Abstract
The aim of the present study was to assess the anti-obesity effects of grape seed extract (GSE) supplement in C57BL/6J mice. Thirty mice were divided into three groups; normal diet control group (ND), high fat diet control group (HD) and high fat diet plus grape seed extract supplemented group (HD+GSE). Results were as follows: 1. GSE supplement reduced the weight gain in mice fed high fat diets; epididymal and back fat weights were lower compared to non-supplemented HD group. 2. Blood lipid concentrations were lower in the HD+GSE group than in the HD group. Serum HDL-C concentrations were higher in the HD+GSE group compared with the other groups. 3. The concentrations of acid-insoluble acylcarnitines (AIAC) in serum and liver were higher in the HD+GSE group than in the HD group. 4. GSE supplementation increased mRNA levels of lipolytic genes such as carnitine palmitoyltransferase-1 (CPT-1) and decreased mRNA levels of lipogenic genes such as acetyl CoA carboxylase (ACC). These findings suggest that grape seed extract supplements in high fat diet might normalize body weight, epididymal and back fat weights, lipid concentrations, and carnitine levels through controlling lipid metabolism.
Obesity occurs when a controlled or regulated system fails to maintain energy balance, resulting in excess body fat storage. The obese population is increasing at an alarming rate throughout the world. Obesity causes many health problems, both independently and in association with other diseases (Formiguera & Canton, 2004). In particular, it is associated with the development of chronic diseases, including hypertension, type 2 diabetes mellitus, cardiovascular disease, osteoporosis, and certain forms of cancer. In addition to promoting obesity, diets with a dense caloric content are associated with an increased risk for developing type 2 diabetes (Petro et al., 2004). Animal models, in which obesity and type 2 diabetes are induced by feeding high caloric diet, are of increasing interest for developing new therapeutic strategies. Obesity is strongly associated with type 2 diabetes. The relative risk of developing diabetes increases by 25% for every 1 kg/m2 increase in BMI above 22 kg/m2 (Finer, 2006; James, 2004). Reducing body fat is a major health goal throughout the world and identifying functional foods that can help reduce body fat and meet other health needs has been the subject of numerous studies (Hasler, 2000); grape seeds are one of the functional ingredients of particular interest in recent years.
Grape is one of the most commonly consumed fruits in the world. It has various biological functions, due to its rich polyphenol ingredients, most of which are contained in its seeds (60-70%) and skin (30%). However, large quantities of grape seed wastes are produced annually by the food processing industry-wine, juice etc (Yoo et al., 2004). Grape ingredients also have healthful properties. Phenolic bioflavanoids from grape seeds and skins are powerful antioxidants. In particular, grape seeds contain two-thirds of the phenols of the grape, 5-8% by weight. Catechin, epicatechin and epicatechin gallate, and gallic acid are the monomeric compounds identified in grape seeds. These, along with dimers, trimers and oligomers of catechin and epicatechin are referred to as procyanidins (Yilmaz & Toledo, 2004). When used with either vitamins C or E, the extract possesses double the antioxidant capacity (Shi et al., 2003). Polyphenol in grape seeds have also been reported to have a variety of biological activities, including antioxidant, antithrombotic, and cardioprotective effects (Jang & Han, 2002; Sano et al., 2005; Pataki et al., 2002).
However, little is known about the effects of grape seed extracts on obesity in diet-induced C57BL/6J mice. Therefore, this study was designed to investigate the effects of grape seed extracts on changes in body weight gain, feed intake, lipid profiles, carnitine levels, and mRNA expressions of enzymes related to lipid metabolism in C57BL/6J mice with high fat diet induced obesity.
Grape seed extract (GSE) (main components: oligomeric proanthocyanidin complexes (OPCs)) (Gabetta et al., 2000) were supplied by Korea Hanlim Co. (Hanlim, Korea). According to the manufacturer, this GSE contained more than 80 procyanidolic value, potential cyanidol chloride (7%), monomer (30%).
Male C57BL/6J mice, aged 4 weeks, were purchased from Charles River Laboratories (Tokyo, Japan). The animals were maintained on a pellet diet (Research Diets, New Brunswick, NJ, USA) for 1 week, and then randomly divided into three groups: normal diet (ND) group, high fat diet (HD) control group, and one experimental group fed a high fat diet with grape seed extract (HD+GSE). Each group consisted of ten mice. Control group mice were orally administered 1ml of distilled water and the other treatment group (HD+GSE) mice were orally administered 1 ml of each extract dissolved in distilled water at the dose of 250 mg/kg body weight. The compositions of the experimental diets (Research Diets, New Brunswick, NJ, USA) are shown in Table 1. The animals were housed in a temperature-controlled environment with a 12-hour light/dark cycle. The animals were given free access to food and water during the entire experimental period. The feed intake and body weight were measured daily and weekly, respectively. The experimental protocol was approved by the Animal and Use Committee of Chonbuk National University (n.07040).
