Abstract
BACKGROUND/OBJECTIVES
Ultraviolet radiation (UV) is a major cause of skin photoaging. Previous studies reported that ethanol extract (PET) of Prunus persica (L.) Batsch flowers (PPF, peach flowers) and its subfractions, particularly the ethylacetate (PEA) and n-butanol extracts (PBT), have potent antioxidant activity and attenuate the UV-induced matrix metalloproteinase (MMP) expression in human skin cells. In this study, we investigated the protective activity of PPF extract against UV-induced photoaging in a mouse model.
MATERIALS/METHODS
Hairless mice were treated with PET or a mixture of PEA and PBT either topically or orally along with UV irradiation. Histological changes and biochemical alterations of mouse skin were examined. Major phenolic compounds in PPF extract were analyzed using an ACQUITY UPLC system.
RESULTS
The overall effects of topical and oral treatments with PPF extract on the UV-induced skin responses exhibited similar patterns. In both experiments, the mixture of PEA and PBT significantly inhibited the UV-induced skin and epidermal thickening, while PET inhibited only the UV-induced epidermal thickening. Treatment of PET or the mixture of PEA and PBT significantly inhibited the UV-induced MMP-13 expression, but not typeⅠ collagen expression. Topical treatment of the mixture of PEA and PBT with UV irradiation significantly elevated catalase, superoxide dismutase (SOD) and glutathione-peroxidase (GPx) activities in the skin compared to those in the UV irradiated control group, while oral treatment of the mixture of PEA and PBT or PET elevated only catalase and SOD activities, but not GPx. Thirteen phytochemical compounds including 4-O-caffeoylquinic acid, cimicifugic acid E and B, quercetin-3-O-rhamnoside and kaempferol glycoside derivatives were identified in the PPF extract.
The UV radiation spectrum contains three regions: UVA (400–320 nm), UVB (320–290 nm), and UVC (290–200 nm). Since UVC is almost completely absorbed by the ozone layer, the wavelengths that reach the earth's surface are UVA and UVB [1], which are considered the causative agents that damage the DNA, protein and lipid structures in the skin [2]. UVB is the main cause of sunburn and probably the most carcinogenic component of sunlight as well [34].
Skin aging is a complex and progressive process leading to functional and aesthetic changes in the skin with both intrinsic and extrinsic factors being responsible for the outcome [5]. Extrinsic skin aging is due to environmental aggressors, such as UV radiation, stress or smoking. However, it is mainly caused by repeated exposure to UV from the sun, called photoaging. Skin photoaging is characterized by coarse and deep wrinkles, thickness, roughness, dyspigmentation and histological changes [678]. Wrinkle formation is known to be closely associated with the degradation of the extracellular matrix (ECM) of the skin, and UV radiation induces the degradation of ECM via complex signaling events including collagen fragmentation and matrix metalloproteinases (MMPs) secretion [6].
MMPs, a group of zinc and calcium-dependent endopeptidases, are involved in the degradation of the ECM components including collagen, elastin, and fibrillin-1 [9]. Increased MMPs and/or decreased collagen production results in loss of collagen content in the skin, ultimately inducing wrinkle formation [10]. MMPs are activated by excessive oxidative stress together with inflammatory responses [11]. Recent studies have demonstrated that inhibition of MMP production suppresses UV-induced epidermal thickening and wrinkle formation in mouse skin. Therefore, the regulation of MMPs is considered an effective strategy for the prevention and treatment of photo-aging [12].
Both endogenous and exogenous reactive oxygen species (ROS) damage the skin cell proteins, leading to photoaging of skin [13]. UV irradiation produces ROS in the skin, which in turn activates the mitogen-activated protein kinase cascade leading to increased expression of MMP-1 and MMP-3, and eventually results in collagen breakdown [14]. Generally, increased ROS is removed by the elaborate antioxidant defense system including catalase, superoxide dismutase (SOD) and glutathione-peroxidase (GPx). However, excessive accumulation of ROS leads to a state of oxidative stress [15]. Many researchers have reported that antioxidant materials isolated or extracted from natural herbs attenuate the risk of skin damage or photoaging induced by UV irradiation in vitro and in vivo [1617181920].
