Abstract
Folate is generally considered as a safe water-soluble vitamin for supplementation. However, we do not have enough information to confirm the potential effects and safety of folate supplementation and the interaction with vitamin B12 deficiency. It has been hypothesized that a greater methyl group supply could lead to compensation for vitamin B12 deficiency. On this basis, the present study was conducted to examine the effects of high-dose folic acid (FA) supplementation on biomarkers involved in the methionine cycle in vitamin B12-deficient rats. Sprague-Dawley rats were fed diets containing either 0 or 100 µg (daily dietary requirement) vitamin B12/kg diet with either 2 mg (daily dietary requirement) or 100 mg FA/kg diet for six weeks. Vitamin B12-deficiency resulted in increased plasma homocysteine (p<0.01), which was normalized by dietary supplementation of high-dose FA (p<0.01). However, FA supplementation and vitamin B12 deficiency did not alter hepatic and brain S-adenosylmethionine (SAM) and S-adenosylhomocysteine (SAH) concentrations and hepatic DNA methylation. These results indicated that supplementation of high-dose FA improved homocysteinemia in vitamin B12-deficiency but did not change SAM and SAH, the main biomarkers of methylating reaction.
Vitamin B12 functions as a coenzyme in two reactions: the synthesis of methionine from homocysteine in the reaction catalysed by 5-methyltetrahydrofolate-dependent methionine synthase and the isomerization of L-methylmalonyl-CoA to succinyl-CoA in the reaction catalysed by L-methylmalonyl-CoA mutase (Ludwig & Matthews, 1997). Vitamin B12-deficiency has been shown to cause depletion of intracellular folate concentration in rat liver (Kutzbach et al., 1967) and human erythrocytes (Cooper & Lowenstein, 1964). Impaired activity of L-methylmalonyl-CoA mutase leads to a high-level of methylmalonic acid (MMA) in serum and urine, which is a useful biomarker of vitamin B12 deficiency (Baik & Russell, 1999; Stabler, 2001). Deficiencies of vitamin B12 and folate have been associated with brain-related disorders, including reversible dementia (Goodwin et al., 1983).
During vitamin B12-deficiency, decreased activity of methionine synthase may cause trapping of folate as 5-methylfolate. Therefore, the methyl group is not available to methylate homocysteine, which leads to high levels of plasma homocysteine and the decreased synthesis of methionine, S-adenosylmethione (SAM), and other metabolites of the methylation cycle (Baik & Russell, 1999; Stabler, 2001). Vitamin B12 deficiency is associated with megaloblastic anemia, neuropathy, and neuropsychiatric disorder (Baik & Russel, 1999). These symptoms may be linked to the block in homocysteine remethylation or the resulting homocysteinemia.
Although folate is a water-soluble vitamin with a low potential toxicity for supplementation and fortification (Bailey & Berry, 2005), there is a potential risk that it may mask undiagnosed vitamin B12-deficiency in pernicious anemia because folic acid (FA) supplementation may improve hematological indices but not the neurological disease (Lachance, 1998). The current animal studies reported negative or benefic effects of excess FA supplementation. FA supplementation may promote the development and progression of already existing, undiagnosed preneoplastic and neoplastic lesions (Kim, 1999). In rats, excess FA supplement (40 mg/kg diet) accelerated the development of colonic neoplasia in rodent model of cancer (Sohn et al., 2003). However, Kim et al. (1996) has reported that moderate-dose folate supplementation (8 mg folate/kg diet) leads to a reduction in the evolution of macroscopic colonic neoplasia, but high-dose FA supplementation (40 mg folic acid/kg diet) did not show the benefic effects. On the contrary, FA supplementation (5 mg folic acid/kg body weight per day) interferes with gastric carcinogenesis induced by N-methyl-N'-nitrosoguanidine in rats, and the authors suggested that the higher serum FA concentration of rats may play an important role in the prevention of gastric cancer (Fei et al., 2006). It is also hypothesized that a greater methyl group supply by FA supplementation could lead to compensation for vitamin B12-deficiency. The objective of this study was to investigate the effects of high-dose FA supplementation on biomarkers involved in the methionine cycle in vitamin B12-deficient rats.