Feed was removed 12 hours before sacrificing. Blood samples were collected from each mouse by orbital/cardiac puncture and incubated on ice water for 1 hour. Serum was separated from the blood by centrifugation (Micro 17R, Vision Scientific Co., Seoul, Korea) at 1,100xg for 15 minutes at 4℃ and kept at -80℃ until analyzed. The liver, kidney, brain, back fat, muscle, and epididymal fat were removed, rinsed with a phosphate-buffered saline solution, wiped with a paper towel, weighed quickly, frozen in liquid nitrogen, and stored at -80℃ until assayed.
Total cholesterol (TC) in the serum and liver tissue was measured by an enzymatic method using a commercial kit (Asan Pharmaceutical Co., Seoul, Korea), The triglyceride (TG) in the serum and liver tissue was enzymatically measured with a commercial kit (Asan Pharmaceutical Co., Seoul, Korea). The high density-lipoprotein cholesterol (HDL-C) fraction was determined by the dextran sulfate-Mg++ method.
Liver tissues were homogenized by adding 50 mg of tissue to 1 ml of 0.3M PCA, centrifuging at 1,500xg and separating the supernatant. Nonesterified carnitine (NEC), acid-soluble acylcarnitine (ASAC), and acid-insoluble acylcarnitine (AIAC) in serum and liver tissues were determined by the radioimmunoenzymatic procedure of Cederblad and Lindstedt (1972), as modified by Sachan et al. (1984). A 100 µl sample was hydrolyzed with 200 µl of 0.6M PCA, centrifuged at 1,500xg for 10min. Acid soluble acyl carnitine (ASAC) and non-esterified carnitine (NEC) were determined in the supernatant and acid insoluble acyl carnitine (AIAC) was determined in the pellet. A 150 µl aliquot of the supernatant was neutralized with 1 M KHCO3 for measurement of NEC. In the ASAC assay, 100 µl of supernatant was hydrolyzed with 0.5 N KOH and neutralized with PCA/MOPS-II solution. The pellet containing AIAC was washed with 0.6 M PCA and drained, hydrolyzed in 0.5 N KOH for 60min in a water bath at 60℃, and neutralized with PCA/MOPS-I. The reaction mixture containing 1 M MOPS buffer, 0.1 M potassium ethyleneglycoltetraacetate, 0.1 M sodium tetrathionate, 0.1 mM [1-C14] acetyl CoA solution (Amersham, Little Chalfont, Buckinghamshire, England) was added to the supernatant in each sample, and reacted with 1 unit of carnitine acetyltransferase (Sigma Chemical Co., St. Louis, MO, USA) in a water bath at 37℃ for 30min. 200 µl of reacted supernatant was passed through a column with ion exchange resin (AG 1×8, 200-400 mesh), which collected the unreacted [1-C14] acetyl carnitine, and the C14 isotope was measured in a Beckman LS-3801 liquid scintillation counter (Beckman Instruments, Palo Alto, CA, USA). Total carnitine was calculated as the sum of NEC, ASAC, and AIAC values.
Total RNA was extracted with Trizol reagent. The concentration was confirmed with a spectrophotometer, and RT-PCR was performed using a one-step RT-PCR kit (ABgene, USA) for cDNA synthesis on a RT-PCR machine (MWG-BIOTECH, PRIMUS), and β-actin cDNA was also prepared as a control. The reaction finished and cDNA product was confirmed by 1.5% agarose gel electrophoresis. We confirmed gene expressions on acyl-CoA synthetase (ACS), carnitine palmitoyl transferase-I (CPT-I), and acetyl-CoA carboxylase (ACC). The relative intensity of all mRNAs was analyzed by using the AlphaEaseFC software (Alpha Innotech Corporation, San Leandro, CA, USA).
All values are expressed as mean ± standard deviation. The data were analyzed by one-way ANOVA using Statistical Package for Social Science (SPSS) version 16.0.1 program, and the differences among groups were assessed using Duncan's multiple range test. Statistical significance was considered at p<0.05.
As shown in Table 2, there were no significant differences in initial body weight among the groups. Final body weight and weight gain of the HD+GSE group was significantly lower than those of the HD group. Feed intake was significantly lower in the HD+GSE group compared to the HD group. Energy intake was significantly lower in the HD+GSE group compared to the HD group. In this study, weight loss of the HD+GSE group is involved in reduction of feed intake and energy intake. These results indicate a possibility that GSE supplementation have feeding control regulation.
As presented in Fig. 1., epididymal fat weight was significantly decreased in the HD+GSE group compared to the HD group. Back fat weight was also decreased significantly in the HD+GSE group compared to the HD group. These data showed that GSE supplementation reduces adipose tissue mass. In this study, weight loss of HD+GSE group is due to the reduction of body fat mass.
The effects of GSE supplementation on lipid concentrations in serum and liver were shown in Table 3. Serum triglyceride (TG) was significantly lower in the HD+GSE group compared to the HD group. Serum total cholesterol (TC) was significantly higher in the HD group than in the other groups. Serum HDL-cholesterol and HDL-cholesterol/total cholesterol (TC) ratio were significantly higher in the HD+GSE group compared to the other groups. Liver triglyceride (TG) and total cholesterol (TC) of HD+GSE group were significantly lower than those of the HD group.
