Abstract
Aloin is a physiologically active anthraquinone present in aloe. There are two isomers of aloin, aloin A and aloin B, occurring as a mixture of diastereomers. The objective of this study was to determine the bioavailability and tissue distribution of aloin. Rats were gavaged with 11.8g/kg aloin, and the levels of aloin and its conjugates were measured in plasma, tissues, and urine. Plasma aloin level showed a peak at 1hr after the administration and the concentration was 59.07±10.5 ng/ml. The 24 h cumulated urinary aloin was 0.03% of the initial dose. These results suggest that aloin is absorbed and reaches a peak plasma level within 1-1.5 h after the administration and a significant portion is possibly metabolized or is excreted in feces. These results can apply to the determination of the adequate intake level of aloe and aloe products to achieve the desired biological effect, and to interprete in vitro study results.
Aloe is widely used in food products, pharmaceuticals, and cosmetics due to its aromatic and therapeutic properties (Davis, 1994; Korkina, 2003). Aloe products have been used to alleviate inflammation and accelerate wound healing and its products ranked first in health food sales of 2005 (Park et al., 2005). Biologically active substrates in aloe include anthraquinone, anthrone derivatives, polysaccharides and chromones (Tom, 2004). Although aloe has been studied for its efficacy, the pharmacokinetic properties of its ingredients are largely unknown. Aloin is the major anthraquinone of aloe exudates and gels (Groon & Reynols, 1986) and it is characterized as the C-glycoside of aloe-emodin (Fig. 1.) (Reynold, 1985). Biological effects of aloin have been examined in a limited number of studies. Aloin increased the alcohol oxidation rate in rats (Chung et al., 1996), inhibited AOM-induced DNA adduct formation in rats (Shimpo et al., 2003), and had cytotoxicity against human breast cancer cell lines (Esmat et al., 2006). Even with these biological functions, the aloin content in aloe products is strictly regulated due to its possible adverse effects including laxative property (Koch, 1996) and cytotoxicity (Avila et al., 1997). Since these biological efficacies of aloin depend on its bioavailability, it is important to understand the absorption and tissue distribution of aloin as a basis for analyzing its benefits and risks. To produce data useful in interpreting many studies regarding in vitro efficacy and toxicity, we investigated the fate of oral aloin intake in rats.
Aloin, β-D-glucuronidase and sulfatase were purchased from Sigma-Aldrich Co. (St. Louis, MO, USA). All other chemicals and solvents (HPLC grade) were obtained from Waters Korea Co. (Seoul, KOREA). The standard stock solution of aloin was made in 70% methanol and stored at -80℃ until use.
Male Sprague-Dawley rats were obtained at 7 weeks of age from Jung-Ang Lab Animal Inc. (Seoul, Korea) and maintained in air-conditioned quarters with 12-hr light/dark cycles. They were given a commercial rat food (Jung-Ang Lab Animal Inc., Seoul, Korea) and water ad libitum. The experiments started after the rats were given at least 1 week to adapt to their new diet and environment. A fresh dose of aloin solution was made with water and administered at dose of 11.8 g/kg. Blood samples were collected from the heart at 0.5, 1, 1.5, 3, 5, 8, 12, 24, 48, and 72 h after the aloin was administered, and placed in heparinized tubes. The blood samples were centrifuged at 2,000 × g for 10 min. The plasma was stored at -80℃ until it was used.
Rats were randomly divided into 11 groups (four rats per group), and given aloin gavage at a dose of 11.8 g/kg. To collect urine, rats were kept in metabolic cages. The animals were sacrificed at 0, 0.5, 1, 1.5, 3, 5, 8, 12, 24, 48, and 72 h after the aloin administration. Liver, kidney, and intestine (20 cm from the stomach) were removed and homogenized with 1 ml of 0.4 M sodium phosphate buffer containing 6 mg of ascorbic acid and 0.5 mg of Na2EDTA (final pH of 6.5). Tissue homogenates were centrifuged at 16,000 × g for 5min, and 200 µl of the supernatant was used to analyze aloin. The results were expressed on the basis of the weight of wet tissues (µg/g). Urine samples were collected before and at 0-24, 24-48, and 48-72 h after the aloin administration. The urine samples were stored at -80℃ until they were used.
The levels of aloin in rat plasma, tissues, and urine were determined by HPLC. The HPLC system consisted of a Waters 600 Controller, Waters 600 Pump, and Waters 486 Detector (Waters Korea Co., Seoul, Korea). The sample was incubated with β-D-glucuronidase and sulfatase at 37℃ for 45 min. The reaction mixture was then extracted with ethyl acetate, and the extract was dried under LN2. The residues were reconstituted in 200 µL of 50% methanol. After the resultant solution was centrifuged at 12,000 × g for 10 min, 15 µL of aliquots were injected into the HPLC column. A Symmetry C18, 4.6'250 mm, column was used with a mobile phase of methanol-water (50:50) at a flow rate of 0.9 ml/min and detection at 220 nm.