Peach flowers (Prunus persica (L.) Batsch flowers, PPF) is consumed as an herbal tea in Korea. Topical application of PPF extract has long been used in Korea as a traditional remedy to alleviate skin disorders including rashes and eczema [2122]. However, scientific researches supporting their biological functions are lacking. Some in vivo and in vitro studies reported that the ethanol/methanol or acetone extract of PPF prevented UVB-induced DNA damage [23] and arachidonate metabolite release [22]. Reports also indicate that the PPF extract prevented LPS-stimulated inflammation in macrophages through its potent antioxidant activity [24].
In our previous study, we observed that the PPF ethanol extract and its sub-fractions, particularly the ethylacetate and n-butanol extracts, ameliorated signs of skin photoaging by reducing the ROS generation and expression of MMPs in UV-irradiated keratinocytes. In addition, the ethylacetate and n-butanol extracts also significantly inhibited the UV-induced downregulation of typeⅠ collagen expression in human dermal fibroblasts [2526].
Based on these results, this study undertook to investigate whether topical application or oral administration of the PPF extract exhibited a protection against UV-induced biochemical alterations leading to skin damage in hairless mice. We also analyzed the major phenolic compounds present in the PPF extract to gather basic data for future research to study the biologically active compounds in PPF extract.
Fresh peach flowers were steamed for a few minutes and air dried for use as a tea product. Dried peach flowers for tea were obtained from a flower tea farm (NAERI ENJOO Flower Tea, Whasoon, Korea) in May 2015. The obtained dried peach flowers were freeze-dried for 24 h in our lab using a freeze-drying machine (Samwon, Seongnam, Korea), and then ground into powder. A voucher specimen of Prunus persica (L.) Batsch (2005-0076) was deposited at the Korea University Herbarium. Powdered PPF was extracted in 10 times volume (v/v) ethanol (Ducksan, Ansan, Korea) with stirring for 24 h at room temperature and this procedure was repeated twice. The combined supernatant was passed through Whatman™ filter paper (No.2; Fisher Scientific, Pittsburgh, USA) and concentrated using a rotary vacuum evaporator (Eyela, Tokyo, Japan). One part of the concentrated PPF ethanol extract was suspended in water, partitioned sequentially by hexane, dichloromethane, ethylacetate, n-butanol and an aqueous layer from the nonpolar toward the polar direction, and the fractions were concentrated. All concentrated extracts were lyophilized and stored at −20℃. The lyophilized ethanol extract of PPF (PET), ethylacetate fraction (PEA) and n-butanol fraction (PBT) were used as the test samples for this study. The yield of PET, PEA, and PBT sample from the freeze-dried peach flowers was 26.7%, 2.1%, and 7.7%, respectively.
Seven-week-old female albino hairless (Skh-1) mice were obtained from Orient Bio Inc. (Seoul, Korea). The mice were acclimated for 1 week prior to the study and had free access to food and water. All experimental protocols were approved by the Institutional Animal Care and Use Committees (No.16-0037-S1A0) at Seoul National University Hospital. Two sets of experiments were carried out separately according to the sample treatment route, namely topical application and oral administration. In the topical experiment, mice were divided into four groups (n = 6/group): (i) sham-irradiated vehicle-treated mice (normal control; T-NC), (ii) UV-irradiated and vehicle-treated mice (UV irradiated control; T-UV), (iii) UV-irradiated and 1.0% PET treated mice (T-PET), and (iv) UV-irradiated and 1.0% mixture of PEA and PBT treated mice (T-PM). The vehicle used was ethanol/polyethylene glycol (3:7, v/v). Sample (2 mg/200 µL/mouse) was applied to the dorsal skin topically 5 times/week for 9 weeks. In the oral administration experiment, mice were divided into four groups (n = 6/group): (i) sham-irradiated vehicle (2.5% dimethyl sulfoxide)-treated mice (O-NC), (ii) UV-irradiated and vehicle-treated mice (O-UV), (iii) UV-irradiated and PET-treated mice (O-PET), and (iv) UV-irradiated and mixture of PEA and PBT treated mice (O-PM). Vehicle (2.5% DMSO, 100 µL) or sample (100 mg/kg BW) was administered orally to the mice using a zonde 5 days a week for 10 weeks. Since the yields of PEA and PBT were low and our previous in vitro studies had shown similar results for both, the mixture of PEA and PBT for the T-PM or O-PM group were prepared in their respective vehicles at a ratio of 1:4(PEA:PBT) corresponding to the extraction yield ratio.