Carnitine concentrations of serum and liver are shown in Table 4. The NEC concentrations in serum were highest in the HD+GSE group. The ASAC concentrations of the HD group were lowest. AIAC concentrations were significantly higher in the ND and HD+GSE group, and lowest in the HD group. TCNE concentrations were highest in the ND group and lowest in the HD group. The acyl/free ratio was highest in the ND group and was significantly lower in the HD and HD+GSE groups. Hepatic NEC concentrations were significantly higher in the ND group. The ASAC concentrations were not different among the groups. The concentrations of AIAC were highest in the HD+GSE group and lowest in the HD group. The hepatic TCNE concentrations were significantly higher in the ND group and lower in the HD group. The acyl/free ratio was not different among the groups.
Hepatic gene expressions are shown in Fig. 2. Acyl-CoA synthetase (ACS) mRNA expressions were not significantly different between the HD and HD+GSE group. However, ACS mRNA expressions were lower in the ND group than in other groups. Carnitine palmitoyl transferase-I (CPT-I) expression was slightly, but significantly, higher in the HD+GSE group than in the other groups. Acetyl-CoA carboxylase (ACC) was significantly higher in the HD group than in other groups, and significantly lower in the HD+GSE group than in other groups.
A variety of naturally occurring grape seed extracts (GSE) have been found to have beneficial effects on health, and these compounds have drawn attention because of their relative safeness and accumulated evidence of physiological properties, antioxidant efficacy, and anti-diabetic effects in animals and humans (Bagchi et al., 2000; Pinent et al., 2004; Yamakoshi et al., 2002). In this study, the antiobesity effects of GSE were investigated by evaluating body weight gain and feed intake, fat weight, and lipid and carnitine concentrations in serum and liver, as well as mRNA expressions in livers of high-fat diet-induced obese C57BL/6J mice. We used GSE at doses of 250 mg/kg/d to demonstrate the antiobesity effects of GSE.
High-energy diets are used widely and have been accepted in nutritional experiments as a good strategy to induce overweight conditions and fat deposition in animals (Kim et al., 2000). Thus, a prolonged period of high-energy feeding induces a marked weight increase in mice. In this study, HD feeding for 12 weeks resulted in obesity, which was associated with increased body weight and fat mass with development of hyperlipidemia. Although initial body weights of mice did not differ among the groups, final weight in the HD+GSE group was 6.65 g lower than that in the high-fat diet group, and feed intake and energy intake were reduced by 0.22 g and 1.17g, respectively, compared to the HD group. Dietary supplementation of GSE (proanthocyanidin) has been reported to have no significant does-independent effects in Sprague-Dawley rats (Kim et al., 2004). However, epididymal fat and back fat pad depositions were significantly lower in the HD+GSE group compared with the HD group. Thus, supplementation with GSE significantly reduced body weight, feed intake and adipose tissue mass in this study.
Additionally, triglyceride and total cholesterol concentrations in serum and liver were significantly lower in the HD+GSE group compared with the HD group. In this study, GSE supplementation decreased triglyceride and total cholesterol concentrations in serum and liver, and increased serum HDL-cholesterol and HDL-cholesterol/triglyceride ratio concentrations. Vinson et al. (2002) reported a significant dose-dependent reduction in serum total cholesterol (TC) and triglyceride (TG) concentrations in an atherosclerosis model supplemented with GSE.
Carnitine (β-hydroxy-γ-trimethylammonium butyrate) is an essential metabolite, which has a number of indispensable roles in intermediary metabolism. Carnitine is transported from the liver to other organs after biosynthesis in the liver from the amino acids lysine and methionine. Carnitine has an important role in the transport of activated long-chain fatty acids from the cytosol to the mitochondrial matrix, where β-oxidation takes place. Carnitine is involved in the transfer of the products of peroxisomal β-oxidation, including acetyl-CoA, to the mitochondria for oxidation in Krebs cycle (Bieber, 1988; Vaz & Wanders, 2002). This means that a lack of carnitine will slow oxidation of fatty acids and fuel an increase in serum lipid levels. In this study, the NEC concentrations in serum were significantly higher in the HD+GSE group than in the ND and HD groups. ASAC and AIAC concentrations in serum were significantly lower in the HD group than in the ND group. Especially, the concentrations of the carnitine fractions in serum were significantly higher in the HD+GSE (GSE administration) group than in the HD group. These changes resulted in a decreased acyl/free carnitine ratio in the HD group compared with the ND group. ASAC concentration and the acyl/free carnitine ratio in liver were not significantly different among groups. The AIAC concentration was significantly higher in the HD+GSE group than in the HD group. Mun et al. (2007) reported that the concentrations of carnitine fractions were significantly higher in the ND group than in the HD group. In our study, concentrations of carnitine fractions were significantly higher in the ND group than in the HD group. Carnitine concentrations in the blood and liver were increased in the GSE supplemented group. Thus, we assume that GSE administration increased β-oxidation of free fatty acids which were increased by high-fat dietary intake.