The plasma concentration-time curves of aloin and its glucuronides after the administration of aloin are shown in Fig. 2. The plasma samples obtained from rats were analyzed for the presence of both free and conjugated aloin. In rats receiving aloin (11.8 g/kg), plasma levels were detectable as early as 0.5 h, and peaked at 1 h followed by a subsequent decline. The plasma concentration at peak level was 59.07 ± 10.5 ng/ml. The plasma concentrations of both free and conjugates at different time points are given in Table 1. Fifty percent of total aloin in plasma was in the form of aloin glucuronide.
The tissue levels of aloin over time are shown in Fig. 3. The peak levels of aloin in liver and intestine were 77.15 ± 15.92 ng/g and 102.37 ± 14.12 ng/g, respectively, at 0.5 h (Table 2). The levels declined rapidly within 90 min. Aloin concentration in the kidney was maximized as 12.46 ± 0.81 ng/g at 5 h (Table 2).
Urinary aloin and its conjugates were excreted within 72 h of the administration (Table 3). Most of the urinary aloin was excreted within 24 h. The cumulative amount of aloin excreted in the urine during the first 24 h was 0.03% of the administrated dose. The cumulative excretion of aloin A and aloin B within 24 h amounted to 2.8 ± 1.1 µg/day and 0.42 ± 0.21 µg/day, respectively. These values correspond to 64.97 and 9.7% of the administered doses, respectively.
The aim of this study was to evaluate the absorption and urinary excretion of aloin to gain a better understanding of its bioavailability and tissue distribution as a basis to interpret in vitro studies, and to determine effective level of intake. To determine its in vivo bioavailability, we used 11.8 g/kg which was LOAEL of aloin (Zhou et al., 2003).
In this study, the level of aloin and their conjugates in plasma peaked at 1 h suggesting a rapid absorption of aloin in the gastrointestinal tract. At the peak plasma aloin level, conjugates of both aloin A and aloin B levels were higher compared to the levels of free aloin A and aloin B. The AUC0-24hr of aloin was determined as 270.81 ± 59.1 ng·h/ml and the half lives of aloin was 1.47 ± 0.24 hr. To maintain a high concentration in plasma, it requires a repeated ingestion of aloin every 1.5 h. The published studies in cell culture system used 50-2500 µM of aloin (Esmat et al., 2005; Wamer et al., 2003). However, the mean peak plasma level was 0.026 µM after the consumption of 11.8 g/kg. Thus this value could serve as a reference for designing and interpreting in vitro experiments for elucidating the mechanisms of action of aloin. Recently, several studies have shown that oral intake leads to anti-cancer (Shimpo et al., 2003) and anti-diabetic effects (Bolkent et al., 2004; Can et al., 2004). These are evidences to support either that the low aloin concentrations are adequate to induce biochemical changes, or that localized tissue aloin is metabolized to more active form(s).
Many in vitro studies have used high concentrations of aloin to test their efficacy presuming that most of aloin consumed would enter the circulation. However, a limited number of studies have been conducted for intestinal metabolism of anthraquinones. Our preliminary in vitro experiment using Caco-2 cell model showed that the absorption rate of aloin was about 6.6% which was similar to the absorption rate of other polyphenols (unpublished data). Due to the β-glycosidic linkage between the sugar and anthranoid structure, the glycoside form is protected against both hydrolysis by acid in the stomach and α-glucosidase activity in the small intestine (Wolffram et al., 2002). Results showed that native aloin as well as glucuronate or sulfate conjugates was identified in the jejunum indicating that aloin is absorbed in the small intestine and subjected to the action of various conjugation enzymes present in the small intestine. There are very few data on intestinal metabolism of anthraquinone. Although the absorption mechanisms of anthraquinones as glycosides are not clear, glycosidic polyphenols are transported into enterocyte by intestinal glucose transporters (GLUTs and SGLT) (Olthof et al., 2000) and can be hydrolyzed by a cytosolic β-glucosidase (Day et al., 1998). Another pathway involves the lactase phloridzine hydrolase (LPH), on the outside of the brush border membrane of the small intestine that catalyzes extracellular hydrolysis of glucosides (Day et al., 2000). Both enzymes are probably involved although their relative contribution for various glucosides is not clear. Moreover, part of them are transported by efflux into the intestinal lumen by multidrug resistance-associated protein 2 (MRP2) (Walle et al., 1999). Both phase I and phase II detoxification enzymes are expressed in the enterocytes. Glycosidic polyphenols are converted to polar β-glucuronides by phase II UDP-glucuronyltransferase (UGTs) (Jäger et al., 1998). These indicated that the absorption and metabolism of glycosidic polyphenols is complex, and requires further investigation.
