Abstract
Aloe products are one of the top selling health-functional foods in Korea, however the adequate level of intake to achieve desirable effects are not well understood. The objective of this study was to determine the intestinal uptake and metabolism of physiologically active aloe components using in vitro intestinal absorption model. The Caco-2 cell monolayer and the everted gut sac were incubated with 5-50 µM of aloin, aloe-emodin, and aloesin. The basolateral appearance of test compounds and their glucuronosyl or sulfated forms were quantified using HPLC. The % absorption of aloin, aloe-emodin, and aloesin was ranged from 5.51% to 6.60%, 6.60% to 11.32%, and 7.61% to 13.64%, respectively. Up to 18.15%, 18.18%, and 38.86% of aloin, aloe-emodin, and aloesin, respectively, was absorbed as glucuronidated or sulfated form. These results suggest that a significant amount is transformed during absorption. The absorption rate of test compounds except aloesin was similar in two models; more aloesin was absorbed in the everted gut sac than in the Caco-2 monolayer. These results provide information to establish adequate intake level of aloe supplements to maintain effective plasma level.
Aloe is widely used in food products, pharmaceuticals, and cosmetics due to its aromatic and therapeutic properties (Davis et al., 1994; Korkina et al., 2003). The aloe product ranked first in health food sales of 2005 in Korea (Park et al., 2005). Aloe leaves consist of gel and latex. Most of the compounds in leaf exudate identified so far are chromones, anthraquinones and anthrones. Aloin is the major anthraquinone of aloe and characterized as the C-glycoside of aloe-emodin (Fig. 1) (Saccù et al., 2001). Aloesin is a chromone derivative isolated from aloe, which is regarded as the parent compound of the aloe chromones (McCarthy & Haynes, 1967).
Due to their polyphenolic structure, aloin, aloe-emodin and aloesin are considered as physiologically active components responsible for reported biological effects such as reduction of inflammation (Speranza et al., 2005), acceleration of wound healing (Maenthaisong et al., 2007; Moore & Cowman, 2008; Ni et al., 2007), and protective effect against liver injury (Chandan et al., 2007).
However, the ingestion of aloe is known to be associated with diarrhea (Ishii et al., 1994) and possible cell cytotoxicity (Esmat et al., 2006). Although the mechanisms that control the balance of these various effects are poorly known, the information on bioavailability of aloe components will provide new insight into a proper amount of oral intake to exert expected in vivo physiological activity. Therefore, we measured intestinal % absorption and transformation of major aloe components using two types of in vitro intestinal absorption model.
Aloin and aloe-emodin were purchased from the Sigma-Aldrich Co. (St. Louis, MO, USA) and aloesin was supplied by Univera Inc. (Seoul, Korea). Dulbecco's modified Eagle medium (DMEM), fetal bovine serum (FBS), sodium pyruvate, L-glutamine, antibiotics-antimycotics solution, and trypsin-EDTA were purchased from GIBCO Co. (Grand Island, NY, USA). HPLC grade methanol and water were from Merck (Darmstadt, Germany). Other chemicals otherwise indicated were from Sigma-Aldrich Co. (St. Louis, MO, USA).
Caco-2 cells were obtained from American Type Culture Collection (ATCC, Rockville, MD, USA). The cells were cultured in DMEM media with high glucose and L-glutamine, supplemented with 10% FBS, 100 U/ml penicillin, 100 µg/ml streptomycin, and 1% nonessential amino acids. The atmosphere was kept with 90-95% relative humidity, 37℃, 5% CO2, and 95% air.
For the uptake studies, Caco-2 cells were seeded on Transwell insert (12-well, Corning Costar Corp., Cambridge, MA, USA) at density of 5×104 cells/cm2. The cells were used between passages number 26-48. The cell medium was replaced every other day for 18-24 days until analysis. The integrity of the cell monolayer was confirmed by the transepithelial electric resistance (TEER) values measured using a Millicell-ERS voltohmmeter (Millipore Corp., USA). The monolayer with TEER of more than 550 Ω·cm2 was used for the uptake experiments.
To measure the apical-to-basolateral uptake, 1.5 ml of HBSS (pH 7.4, 37℃) was added to the basolateral chamber of the Transwell insert and then 0.5 ml of the test solution (pH 6.0, 37℃) containing aloe components (aloin, aloe-emodin, or aloesin) was added to the apical side. Aloin, aloe-emodin, or aloesin was dissolved in dimethyl sulfoxide (DMSO) and diluted with HBSS prior to starting the experiment. The final concentrations of each compound were 5, 10, and 50 µM. These test solutions were added on the apical side of Caco-2 monolayers and incubated for 1 hr at 37℃. After the incubation, the apical and the basolateral solutions were collected and stored at -70℃ until assayed. The % absorption of aloe components was calculated as (basolateral side concentration of aloe components after incubation × 100)/(apical side treatment concentration of aloe components).