For the topical experiment, the mouse dorsal skin was irradiated with UV, 3 times/week for 8 weeks, starting from the 2nd week of the experiment. UV irradiation was initiated with 100 mJ/cm2 (one minimal erythema dose, MED) and the dose was gradually increased weekly to a final dose of 2.5 MED. The total irradiation dose for the topical experiment was 3,360 mJ/cm2. For oral administration, the UV strength was maintained at 1 MED for the 1st week, 1.5 MED for the 2nd week, 2 MED for the 3rd week and 2.5 MED for the remaining weeks. UV irradiation was provided by four fluorescent sun lamps (TL 20W/12 RS, Philips, Netherlands) emitting a continuous spectrum between 275 and 380 nm (peak: 290–320 nm). A Kodacel filter (TA 401/407, Kodak, Rochester, NY, USA) was used to block the wavelengths ≤ 290 nm (UVC). The UV dosage was quantified using a UV meter (Variocontrol, Herbert Waldmann, Villingen-Schwenningen, Germany). Body weight was measured weekly. At the last day of each experiment, mice were anesthetized by an intramuscular injection of a mixture of zoletil (Virbac, Carros, France) and xylazine (Rompun, Bayer, Leverkusen, Germany), and a biopsy of the dorsal skin tissue was collected. Skin samples were then prepared for staining or biochemical measurements.
Just before sacrifice, the mouse dorsal skin was lifted by pinching gently, and the skinfold thickness was measured at the midway between the neck and hips using a digital caliper (Mitsutoyo, Kanagawa, Japan).
Mouse skin samples were fixed overnight at 4℃ with 4% paraformaldehyde in phosphate buffered saline (PBS), and then embedded in paraffin. Paraffin sections (4 µm thick) were mounted on silane-coated slides and stained with H&E. Imaging was done using a model D70 microscopic digital camera (Olympus, Tokyo, Japan) connected to a BX51 light microscope (Olympus). The epidermal thickness was measured at 10 random sites in each photograph using the Image J analysis software (NIH, Bethesda, MD, USA).
Mouse skin samples were homogenized and total RNA was extracted at 4℃ in TRIZOL reagent (Ambion-Thermo Fisher Scientific, MA, USA) using TissueLyser (Quiagen, Valencia, CA, USA) and 5 mm stainless steel beads (Qiagen, Valencia, CA, USA). The isolated RNA was reverse-transcribed into cDNA using the PrimeScript™ Reverse Transcriptase reagent kit (Takara Bio Inc., Otsu, Japan) according to the manufacturer's instructions. The mRNA expression of MMP-13 and typeⅠ collagen (Collagen Ⅰ)was determined using a 7500 real-time quantitative PCR system (Applied Biosystems, Foster City, USA) and a SYBR green detection system (Roche, Mannheim, Germany). PCR was performed using the following primers: MMP-13 (mouse) forward 5′-CAT CCA TCC CGT GAC CTT AT-3′, and reverse 5′-GCA TGA CTC TCA CAA TGC GA-3′; Collagen I (mouse) forward 5′-TCG TGA CCG TGA CCT TGC G-3′ and reverse 5′-GAG GCA CAG ACG GCT GAG TAG-3′; 36B4 (endogenous reference) forward, 5′-TGG GCT CCA AGC AGA TGC-3′, and reverse, 5′-GGC TTC GCT GGC TCC CAC-3′. Data were analyzed using the 2−ΔΔCT method [27] and expressed as fold changes of gene expression relative to 36B4.