The liver receives aloin from the mesenteric vein. We analyzed the aloin levels in the liver at several sampling times (0.5, 1.5, and 5 h after aloin gavage). After a short period of time, aloin was detectable in the liver (Table 2). The presence of a high proportion of glucuronidated aloins in rat liver possibly suggests that a significant extent of metabolism occurs in the liver. Since we measured total aloin and not aloe-emodin, aloin may also be converted to aloe-emodin by liver enzymes. Aloe-emodin can be biotransformed to 2-hydroxyemodin in the liver by cytochrome P450 1A2 (Mueller et al., 1998). In pharmacokinetic-metabolic studies with aloe-emodin, 15% of the dose was excreted in urine and the rest in feces (Maity et al., 2001). In this study, glucuronic acid or sulfate conjugated forms of aloin were also detected in the kidney, although aloin presented as an unconjugated form in the majority of tissues (intestine, liver and kidney). These results indicate that aloin undergoes deconjugation process when it enters the tissue. In liver cells, β-glucuronidase is responsible for the metabolism of polyphenol glucuronides (O'Leary et al., 2003).
Native aloin as well as its glucuronidated or sulfated metabolites was present in urine and their excretion rate was relatively low as compared to the amount ingested. In this study, urinary recovery of aloin was 0.03% of the oral intake. This finding is in agreement with the results of several other investigations which found that excretion rate of polyphenols in urine were between 0.005-0.1% of the intake (Bub et al., 2001; Chen et al., 1997; Matsumoto et al., 2001). Excretion percentages of aloin A and aloin B in urine were 64.7 and 9.7% of total aloin, respectively, indicating that aloin B would be more effective form of aloin than aloin A in rats. In a study using tea cathechins, β-elimination half-lives of epigallocatechin-gallate (EGCG) was greatest followed by epigallocatechin (EGC) and then epicatechin (EC), and EGCG is known as a strong antioxidant, anti-inflammatory and anti-cancer agent in green tea (Kim et al., 2006; Landis-Piwowar et al., 2007; Rice-Evans et al., 1995). These studies indicate that larger proportion of compounds circulating is possibly associated with its biological activity in vivo. In other bioavailability studies of polyphenols, they often reached the peak plasma level at 1-2 h after oral administration and then were rapidly decreased (Chen et al., 1997; Chow et al., 2001). In agreement with these results, aloin is also rapidly taken up and then excreted. Consequently, the fast excretion of aloin is facilitated by the conjugation of the aglycon to sulfate and glucuronide groups like other polyphenols. These pharmacokinetic parameters are useful for selecting the dose and dose frequency of aloe consumption for human studies.
This study confirmed that aloin was absorbed in its unchanged glycated forms in vivo. Further work is required to characterize the aloin metabolites and asses their potential biological activity at practical plasma concentrations.
Figures and Tables
Fig. 2
Plasma concentration-time curve of aloin in rats after gavage administration of aloin at 11.8 g/kg rat body weight. Results are expressed as mean ± SD on a sample size of four rats/group
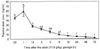
Fig. 3
Tissue concentration of aloins (aloin A, aloin B, conjugates) in rats after gavage administration of aloin at 11.8 g/kg rat body weight. Results are expressed as mean ± SD on a sample size of four rats/group.
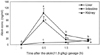
Fig. 4
Urinary excretion of aloins (aloin A, aloin B, conjugates) in rats after gavage administration of aloin at 11.8 g/kg rat body weight. Results are expressed as mean±SD on a sample size of four rats/group.
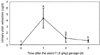
Table 2
Tissue concentration of aloins (aloin A, aloin B, conjugates) in rats after aloin (11.8 g/kg) gavagea)
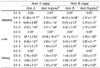
References
1. Avila H, Rivero J, Herrera F, Fraile G. Cytotoxicity of a low molecular weight fraction from Aloe vera (Aloe barbadensis Miller) gel. Toxicon. 1997. 35:1423–1430.