After an overnight fast, the rats were sacrificed by a qualified person, and the entire small intestine was quickly excised and flushed through several times with NaCl solution (0.9%, w/v) at room temperature and immediately placed in warm (37℃), oxygenated TC 199 medium. The intestine was gently everted over a glass rod (2.5 mm diameter), and one end was clamped and the whole length of the intestine was filled with fresh oxygenated medium and sealed with a second clamp and the resulting large gut sac was divided into sacs of around 2.5 cm in length using braided silk sutures. For each experiment, 12-15 sacs were prepared, starting from the end of the duodenum, to ensure that sacs were from the upper/mid-jejunum where transport activity is maximal. To study tissue uptake of aloin, aloe-emodin or aloesin, 10 µM test compounds were added to the chambers. The sacs were then placed in individual incubation chambers containing 6 ml of pre-gassed oxygenated media at 37℃. At one hour incubation, sacs were removed, washed three times in saline (0.9%, w/v) and blotted dry, cut open and the serosal fluid drained into small tubes. Each sac was weighed before and after serosal fluid collection to calculate the volume inside the sac. The protein content of the digest or homogenates was determined using the modified Lowry method (Keller & Neville, 1986) with bovine serum albumin as standard and the uptake into the serosal side was expressed as nmol/mg of tissue protein.
The apical and the basolatral solutions and the serosal and the mucosal fluids were each divided into two aliquots. Half of either apical or basolateral solution was mixed with 20 U of a sulfatase type H-5 solution in 100 mmol/L acetate buffer (0.1 mL, pH 5.0) and incubated at 37℃ for 45 min. Then, the same volume of methanol was added to the mixture and centrifuged at 10,000 × g for 10 min. The resulting supernatant solution was used as a sulfatase-treated sample. The other half was dissolved and used as an untreated sample. The amounts of the metabolites (glucuronides/sulfates) were calculated by the difference between the amounts of aloin/aloe-emodin/aloesin from sulfatase-treated samples and those from untreated samples. Because sulfatase type H-5 possesses sulfatase, glucuronidase, and glucosidase activities, other metabolized forms, such as methylated forms, were not identified in this study.
Aloin, aloe-emodin, and aloesin were identified by HPLC analysis using a C18 column (150 × 4.6 mm i.d.; 5 µm). The mobile phase at a flow rate of 1.0 ml/min was composed of acetonitrile/water (80:20, v/v) for aloin, and methanol/water (80:20, v/v) for aloesin. The eluate was monitored with a UV detector at 254 nm. For the analysis of aloe-emodin, HPLC was performed using a TSP system (Thermo Quest, Tokyo, Japan) equipped with two P4000 gradient pumps, a UV 6000 photodiode array detector (200-500 nm range; 5 nm bandwidth) and an LCQ ESI/MS detector controlled by Chromoquest software (version 2.51; 1998).
Aloin applied to the apical side of Caco-2 monolayer at a concentration range between 5-50 µM increased aloin and its glucuronated or sulfated forms at basolateral side (Table 1). Aloin concentration was 0.11, 0.42, and 1.99 nmol/cm2 culture area and its metabolized conjugates concentration was 0.05, 0.11, and 0.62 nmol/cm2 culture area when 5, 10, and 50 µM of aloin was applied, respectively. The results imply that a significant amount of aloin is converted by phase II enzyme present in the epithelial cells.
Aloe-emodin, the aloin aglycon, was applied to the apical side of Caco-2 monolayers at 5-50 µM, and not only aloe-emodin but its glucuronides/sulfates were detected in the basolateral side solution after 1 hour incubation (Table 2). Aloe-emodin concentration was 0.13, 0.86, and 2.51 nmol/cm2 culture area and its metabolized conjugates concentration was 0.06, 0.12, and 0.92 nmol/cm2 culture area when cells were treated with 5, 10, and 50 µM, respectively. The absorption rate of aloe-emodin was higher than that of aloin. There was a dose-dependent increase in absorption rate. The absorption rate of 50 µM aloe-emodin, however, was lower than that of 10 µM aloe-emodin, indicating that aloe-emodin may start to approach to physiological saturation levels at 50 µM treatment.
Aloesin, a chromone aglycon applied to the apical side of Caco-2 monolayers at 5-50 µM of concentration was appeared as aloesin and its glucuronides/sulfates forms in the basolateral side solution after 1 hour incubation (Table 3). Unlike aloin or aloe-emodin, the amount of glucuronides/sulfates forms was higher than that of aglycon, suggesting that phase II enzymes may play an important role in the aloesin absorption. The % absorption of aloesin was 7.61%, 13.64%, and 8.14% at 5, 10, and 50 µM, respectively, which were higher than those of either aloin or aloe-emodin (Table 4). Aloesin showed a similar absorption pattern with aloe-emodin.