Lactobacillus casei (7469) was obtained from American Type Culture Collection (Manassas, VA, USA). Folic acid depleted casein medium was obtained from Difco Laboratories (Detroit, MI, USA) and 7-fluoro-benzo-2-oxa-1, 3-diazole-4-sulfonate (SBDF) was obtained from Wako Chemicals (Osaka, Japan), respectively. L-Homocystine, tri-n-butylphosphine, DL-methionine, folic acid, SAM and SAH were purchased from Sigma (Sigma-Aldrich Chemical Co., St. Louis, MO, USA). CpG methylase was obtained from Perkin-Elmer (Waltham, MA, USA) and [3H-methyl]S-adenosylmethionine was purchased from Amersham Life Science (Picataway, NJ, USA). All chemicals were of the highest purity commercially available.
Animal experiments followed protocols approved by the NIH Guide for the Care and Use of Laboratory Animals (NIH Publications NO. 80-23, revised 1996). Male Sprague-Dawley rats (initial weight of 170-180 g) were obtained from SLC (Tokyo, Japan) and housed individually in wire-mesh cages with a daily light cycle from 0600 to 2000 hr and controlled temperature (20 ± 2℃) and humidity (50 ± 5%). After a 1-wk acclimation period, the rats were classified into four groups (n=8) by a randomised block design. They were fed semisynthetic powdered diets consisting of the following (g/kg): carbohydrate, 482 (corn starch, 340, dextrinzed cornstarch, 42, sucrose, 100); protein, 200 (casein, >85%); lipids, 50 (soybean oil); cellulose, 35; pectin, 50; and minerals plus vitamins (AIN93). We added 5% of pectin to the diets to accelerate vitamin B12-depletion (Cullen & Oace, 1989). Groups of rats were fed one of the following diets: 1) control diet (100 µg vitamin B12 and 2 mg FA/kg diet); 2) FA supplemented diet (100 µg vitamin B12 and 100 mg FA/kg diet) (we added 100 mg FA to the basal diet for providing 5 mg FA/kg body weight/day.) ; 3) vitamin B12-deficient diet (0 µg vitamin B12 and 2 mg FA/kg diet); 4) vitamin B12-deficient/ FA supplemented diet (0 µg vitamin B12 and 100 mg FA/kg diet). During the experiment, body weight was measured once a week and food intake was determined 3 times each week.
Twenty-four hr urine samples for methylmalonic acid (MMA) measurement were collected in tubes containing 5 ml of 0.4 N sulphuric acid on the 42nd day and stored at -20℃ until assay. Following 6 wks of feeding experimental diets, rats were anesthetized and blood was collected by heart puncture using a heparinized syringe. Blood was immediately centrifuged for 15 min at 3,000 rpm to collect plasma. Livers and brains were removed, weighed and rapidly frozen. Samples were stored at -70℃ until use.
Analysis of plasma homocysteine was performed using a modification of the liquid chromatographic method described by Araki and Sako (1987). Separation and quantification was carried out with a Shimadzu system with a LC-10AT pump, an RF-10AXL fluorescence detector, and a Chromatopac D-R6A integrator (Shimadzu, Kyoto, Japan). Homocysteine was separated with a Hypersil ODS analytical column (250×4.6 mm I.D., 5 µm particle size (Thermo-Keystone, Runcorn, Great Britain). LC was run isocratically with a 20 mM sodium acetate buffer, pH 4.0, containing 2% methanol, at a flow rate of 1 mL/min. Filtered samples (50 µl) were injected and the fluorescence intensity was measured with excitation at 385 nm and emission at 515 nm.
Folate was analysed by a microplate assay method using L. casei (ATCC 7469) according to Tamura (1990). Portions of liver were homogenized and autolysed for hydrolysis of γ-glutamyl residues in the presence of sodium ascorbate at 37℃, and the supernatants were used for folate assay.