2. Bolkent S, Akev N, Ozsoy N, Sengezer-Inceli M, Can A, Alper O, Yanardag R. Effect of Aloe vera (L.) Burm. Fil. Leaf gel and pulp extracts on kidney in type-II diabetic rat models. Indian J Exp Biol. 2004. 42:48–52.
3. Bub A, Watzlm B, Heeb D, Rechkemmer G, Briviba K. Malvidin-3-glucoside bioavailability in humans after ingestion of red wine, dealcoholized red wine and red grape juice. Eur J Nutr. 2001. 40:113–120.


4. Can A, Akev N, Ozsoy N, Bolkent S, Arda BP, Yanardag R, Okyar A. Effect of Aloe vera leaf gel and pulp eatracts on the liver in type-II diabetic rat models. Biol Pharm Bull. 2004. 27:694–698.


5. Chen L, Lee MJ, Li H, Yang CS. Absorption, distribution, elimination of tea polyphenols in rats. Drug Metab Dispos. 1997. 25:1045–1050.
6. Chow HH, Cai Y, Alberts DS, Hakim I, Dorr R, Shahi F, Crowell JA, Yang CS, Hara Y. Phase I pharmacokinetic study of tea polyphenols following single-dose administration of epigallocatechin gallate and polyphenon E. Cancer Epidemiol Biomarkers Prev. 2001. 10:53–58.
7. Chung JH, Cheong JC, Lee JY, Roh HK, Cha YN. Acceleration of the alcohol oxidation rate in rats with aloin, a quinine derivative of Aloe. Biochem Pharmacol. 1996. 52:1461–1468.


8. Davis RH, DiDonato JJ, Johnson RW, Stewart CB. Aloe vera, hydrocortisone, and sterol influence on wound tensile strength and anti-inflammation. J Am Podiatr Med Assoc. 1994. 84:614–621.


9. Day AJ, Cañada FJ, Díaz JC, Kroon PA, Mclauchlan R, Faulds CB, Plumb GW, Morgan MR, Williamson G. Dietary flavonoid and isoflavone glycosides are hydrolysed by the lactase site of lactase phlorizin hydrolase. FEBS Lett. 2000. 25:166–170.


10. Day AJ, DuPont MS, Ridley S, Rhodes M, Rhodes MJ, Morgan MR, Williamson G. Deglycosylation of flavonoid and isoflavonoid glycosides by human small intestine and liver beta-glucosidase activity. FEBS Lett. 1998. 25:71–75.
11. Esmat AY, El-Gerzawy SM, Rafaat A. DNA ploidy and S phase fraction of breast and ovarian tumor cells treated with a natural anthracycline analog (aloin). Cancer Biol Ther. 2005. 4:108–112.


12. Esmat AY, Tomasetto C, Rio MC. Cytotoxicity of a natural anthraquinone (aloin) against human breast cancer cell lines with and without ErbB-2: topoisomerase II alpha coamplification. Cancer Biol Ther. 2006. 5:97–103.


13. Groon QJ, Reynols T. Barbaloin in aloe species. Planta Med. 1987. 53:345–348.
14. Jäger W, Zembsch B, Wolschann P, Pittenauer E, Senderowicz AM, Sausville EA, Sedlacek HH, Graf J, Thalhammer T. Metabolism of the anticancer drug flavopiridol, a new inhibitor of cyclin dependent kinases, in rat liver. Life Sci. 1998. 62:1861–1873.