To compare the Caco-2 monolayer with the everted gut sac as an in vitro model of intestinal absorption, everted gut sacs were incubated with aloin, aloe-emodin, and aloesin at 10 µM concentration. As shown in Table 5, both aloe components and their glucuronide/sulfate forms were also detected in the everted gut sac model. The levels of aloin and aloe-emodin were higher than their metabolized conjugates, whereas the level of aloesin was less than its metabolized conjugates in the sac (Table 5). The % absorption of both aloin and aloe-emodin was similar to the Caco-2 monolayer data, while more aloesin were shown to be absorbed in the gut sac compared to the Caco-2 monolayer (p<0.05) (Fig. 2).
In this study, we determined the absorption rate and absorption forms of aloin, aloe-emodin, and aloesin using the Caco-2 cell monolayer model and the everted gut sac model. Despite the frequent use of aloe and its products, limited information is available for their bioavailability which is a very common phenomenon for other phytochmicals as well.
Caco-2 cells are derived from the human colon carcinoma, however, they spontaneously differentiate into the absorptive intestine-like cells during culture, such as microvillous structure, carrier-mediated transport system, and brush-border enzyme (Hidalgo et al., 1989). These features of Caco-2 cell line are similar to those of the small intestine rather than the colon. Due to its similarity to human intestinal epithelium, Caco-2 cell culture model has been widely used to determine the absorption rate of chemicals in food or drug. The everted gut sac is also a useful in vitro model to study drug transport (Barthe et al., 1999) and provides information on absorption mechanisms. It mimics in vivo intestinal environment, however needs to be carefully prepared from rat small intestine for good morphology. The everted gut sac is metabolically active only for 2 h at 37℃.
In this study, the % absorption of aloin, aloe-emodin, and aloesin was ranged from 5.51% to 6.60%, 6.60% to 11.32%, and 7.61% to 13.64%, respectively, between 5 µM and 50 µM. The mechanisms and characteristics of intestinal anthraquinone absorption are not well understood compared to other polyphenols. Alves et al. (2004) reported that emodin showed a higher affinity for phospholipid membranes than aloin did. Affinity to the cellular membrane plays an important role in the efficiency of cellular uptake by passive diffusion. Azuma et al. (2002) showed that a combination of lipids and emulsifiers is necessary for enhancing quercetin absorption. Thus the lower concentration in basolateral solution probably resulted from the poor lipophilic properties of aloin. The initial step in the absorption process for polyphenols is deglycosylation. Previous studies (Lambert et al., 1999; Németh et al., 2003; Wilkinson et al., 2003) found specific intracellular and membrane-bound hydrolyzing enzyme activity in the small intestine is a critical determinant in polyphenol absorption process. This might explain the difference in cellular absorption efficiency between aloin and aloe-emodin.
An alternative absorptive mechanism involves transport of the polyphenol glycoside into the enterocyte as an intact form via the function of a sugar transporter such as sodium-dependent glucose transporter-1 (SGLT1) (Olthof et al., 2000). Sodium-dependent efflux of pre-loaded radio-labeled galactose by rat everted jejunum sacs provided evidence that the SGLT-1 interacts with flavonoid glycosides including quercetin-3-glucoside (Q3Glc) and quercetin-4'-glucoside (Q4'Glc) (Gee et al., 1998).
During absorption, polyphenols are metabolized in the Caco-2 cells by a phase II metabolic enzyme such as glucuronyl-, sulfate-, or glutathione-transferase, each of which may play an important role in drug accumulation and transport in human intestine (Vereczkey et al., 2005). Our results suggest that a significant proportion of aloin and aloe-emodin was converted to their glucuronide/sulfate conjugates during absorption. Mailleau et al. (1998) reported that SGLT1 activity was rapidly increased from day 12 up to day 20 post-seeding of Caco-2 cells. Studies also reported that monoglucosides of polyphenols can be transported across the apical membrane of enterocytes by the SGLT1 (Gee et al., 1998; Walgren et al., 2000). The absorption of aloin in its unchanged form in this study may indicate the involvement of the SGLT1, since aloin shares a basic monoglucoside structure. The lower % absorption of aloin compared to aloe-emodin may be explained by a limited number of receptors/transporters capable of handling the molecule. In a recent study, Teng et al. (2007) reported that the SGLT1 blocker can inhibit the cellular accumulation of emodin across the apical membrane of Caco-2 cells. This suggests that partial intestinal absorption of emodin may be transported by SGLT1. These results indicate that the absorption of aloe-emodin, crossing the apical membrane in Caco-2 cells, may be partially influenced by SGLT1 and be metabolized by phase II enzymes.