Portions of frozen liver were homogenized with 0.4 M HClO4. Samples were centrifuged at 2000×g at 4℃ for 30 min. Each supernatant was filtered through a 0.45-µm filter. SAM and SAH were measured on a Shimadzu LC-10 HPLC equipped with 250×4.6 mm Ultrasphere 5-µm ODS Betasil analytical column (Thermo-Keystone, Runcorn, Great Britain) according to Wagner et al. (1984)
To assess the methylation status of CpG sites in genomic DNA, the in vitro methyl acceptance capacity of DNA was determined using [3H-methyl]SAM as a methyl donor and CpG DNA methylase according to Pogribny et al. (1999) Briefly, DNA (1 µg) purified from liver was incubated with 185 kBq of [3H-methyl]SAM, 4 units of Sss1 methyltransferase, 1 X Sss1 buffer (50 mmol/L NaCl, 10 mmol/L Tris-HCl, 10 mmol/L EDTA and 1 mmol/L dithiothreitol, pH 8.0). Samples were subsequently applied on DE-81 filters, washed, dried and counted using scintillation counter. The endogenous DNA methylation status is reciprocally related to the exogenous [3H-methyl] incorporation.
Methylmalonic acid was determined using the spectroscopic method by coupling with diazotized p-nitroaniline (Giorgio & Plaut, 1965).
Results were expressed as mean ± SE. Data were analyzed with a two-way ANOVA (dietary vitamin B12 and dietary folate) using SPSS 14.0 for window (SPSS, Inc., Chicago, IL, USA). When the ANOVA was significant, multiple comparisons between means were made using Duncan's multiple range test. Differences were considered significant at p<0.05.
In the present study, all the rats appeared to be healthy and FA-supplemented diets and vitamin B12-deficient diets did not alter food intake and body weight of the rats from control during the experimental periods (Table 1). However, rats fed vitamin B12-deficent/ FA-supplemented diets had slightly lower food efficiency ratio (g gain/g feed) compared to other experimental groups (p<0.05). Rats fed the vitamin B12-deficient diets excreted high levels of methylmalonic acid in urine (3976 ± 590 or 3570 ± 523 µmol/kg/d) (p<0.001), which indicates vitamin B12-deficiency induced (Table 2).
FA supplementation increased liver folate concentration significantly both in the rats fed vitamin B12-adequate diets (31.1 ± 5.4 vs. 23.1 ± 3.4 nmol/g) and in the rats fed vitamin B12-deficient diets (21.5 ± 2.2 vs. 14.2 ± 2.9 nmol/g) (p<0.01) (Table 2). However, FA supplementation increased plasma folate only in the rats fed vitamin B12-adequate diets (660.0 ± 112.4 vs. 252.8 ± 38.1 pmol/ml), but not in the rats fed vitamin B12-deficient diets (337.3 ± 179.2 vs. 334.5 ± 92.2 pmol/ml) (p<0.01).
Vitamin B12-deficient/folate-adequate diet increased plasma homocysteine compared to control group (7.65 ± 1.65 vs. 4.66 ± 0.62 nmol/ml) (p<0.01) (Fig. 1). FA supplementation normalized plasma homocysteine level from 7.65 ± 1.65 nmol/ml to 5.68 ± 1.43 nmol/ml (p<0.01).
Regarding the main biomarkers involved in the functioning of the methionine cycle, liver and brain SAM and SAH levels were not altered by vitamin B12-deficient diets or FA-supplemented diets (Table 3). In consequence, the SAM:SAH ratio remained unchanged by vitamin B12-deficiency and FA supplementation. Hepatic DNA methylation also was not altered by vitamin B12-deficiency and FA-supplementation (Table 3).
The present results showed that dietary supplementation of high-dose FA in vitamin B12-adequate and vitamin B12-deficienct rats did not affect body weight gain. It has been reported that pectin accelerates vitamin B12-deficiency by interfering with enterohepatic recycling of vitamin B12 and by stimulating microbial propionate production (Cullen & Oace, 1989). In this study, five percent of pectin was added to the basal diets to accelerate vitamin B12 depletion. Excretion of high levels of MMA in urine indicated that vitamin B12-deficiency was induced by feeding vitamin B12-deficient diet (p<0.001) (Table 2). Association between low vitamin B12 status and elevated urinary MMA excretion is well established (Stabler, 2001).
