morimoto M, Reitz RC, Koop D, Klopfenstein B, Estes K, Clot P, Ingelman-Sundberg M, Albano E. Lipid peroxidation, CYP 2E1 and arachidonic acid metabolism in alcoholic liver disease in rats. J Nutr. 1997. 127:907S–911S.
11. Gonzalez FJ. Role of cytochromes P450 in chemical toxicity and oxidative stress: studies with CYP2E1. Mutat Res. 2005. 569:101–110.


12. Guarner F, Boughton-Smith NK, Blackwell GJ, Moncada S. Reduction by prostacyclin of acetaminophen-induced liver toxicity in the mouse. Hepatology. 1988. 8:248–253.


13. Hennig B, Toborek M. Fatty acid-mediated effects on the glutathione redox cycle in cultured endothelial cells. Am J Clin Nutr. 1994. 59:60–65.


14. Hennig B, Enoch C, Chow CK. Protection by vitamin E against endothelial cell injury by linoleic acid hydroperoxides. Nutr Res. 1987. 7:1253–1259.


15. Huang CJ, Fwu ML. Protein insufficiency aggravates the enhanced lipid peroxidation and reduced activities of antioxidant enzymes in rats fed diets high in polyunsaturated fat. J Nutr. 1992. 122:1182–1189.


16. Ito Y, Abril ER, Bethea NW, McCuskey MK, McCuskey RS. Dietary steatotic liver attenuates acetaminophen hepatotoxicity in mice. Microcirculation. 2006. 13:19–27.


17. Jaya DS, Augstine J, Menon VP. Role of lipid peroxides, glutathione and antiperoxidative enzymes in alcohol and drug toxicity. Ind J Exp Bio. 1993. 31:453–459.
18. Kuralay F, Akarca US, Özütemiz AÖ, Kutay F, Batur Y. Possible role of glutathione in prevention of acetaminophen-induced hepatotoxicity enhanced by fish oil in male Wistar rats. J Toxicol Environ Health A. 1998. 53:223–229.


19. Lee C, Barnett J, Reaven PD. Liposomes enriched in oleic acid are less susceptible to oxidation and have less proinflammatory activity when exposed to oxidizing conditions. J Lipid Res. 1998. 39:1239–1247.


20. Lim SP, Andrew FJ, O'Brien PE. Misoprostol protection against acetaminophen-induced hepatotoxicity in the rat. Dig Dis Sci. 1994. 39:1249–1256.


21. McDanell RE, Beales D, Henderson L, Sethi JK. Effect of dietary fat on the in vitro hepatotoxicity of paracetamol. Biochem Pharmacol. 1992. 44:1303–1306.


22. Momchilova A, Petkova D, Mechev I, Dimotrov G, Koumanov K. Sensitivity of 5'-nucleotidase and phospholipase A2 towards liver plasma membrane modifications. Int J Biochem. 1985. 17:787–792.


23. Moussa M, Tkaczuk J, Ragab J, Garcia J, Abbal M, Ohayon E, Ghisolfi J, Thouvenot J. Relationship between the fatty acid composition of rat lymphocytes and immune functions. Br J Nutr. 2000. 83:327–333.


24. Nakamura MT, Tang AB, Villanueva J, Halsted CH, Phinney SD. Selective reduction of Δ6 and Δ5 desaturase activities but not Δ9 desaturase in micropigs chronically fed ethanol. J Clin Invest. 1994. 93:450–454.


25. Nanji AA, Hossein-Sadrzadeh SM, Dannenberg AJ. Liver microsomal fatty acid composition in ethanol-fed rats: effect of different dietary fats and relationship to liver injury. J Nutr. 1994a. 18:1024–1028.


26. Nanji AA, Hossein-Sadrzadeh SM, Yang EK, Fogt F, Meydani M, Dannenberg AJ. Dietary saturated fatty acids: a novel treatment for alcoholic liver disease. Gastroenterology. 1995. 109:547–554.


27. Nanji AA, Khwaja S, Sadrzadeh SMH. Decreased prostacyclin production by liver non-parenchymal cells precedes liver injury in experimental alcoholic liver disease. Life Sci. 1994b. 54:455–461.


28. Nanji AA, Mendenhall CL, French SW. Beef fat prevents alcoholic liver disease in the rat. Alcohol Clin Exp Res. 1989. 13:15–19.


29. Nanji AA, Miao L, Thomas P, Rahemtulla A, Khwaja S, Zhao S, Peter D, Tahan SR, Dannenberg AJ. Enhanced cyclooxygenase-2 gene expression in alcoholic liver disease in the rat. Gastroenterology. 1997. 112:943–951.


30. Nanji AA, Zhao S, Lamb RG, Sadrzadeh SMH, Dannenberg AJ, Waxman DJ. Changes in microsomal phospholipases and arachidonic acid in experimental alcoholic liver injury: Relationship to cytochrome P-450 2E1 induction and conjugated diene formation. Alcohol Clin Exp Res. 1993. 17:598–603.


31. Németh K, Mézes M, Gaál T, Bartos A, Balogh K, Husvéth F. Effect of supplementation with methionine and different fat sources on the glutathione redox system of growing chickens. Acta Vet Hung. 2004. 52:369–378.


32. Porubsky PR, Meneely KM, Scott EE. Structures of human cytochrome P-450 2E1. Insights into the binding of inhibitors and both small molecular weight and fatty acid substrates. J Biol Chem. 2008. 283:33698–33707.
33. Reeves PG, Nielsen F, Fahey G. AIN-93 purified diets for laboratory rodents: Final report of the American Institute of Nutrition Ad Hoc writing committee on the reformulation of the AIN-76A Rodent diet. J Nutr. 1993. 123:1939–1951.


34. Ronis MJ, Korourian S, Zipperman M, Hakkak R, Badger TM. Dietary saturated fat reduces alcoholic hepatotoxicity in rats by altering fatty acid metabolism and membrane composition. J Nutr. 2004. 134:904–912.


35. Roskams T, Yang SQ, Koteish A, Durnez A, DeVos R, Huang X, Achten R, Verslype C, Diehl AM. Oxidative stress and oval cell accumulation in mice and humans with alcoholic and nonalcoholic fatty liver disease. Am J Pathol. 2003. 163:1301–1311.


36. Sedlak J, Lindsay R. Estimation of total, protein-bounded and nonprotein sulfhydryl groups in tissues with Ellman's Reagent. Anal Biochem. 1968. 25:192–205.


37. Slim RM, Toborek M, Watkins BA, Boissonneault GA, Hennig B. Susceptibility to hepatic oxidative stress in rabbits fed different animal and plant fats. J Am Coll Nutr. 1996. 15:289–294.