We observed that the absorption rate of aloe-emodin was significantly higher at 10 µM treatment compare to those of the 5 µM or 50 µM treatment, indicating that aloe-emodin may start to approach to physiological saturation level at 50 µM treatment.
Chromones form a class of compounds, the importance of which arises from their wide natural occurrence as subunits in flavonoids (Miao & Yang, 2000) and their biological activities (Hadjeri et al., 2003). However, reports on the metabolism or absorption properties of chromones are limited (Kanai et al., 1979; Maurizis et al., 1991; Muni et al., 1978). The present study indicated that aloesin possesses similar absorption pattern with aloe-emodin and also reaches physiological saturation levels at 50 µM. The results also indicated that chromones form conjugates in the human small intestine by UDP-glucuronosyltransferase (UGT) (Vaidyanathan & Walle, 2002). Liu et al. (2007) indicated that well-expressed UGT isoforms in the Caco-2 cells, UGT1A1, UGT1A3, UGT1A6, and UGT2B7, were capable of metabolizing flavonoids.
The absorption rate of aloesin were 7.61%, 13.64%, and 8.14% at 5, 10, and 50 µM, respectively, which were higher than those of either aloin or aloe-emodin, and the absorbed concentration was higher at 50 µM compared to either aloin or aloe-emodin. Free hydroxyl groups in flavonoids can hinder transport in Caco-2 cell monolayer (Tammela et al., 2004). Ollila et al. (2002) stated that polyhydroxylated chemicals show longer retention delays in membranes, and this is most likely due to hydrogen-bond formation between their hydroxyl groups and polar groups of the lipid molecules at the lipid/water interface. Aloin and aloe-emodin have two hydroxyl groups, while aloesin has one hydroxyl group in their structure. Therefore, hydroxyl group would be responsible for high absorption of aloesin.
The everted gut sac experiment was conducted to compare the Caco-2 monolayer data. The absorption rate of both aloin and aloe-emodin were similar rate Caco-2 monolayer model data, while aloesin absorption percentage was significantly higher in the everted gut sac model. One of the functional differences between normal intestine and Caco-2 cells is its lack of expression of the cytochrome P450 and LPH (Paine & Fisher, 2000). Moreover, Caco-2 cells do not always express appropriate amounts of transporters or enzymes, which may introduce bias. Therefore, the everted gut sac model may provide more useful in vitro bioavailability screening tool for many physiologically active herbal components.
This study provided information on the intestinal absorption rate and possible absorption mechanisms of aloe components. These results may be used to establish adequate intake level of aloe supplements to reach effective plasma concentration.
Figures and Tables
Fig. 2
Percent absorptions of aloe components in Caco-2 monolayer model and everted gut sac model. To measure the absorption in everted gut sac model, aloin, aloe-emodin, or aloesin solutions (10 µM) were incubated with everted gut sac for 1 hr at 37℃. After the incubation, the solutions in the sacs were assayed by HPLC. The amounts of the glucuronides/sulfates conjugates were calculated by the difference between the amounts of aloin, aloe-emodin, or aloesin from sulfatase-treated samples and those from untreated samples. Values are means ± S.D. Means with letters (a, b, c) within a column are significantly different from each other at p<0.05 as determined by Duncan's multiple range test. Means with # is significantly different from corresponding everted gut sac, #<0.01.
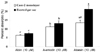
Table 1
Concnetration of aloin and its glucuronides/sulfates in basolateral side of Caco-2 monolayer model
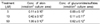
Table 2
Concnetration of aloe-emodin and its glucuronides/sulfates in basolateral side of Caco-2 monolayer model
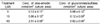
Table 3
Concnetration of aloesin and its glucuronides/sulfates in basolateral side of Caco-2 monolayer model
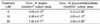
References
1. Alves DS, Pérez-Fons L, Estepa A, Micol V. Membrane-related effects underlying the biological activity of the anthraquinones emodin and barbaloin. Biochem Pharmacol. 2004. 68:549–561.