As expected, FA supplementation increased plasma folate and hepatic folate 2.6 folds and 1.3 folds in rats fed vitamin B12-adequate diets, respectively (p<0.001). Vitamin B12-deficieny decreased hepatic folate, but did not alter plasma folate (Table 2). Plasma folate was increased by FA supplementation only in the rats fed vitamin B12-adequate diets, not in the rats fed vitamin B12-deficient diet (p<0.01). These results suggest that vitamin B12 deficiency may cause 'methylfolate trapping', which leads to the impaired storage of intracellular folate in spite of relatively high plasma folate levels (Stabler, 2001).
Because vitamin B12 functions as a coenzyme for remethylation of homocysteine, vitamin B12 deficiency increased plasma homocysteine, implying less homocysteine being remethylated to methionine (Fig. 1). The present study demonstrated that dietary FA supplementation lowered plasma homocysteine to the control level in vitamin B12-deficient rats (Fig. 1). Plasma homocysteine is known as a biomarker for both vitamin B12 and folate status and plasma homocysteine is inversely related with folate status (Ubbink et al., 1993). Mildly elevated serum homocysteine levels have been considered to be an important risk factor for cognitive and neurological diseases, such as Alzheimer disease and Parkinson disease and brain ischemia (Boutell et al., 1998; Kuhn et al, 1998; Seshadri et al., 2002; White et al., 2001).
SAM is an essential component in many methylation reactions (Finkelstein, 1990). Both folate and vitamin B12 are involved in remethylation of homocysteine to methionine, which is a precursor of SAM, the primary methyl group donor for DNA, proteins, phospholipids, amines and neurotransmitters (Finkelstein, 1990; Selhub & Miller, 1992). SAM is converted to SAH after transfer of the methyl group. DNA methylation is an important epigenetic determinant in gene expression, the maintenance of DNA integrity and stability, chromatin modifications and the development of mutations (Jones & Baylin, 2002; Jones & Laird, 1999). In the rodent model, a definite effect of FA supplementation on SAM and SAH concentrations has not been established yet. In the present study, FA supplementation did not affect hepatic and brain SAM and SAH concentrations and SAM:SAH ratio, although FA supplementation increased hepatic folate concentration. Hepatic genomic DNA methylation was not significantly changed by vitamin B12-deficiency and FA supplementation. Therefore, the present results may indicate that high-dose FA supplementation in vitamin B12 deficiency may not affect the efficiency of methylation in the liver and brain, which implies "methylfolate trapping". Achón et al. (1999) showed that supranormal folate intakes may be associated with impairment in dietary protein metabolic utilization and this effect could be related with a xenobiotic behavior of FA at high levels. In accord with the present findings, a current study also reported that excess dietary FA (40 mg folate/kg diet) did not alter hepatic SAM and SAH in aged rats (Achón et al., 2007). We are speculating the reason why vitamin B12-deficiency did not alter most biomarkers of methylating reaction, plausibly due to the short experimental period of vitamin B12 depletion in this study.
In summary, dietary supplementation of high-dose FA improved homocysteinemia in vitamin B12-deficient rats but did not alter hepatic and brain SAM and SAH levels which are the main biomarkers of methylating reaction in rats, although FA supplementation increased hepatic folate concentration.
Figures and Tables
Fig. 1
Effect of high-dose FA supplementation on plasma homocysteine in rats fed vitamin B12-adequate or vitamin B12-deficienct diets in a 2×2 design. Values are expressed as means with their standard errors depicted by vertical bars (n=8). Two-way ANOVA; FA supplementation, p<0.01; vitamin B12, p<0.01. Different superscripts are significantly different at p<0.05 by Duncan's multiple-range test.
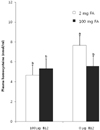
References
1. Achón M, Alonso-Aperte E, Ubeda N, Varela-Moreiras G. Supranormal dietary folic acid supplementation: effects on methionine metabolism in weanling rats. Br J Nutr. 2007. 98:490–496.