In evaluating the differences in lipid metabolism with GSE supplementation, we measured the hepatic ACS, CPT-I, and ACC mRNA levels of mice that were fed ND, HD and HD with GSE. Long-chain fatty acyl-CoA synthetase (ACS), as the first enzyme for fatty acid metabolism, plays a key role in both synthesis of cellular lipids and degradation of fatty acids via β-oxidation (Suzuki et al., 1990). Acyl-CoA synthetase (ACS) mRNA expressions were not significantly different between the HD and HD+GSE group. However, ACS mRNA expressions were lower in the ND group than in other groups.
The expression levels of ACS may modulate the metabolic flux of acyl-CoA in the liver and adipose tissue that utilize fatty acids for the generation or storage of energy (Ryu et al., 2003). Carnitine palmitoyltransferase-I (CPT-I) is located on the outer mitochondrial membrane and facilitates the movement of the long chain fatty acid (LCFA) into mitochondrial matrix. Therefore, it functions as a rate-limiting enzyme of β-oxidation in the mitochondria by having a key role in the transport of LCFA (McGarry et al., 1978). In our results, the hepatic CPT-I mRNA levels in the mice were slightly, but significantly, higher in the HD+GSE group than in the other groups. Earlier studies reported that hepatic CPT-I mRNA expression is increased by high fat diet with kochujang supplementation, suggesting that the control of CPT-I mRNA expression is a key feature in the regulation of fatty acid oxidation (Koo et al., 2008). Another study reported that CPT-I enzyme activity was increased by the up-regulation of CPT-I transcription that was mediated by genistein, soy isoflavone, and daidzein (Shin et al., 2006). The present study supports the results of these earlier studies by demonstrating increased hepatic CPT-I mRNA expression in the GSE supplemented group. Acetyl-CoA carboxylase (ACC), which catalyses an ATP dependent reaction is considered a synthesis pathway (Memon et al., 1999). The activity of ACC also modulates the rate of β-oxidation of fatty acid. Malonyl-CoA decreases the transport of LCFA acyl-CoA from cytoplasm to the mitochondrial matrix by inhibiting carnitine palmitoylacyltransferase I via an allosteric mechanism (McGarry et al., 1978). In our study, GSE supplementation decreased hepatic ACC mRNA levels. Malonyl-CoA, synthesized by ACC, is a potent CPT-I inhibitor as well as the first committed intermediate in the pathway of fatty acid biosynthesis (Bonnefont et al., 1999). These data support that high-fat diet or fasting causes a reduction in fatty acid synthesis by decreasing the synthesis of acetyl CoA carboxylase (Achouri et al., 2005). In this study, Fig. 2 showed that GSE supplement significantly increased hepatic lipolysis enzyme expression such as CPT-I, whereas significantly decreased lipogenic enzyme expression such as ACC. These results suggest that GSE supplementation decrease body fat weight by suppression of adipogenic enzyme such ACC and overexpression of lipolytic enzyme such as CPT-I.
In conclusion, we have demonstrated that GSE has beneficial effects and reverses some of the negative effects of a high fat diet. It provided evidence that GSE supplementation decreases body weight gain, feed intake, TG and TC in serum and liver. The study observed significant differences in carnitine concentrations among carnitine fractions (NEC, ASAC, AIAC, TCNE). Although there were few differences in gene expressions, GSE supplementation increased hepatic CPT-I mRNA concentrations above those of the ND group. GSE supplementation decreased hepatic ACC mRNA concentrations below those of the ND group. Finally, the effects of GSE on high-fat diet-induced obese mice indicate that grape seed extract possesses potential antiobesity effects and may relieve obesity-related symptoms, including hyperlipidemia, cardiovascular diseases and insulin resistance.
Figures and Tables
Fig. 1
Adipose tissue mass of mice fed experimental diets for 12weeks. Mean ± SD of 10 mice per group. Values with different superscripts are significantly different by ANOVA with Duncan's multiple range test at p<0.05. ND: Normal diet, HD: High fat diet, HD+GSE: High fat diet plus grape seed extract
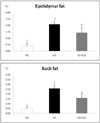
Fig. 2
Hepatic gene expressions. Mean ± SD of 10 mice per group. Values with different superscripts are significantly different by ANOVA with Duncan's multiple range test at p<0.05. ND: Normal diet, HD: High fat diet, HD+GSE: High fat diet plus grape seed extract
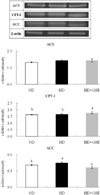
References
1. Achouri Y, Hegarty BD, Allanic D, Becard D, Hainault I, Ferre P, Foufelle F. Long chain fatty acyl-CoA synthetase expression is induced by insulin and glucose: Involvement of sterol regulatory element-binding protein-1c. Biochimie. 2005. 87:1149–1155.