2. Azuma K, Ippoushi K, Ito H, Higashio H, Terao J. Combination of lipids and emulsifiers enhances the absorption of orally administered quercetin in rats. J Agric Food Chem. 2002. 50:1706–1712.


3. Barthe L, Woodley J, Houin G. Gastrointestinal absorption of drugs: methods and studies. Fundam Clin Pharmacol. 1999. 13:154–168.


4. Chandan BK, Saxena AK, Shukla S, Sharma N, Gupta DK, Suri KA, Suri J, Bhadauria M, Singh B. Hepatoprotective potential of Aloe barbadensis Mill. against carbon tetrachloride induced hepatotoxicity. J Ethnopharmacol. 2007. 111:560–566.


5. Davis RH, Donato JJ, Hartman GM, Haas RC. Anti-inflammatory and wound healing activity of a growth substance in Aloe vera. J Am Podiatr Med Assoc. 1994. 84:77–81.


6. Esmat AY, Tomasetto C, Rio MC. Cytotoxicity of a natural anthraquinone (Aloin) against human breast cancer cell lines with and without ErbB-2: topoisomerase IIalpha coamplification. Cancer Biol Ther. 2006. 5:97–103.


7. Gee JM, DuPont MS, Rhodes MJ, Johnson IT. Quercetin glucosides interact with the intestinal glucose transport pathway. Free Radic Biol Med. 1998. 25:19–25.


8. Hadjeri M, Barbier M, Ronot X, Mariotte AM, Boumendjel A, Boutonnat J. Modulation of P-glycoprotein-mediated multidrug resistance by flavonoid derivatives and analogues. J Med Chem. 2003. 46:2125–2131.


9. Hidalgo IJ, Raub TJ, Borchardt RT. Characterization of the human colon carcinoma cell line (Caco-2) as a model system for intestinal epithelial permeability. Gastroenterology. 1989. 96:736–749.


10. Ishii Y, Tanizawa H, Takino Y. Studies of aloe. V. Mechanism of cathartic effect. Biol Pharm Bull. 1994. 17:651–653.
11. Kanai Y, Nakai Y, Nakajima N, Tanayama S. Metabolic disposition of 6-ethyl-3-(1H-tetrazol-5-yl)-chromone, a new antiallergic agent, in the rat, guinea-pig, rabbit, dog and monkey. Xenobiotica. 1979. 9:33–50.


12. Keller RP, Neville MC. Determination of total protein in human milk: comparison of methods. Clin Chem. 1986. 32:120–123.


13. Korkina L, Suprun M, Petrova A, Mikhal'chik E, Luci A, De Luca C. The protective and healing effects of a natural antioxidant formulation based on ubiquinol and aloe vera against dextran sulfate-induced ulcerative colitis in rats. Biofactors. 2003. 18:255–264.


14. Lambert N, Kroon PA, Faulds CB, Plumb GW, McLauchlan WR, Day AJ, Williamson G. Purification of cytosolic beta-glucosidase from pig liver and its reactivity towards flavonoid glycosides. Biochim Biophys Acta. 1999. 1435:110–116.


15. Liu X, Tam VH, Hu M. Disposition of flavonoids via enteric recycling: determination of the UDP-glucuronosyltransferase isoforms responsible for the metabolism of flavonoids in intact Caco-2 TC7 cells using siRNA. Mol Pharm. 2007. 4:873–882.


16. Maenthaisong R, Chaiyakunapruk N, Niruntraporn S, Kongkaew C. The efficacy of aloe vera used for burn wound healing: a systematic review. Burns. 2007. 33:713–718.