2. Achón M, Reyes L, Alonso-Aperte E, Ubeda N, Varela-Moreiras G. High dietary folate supplementation affects gestational development and dietary protein utilization in rats. J Nutr. 1999. 129:1204–1208.


3. Araki A, Sako Y. Determination of free and total homocysteine in human plasma by HPLC with fluorescence detection. J Chromatogr. 1987. 422:43–52.


5. Bailey LB, Berry RJ. Folic acid supplementation and the occurrence of congenital heart defects, orofacial clefts, multiple births, and miscarriage. Am J Clin Nutr. 2005. 81:1213S–1217S.


6. Boutell JM, Wood JD, Harper PS, Jones AL. Huntingtin interacts with cystathionine beta-synthase. Hum Mol Genet. 1998. 7:371–378.


7. Cooper BA, Lowenstein L. Relative folate deficiency of erythrocytes in pernicious anemia and its correction with cyanocobalmin. Blood. 1964. 24:502–521.


8. Cullen RW, Oace SM. Dietary pectin shortens the biological half-life of vitamin B-12 in Rats by increasing fecal and urinary losses. J Nutr. 1989. 119:1121–1127.


9. Fei SJ, Xiao SD, Peng YS, Chen XY, Shi Y. Chemopreventive effects of rofecoxib and folic acid on gastric carcinogenesis induced by N-methyl-N'-nitro-N-nitrosoguanidine in rats. Chin J Dig Dis. 2006. 7:134–140.


11. Giorgio AJ, Plaut GW. A method for the colorimetric determination of urinary methylmalonic acid in pernicious anemia. J Lab Clin Med. 1965. 66:667–676.
12. Goodwin JS, Goodwin JM, Garry PJ. Association between nutritional status and cognitive functioning in a healthy elderly population. JAMA. 1983. 249:2917–2921.


13. Jones PA, Baylin SB. The fundamental role of epigenetic events in cancer. Nat Rev Genet. 2002. 3:415–428.


14. Jones PA, Laird PW. Cancer epigenetics comes of age. Nat Genet. 1999. 2:163–167.
15. Kim YI. Folate and carcinogenesis: evidence, mechanisms, and implications. J Nutr Biochem. 1999. 10:66–88.


16. Kim YI, Salomon RN, Graeme-Cook F, Choi SW, Smith DE, Dallal GE, Mason JB. Dietary folate protects against the development of macroscopic colonic neoplasia in a dose responsive manner in rats. Gut. 1996. 39:732–740.


17. Kuhn W, Roebroek R, Blom H, van Oppenraaij D, Müler T. Hyperhomocysteinaemia in Parkinson's disease. J Neurol. 1998. 245:811–812.


18. Kutzbach C, Galloway E, Stokstad EL. Influence of vitamin B12 and methionine on levels of folic acid compounds and folate enzymes in rat liver. Proc Soc Exp Biol Med. 1967. 124:801–805.


20. Ludwig ML, Matthews RG. Structure-based perspectives on B12-dependent enzymes. Annu Rev Biochem. 1997. 66:269–313.
21. Pogribny I, Yi P, James SJ. A sensitive new method for rapid detection of abnormal methylation patterns in global DNA and within CpG islands. Biochem Biophys Res Commun. 1999. 262:624–628.


22. Selhub J, Miller JW. The pathogenesis of homocysteinemia: interruption of the coordinate regulation by S-adenosylmethionine of the remethylation and transsulfuration of homocysteine. Am J Clin Nutr. 1992. 55:131–138.


23. Seshadri S, Beiser A, Selhub J, Jacques PF, Rosenberg IH, D'Agostino RB, Wilson PW, Wolf PA. Plasma homocysteine as a risk factor for dementia and Alzheimer's disease. N Engl J Med. 2002. 46:476–483.


24. Sohn KJ, Stempak JM, Reid S, Shirwadkar S, Mason JB, Kim YI. The effect of dietary folate on genomic and p53-specific DNA methylation in rat colon. Carcinogenesis. 2003. 24:81–90.


25. Stabler SP. Bowman BA, Russell RM, editors. Vitamin B-12. Present Knowledge in Nutrition. 2001. 8th edition. Washington DC. USA: ILSE Press;230–240.


26. Tamura T. Picciano MF, Stokstad ELR, Gregory JF, editors. Microbiological assay of folate. Folic Acid Metabolism in Health and Disease. 1990. New York. USA: Wiley-Liss;121–137.
27. Ubbink JB, Vermaak WJ, van der Merwe A, Becker PJ. Vitamin B-12, vitamin B-6, and folate nutritional status in men with hyperhomocysteinemia. Am J Clin Nutr. 1993. 57:47–53.


28. Wagner J, Claverie N, Danzin C. A rapid high-performance liquid chromatographic procedure for the simultaneous determination of methionine, ethionine, S-adenosylmethionine, S-adenosylethionine, and the natural polyamines in rat tissues. Anal Biochem. 1984. 140:108–116.


29. White AR, Huang X, Jobling MF, Barrow CJ, Beyreuther K, Masters CL, Bush AI, Cappai R. Homocysteine potentiates copper- and amyloid beta peptide-mediated toxicity in primary neuronal cultures: possible risk factors in the Alzheimer's-type neurodegenerative pathways. J Neurochem. 2001. 76:1509–1520.