15. Kim SH, Park HJ, Lee CM, Choi IW, Moon DO, Roh HJ, Lee HK, Park YM. Epigallocatechin-3-gallate protects toluene diisocyanate-induced airway inflammation in a murine model of asthma. FEBS Lett. 2006. 580:1883–1890.


16. Koch A. Metabolism of aloin-the influence of nutrition. J Pharm Biomed Anal. 1996. 14:1335–1338.
17. Korkina L, Suprun M, Petrova A, Mikhal'chik E, Luci A, De Luca C. The protective and healing effects of a natural antioxidant formulation based on ubiquinol and Aloe vera against dextran sulfate-induced ulcerative colitis in rats. Biofactors. 2003. 18:255–264.


18. Landis-Piwowar KR, Huo C, Chen D, Milacic V, Shi G, Chan TH, Dou QP. A novel prodrug of the green tea polyphenol(-)-epigallocatechin-3-gallate as a potential anticancer agent. Cancer Res. 2007. 67:4303–4310.


19. Maity TK, Mandal SC, Bhakta T, Pal M, Saha BP. Metabolism of 1,8-dihydroxy 3-hydroxy methyl anthraquinone (aloe-emodin) isolated from the leaves of Cassia tora in albino rats. Phytother Res. 2001. 15:459–460.


20. Matsumoto H, Inaba H, Kishi M, Tominaga S, Hirayama M, Tsuda T. Orally administered delphinidin 3-rutinoside and cyanidin 3-rutinoside are directly absorbed in rats and humans and appear in the blood as the intact forms. J Agric Food Chem. 2001. 49:1546–1551.


21. Mueller SO, Stopper H, Dekant W. Biotransformation of the anthraquinones emodin and chrysophanol by cytochrome P450 enzymes. Bioactivation to genotoxic metabolites. Drug Metab Dispos. 1998. 26:540–546.
22. O'Leary KA, Day AJ, Needs PW, Mellon FA, O'Brien NM, Williamson G. Metabolism of quercetin-7- and quercetin-3-glucuronides by an in vitro hepatic model: the role of human beta-glucuronidase, sulfotransferase, catechol-O-methyltransferase and multi-resistant protein 2 (MRP2) in flavonoid metabolism. Biochem Pharmacol. 2003. 65:479–491.
23. Olthof MR, Hollman PC, Vree TB, Katan MB. Bioavailabilities of quercetin-3-glucoside and quercetin-4'-glucoside do not differ in humans. J Nutr. 2000. 130:1200–1203.


24. Park YG, Park MY, Sung MK, Kwon H. Study on the intake pattern of health intended foods depending on inclusion of proclaimed health functional food materials. Journal of the Korean Society of Food Science and Nutrition. 2005. 34:374–379.


25. Reynold T. The compounds in Aloe leaf exudates: a review. Bot J Lin Soc. 1985. 90:157–177.
26. Rice-Evans CA, Miller NJ, Bolwell PG, Bramley PM, Pridham JB. The relative antioxidant activities of plant-derived polyphenolic flavonoids. Free Radic Res. 1995. 22:375–383.


27. Shimpo K, Chihara T, Beppu H, Ida C, Kaneko T, Hoshino M, Kuzuya H. Inhibition of azoxymethane-induced DNA adduct formation by Aloe arborescens var. natalensis. Asian Pac J Cancer Prev. 2003. 4:247–251.
28. Tom R. Aloes-the genus aloe. 2004. Florida. USA: CRC press;40–47.
29. Walle UK, Galijatovic A, Walle T. Transport of the flavonoid chrysin and its conjugated metabolites by the human intestinal cell line Caco-2. Biochem Pharmacol. 1999. 58:431–438.


30. Wamer WG, Vath P, Falvey DE. In vitro studies on the photobiological properties of aloe emodin and aloin A. Free Radic Biol Med. 2003. 34:233–242.


31. Wolffram S, Block M, Ader P. Quercetin-3-glucoside is transported by the glucose carrier SGLT1 across the brush border membrane of rat small intestine. J Nutr. 2002. 132:630–635.


32. Zhou Y, Feng Y, Wang H, Yang H. 90-day subchronic toxicity study of aloe whole-leaf powder. Wei Sheng Yan Jiu. 2003. 32:590–593.