2. Bagchi D, Bagchi M, Stohs SJ, Das DK, Ray SD, Kuszynski CA, Joshi SS, Pruess HG. Free radicals and grape seed proanthocyanidin extract: importance in human health and disease prevention. Toxicology. 2000. 148:187–197.


4. Bonnefont JP, Demaugre F, Prip-Buus C, Saudubray JM, Brivet M, Abadi N, Thuillier L. Carnitine palmitoyltransferase deficiencies. Mol Genet Metab. 1999. 68:424–440.


5. Cederblad G, Lindstedt S. A method for the determination of carnitine in the picomole range. Clin Chim Acta. 1972. 37:235–243.


7. Formiguera X, Canton A. Obesity: epidemiology and clinical aspects. Best Pract Res Clin Gastroentenol. 2004. 18:1125–1146.


8. Gabetta B, Fuzzati N, Griffini A, Lolla E, Pace R, Ruffilli T, Peterlongo F. Characterization of proanthocyanidins from grape seeds. Fitoterapia. 2000. 71:162–175.


11. Jang JK, Han JY. The antioxidant ability of grape seed extracts. Korean Journal of Food Science and Technology. 2002. 34:524–528.
12. Kim H, Hall P, Smith M, Kirk M, Prasain JK, Barnes S, Grubbs C. Chemoprevention by grape seed extract and genistein in carcinogen-induced mammary cancer in rats is diet dependent. J Nutr. 2004. 134:3445S–3452S.