17. Mailleau C, Capeau J, Brahimi-Horn MC. Interrelationship between the Na+/glucose cotransporter and CFTR in Caco-2 cells: relevance to cystic fibrosis. J Cell Physiol. 1998. 176:472–481.


18. Maurizis JC, Nicolas C, Verny M, Ollier M, Faurie M, Payard M, Veyre A. Biodistribution and metabolism in rats and mice of bucromarone. Drug Metab Dispos. 1991. 19:94–99.
19. McCarthy TJ, Haynes LJ. The distribution of alosein in some south African aloe species. Planta Med. 1967. 15:342–344.


20. Miao H, Yang Z. Regiospecific carbonylative annulation of iodophenol acetates and acetylenes to construct the flavones by a new catalyst of palladium-thiourea-dppp complex. Org Lett. 2000. 2:1765–1768.


21. Moore Z, Cowman S. A systematic review of wound cleansing for pressure ulcers. J Clin Nurs. 2008. 17:1963–1972.


22. Muni IA, Leeling JL, Helms RJ, Johnson N. Antiallergic chromones. I. Disposition of 5-(3-p-cyanophenoxy-2-hydroxy-1-propoxy)-2-(1H-tetrazol-5-yl) chromone in four mammalian species. Toxicol Appl Pharmacol. 1978. 43:527–534.


23. Németh K, Plumb GW, Berrin JG, Juge N, Jacob R, Naim HY, Williamson G, Swallow DM, Kroon PA. Deglycosylation by small intestinal epithelial cell beta-glucosidases is a critical step in the absorption and metabolism of dietary flavonoid glycosides in humans. Eur J Nutr. 2003. 42:29–42.
24. Ni Y, Turner D, Yates K, Tizard I. Stabilization of growth factors relevant to wound healing by a plant cell wall biomaterial. Planta Med. 2007. 73:1260–1266.


25. Ollila F, Halling K, Vuorela P, Vuorela H, Slotte JP. Characterization of flavonoid--biomembrane interactions. Arch Biochem Biophys. 2002. 399:103–108.


26. Olthof MR, Hollman PC, Vree TB, Katan MB. Bioavailabilities of quercetin-3-glucoside and quercetin-4'-glucoside do not differ in humans. J Nutr. 2000. 130:1200–1203.


27. Paine MF, Fisher MB. Immunochemical identification of UGT isoforms in human small bowel and in caco-2 cell monolayers. Biochem Biophys Res Commun. 2000. 14:1053–1057.


28. Park YG, Park MY, Sung MK, Kwon H. Study on the intake pattern of health intended foods depending on inclusion of proclaimed health functional food materials. Journal of the Korean Society of Food Science and Nutrition. 2005. 34:374–379.


29. Saccù D, Bogoni P, Procida G. Aloe exudate: characterization by reversed phase HPLC and headspace GC-MS. J Agric Food Chem. 2001. 49:4526–4530.


30. Speranza G, Morelli CF, Tubaro A, Altinier G, Durì L, Manitto P. Aloeresin I, an anti-inflammatory 5-methylchromone from cape aloe. Planta Med. 2005. 71:79–81.


31. Tammela P, Laitinen L, Galkin A, Wennberg T, Heczko R, Vuorela H, Slotte JP, Vuorela P. Permeability characteristics and membrane affinity of flavonoids and alkyl gallates in Caco-2 cells and in phospholipid vesicles. Arch Biochem Biophys. 2004. 425:193–199.


32. Teng ZH, Zhou SY, Ran YH, Liu XY, Yang RT, Yang X, Yuan CJ, Mei QB. Cellular absorption of anthraquinones emodin and chrysophanol in human intestinal Caco-2 cells. Biosci Biotechnol Biochem. 2007. 71:1636–1643.


33. Vaidyanathan JB, Walle T. Glucuronidation and sulfation of the tea flavonoid (-)-epicatechin by the human and rat enzymes. Drug Metab Dispos. 2002. 30:897–903.


34. Vereczkey L, Jemnitz K, Monostory K, Veres Z, Kóbori L. The role of drug metabolizing enzymes in the effect and side-effect of the drugs. Orv Hetil. 2005. 146:947–952.
35. Walgren RA, Lin JT, Kinne RK, Walle T. Cellular uptake of dietary flavonoid quercetin 4'-beta-glucoside by sodium-dependent glucose transporter SGLT1. J Pharmacol Exp Ther. 2000. 294:837–843.