13. Kim JY, Nolte LA, Hansen PA, Han DH, Ferguson K, Thompson PA, Holloszy JO. High-fat diet-induced muscle insulin resistance: relationship to visceral fat mass. Am J Physiol Regul Integr Comp Physiol. 2000. 279:R2057–R2065.


14. Koo BS, Seong SH, Kown DY, Sohn HS, Cha YS. Fermented kochujang supplement shows anti-obesity effects by controlling lipid metabolism in C57BL/6J mice fed high fat diet. Food Sci Biotechnol. 2008. 17:336–342.
15. McGarry JD, Leatherman GF, Foster DW. Carnitine palmitoyltransferase I. The site of inhibition of hepatic fatty acid oxidation by malonyl-CoA. J Biol Chem. 1978. 253:4128–4136.


16. Memon RA, Fulle J, Moser AH, Smith PJ, Grunfeld C, Feingold KR. Regulation of putative fatty acid transporters and acyl-CoA synthetase in liver and adipose tissue in ob/ob mice. Diabetes. 1999. 48:121–127.


17. Mun EG, Soh JR, Cha YS. L-Carnitine reduces obesity caused by high-fat diet in C57BL/6J mice. Food Sci Biotechnol. 2007. 16:228–233.
18. Pataki T, Bak I, Kovacs P, Bagchi D, Das DK, Tosaki A. Grape seed proanthocyanidins improved cardiac recovery during reperfusion after ischemia in isolated rat hearts. Am J Clin Nutr. 2002. 75:894–899.


19. Petro AE, Cotter J, Cooper DA, Peters JC, Surwit SJ, Surwit RS. Fat, carbohydrate, and calories in the development of diabetes and obesity in the C57BL/6J mouse. Metabolism. 2004. 53:454–457.


20. Pinent M, Blay M, Blade MC, Salvado MJ, Arola L, Ardevol A. Grape seed-derived procyanidins have an antihyperglycemic effect in streptozotocin-induced diabetic rats and insulinomimetic activity in insulin-sensitive cell lines. Endocrinology. 2004. 145:4985–4990.


21. Ryu MH, Cha YS. The effects of a high-fat or high-sucrose diet on serum lipid profiles, hepatic acyl-CoA synthetase, carnitine palmitoyltransferase-I, and the acetyl-CoA carboxylase mRNA levels in rats. J Biochem Mol Biol. 2003. 36:312–318.


22. Sachan DS, Rhew TH, Ruark RA. Ameliorating effects of carnitine and its precursors on alcohol-induced fatty liver. Am J Clin Nutr. 1984. 39:738–571.


23. Sano T, Oda E, Yamashita T, Naemura A, Ijiri Y, Yamakoshi J, Yamamoto J. Anti-thrombotic effect of proanthocyanidin, a purified ingredient of grape seed. Thromb Res. 2005. 115:115–121.


24. Shi J, Yu J, Pohorly JE, Kakuda Y. Polyphenolics in grape seeds-biochemistry and functionality. J Med Food. 2003. 6:291–299.


25. Shin ES, Cho SY, Lee EH, Lee SJ, Chang IS, Lee TR. Positive regulation of hepatic carnitine palmitoyl transferase 1A (CPT1A) activities by soy isoflavones and L-carnitine. Eur J Nutr. 2006. 45:159–164.


26. Suzuki H, Kawarabayasi Y, Kondo J, Abe T, Nishikawa K, Kimura S, Hashimoto T, Yamamoto T. Structure and regulation of rat long-chain acyl-CoA synthetase. J Biol Chem. 1990. 265:8681–8685.


28. Vinson JA, Mandarano MA, Shuta DL, Bagchi M, Bagchi D. Beneficial effects of a novel IH636 grape seed proanthocyanidin extract and a niacin-bound chromium in a hamster atherosclerosis model. Mol Cell Biochem. 2002. 240:99–103.
29. Yamakoshi J, Saito M, Kataoka S, Kikuchi M. Safety evaluation of proanthocyanidin-rich extract from grape seeds. Food Chem Toxicol. 2002. 40:599–607.


30. Yilmaz Y, Toledo RT. Health aspects of functional grape seed constituents. Trends in Food Science & Technology. 2004. 15:422–433.


31. Yoo MA, Chung HK, Kang MH. Evaluation of physicochemical properties in different cultivar grape seed waste. Food Sci Biotechnol. 2004. 13:26–29.