Mouse skin samples were lysed in RIPA lysis buffer (Sigma-Aldrich, St. Louis, USA) containing a protease and phosphatase inhibitor cocktail (Roche, Basel, Switzerland) using the TissueLyser (Qiagen) at 4℃. The protein concentration was determined by the Pierce bicinchoninic acid (BCA) protein assay kit (Thermo Scientific, Rockford, IL, USA). For Western blotting, equal amounts of protein were separated on 8~10% sodium dodecyl sulfate-polyacrylamide gel electrophoresis (SDS-PAGE) and then transferred to PVDF membranes (GE Healthcare Life Sci., Germany). The membrane was blocked in 5% skim milk in Tris-buffered saline containing 0.1% Tween-20 (TBST, pH 8.0), and incubated with primary antibodies against MMP-13 (Thermo Fisher Scientific, Waltham, MA, USA), collagen TypeⅠ (Calbiochem, EMD Millipore Co., Temecula, CA, USA) and β-actin (Santa Cruz Biotechnology) for 18 h at 4℃. The bound antibodies were detected with horseradish peroxidase-conjugated secondary antibody. Blotting protein was developed by enhanced chemiluminescence solution (Tanslab, Daejeon, Korea) and visualized using a Luminescent Image Analyzer (FujiFilm, Tokyo, Japan).
Mouse skin samples were homogenized using the TissueLyser (Qiagen) in 9 volumes of cold PBS at 4℃, and centrifuged 10,000 g for 15 min at 4℃. The supernatant was collected and evaluated for the antioxidant enzyme activity and protein concentration. The catalase activity, determined by measuring the reduced absorbance at 240 nm for 1 min according to the method of Aebi [28], was expressed as nmole/min/mg protein. Cu/Zn-SOD activity was determined by measurement of the absorbance change for 2 min at 420 nm [29]. The SOD activity was expressed as a unit (U)/mg protein. One unit of enzyme activity was defined as the amount of enzyme required to inhibit the rate of pyrogallol oxidation by 50%. GPx activity was determined by the method of Tappel [30] using cumene hydroperoxide as a substrate. The oxidation rate of NADPH was monitored at 340 nm. GPx activity was expressed as nmole/min/mg protein. The protein concentration was determined using the Pierce BCA protein assay kit (Thermo Scientific).
Mouse skin samples were homogenized in 9 volumes of cold PBS and centrifuged at 8,000 g for 15 min at 4℃. The total supernatant was used to measure the pro-inflammatory cytokine levels. Interleukin (IL)-1β, IL-6 and tumor necrosis factor (TNF)-α concentrations were determined using ELISA kits (R&D Systems, Minneapolis, USA) according to the manufacturer's instructions. The concentration of each cytokine was normalized to the protein concentration in the skin sample.
Major phytochemical compounds existing in PET, PEA and PBT, were analyzed using an ACQUITY UPLC system (Waters, Milford, MA, USA) equipped with BEH C18 column (100 × 2.1 mm, 1.8 µm; Waters) equilibrated with water containing 0.1% formic acid. The solubilized sample in 70% methanol was eluted in a gradient consisting of acetonitrile containing 0.1% formic acid at a flow rate of 0.4 mL/min. The eluted compounds were analyzed by a Q-TOF/MS (Waters) in the electrospray ionization-positive mode. The voltages of the capillary and sampling cones were set at 3.0 kV and 30 V, respectively. The temperatures of the source and desolvation were set at 120℃ and 300℃, respectively, and the desolvation flow rate was maintained at 800 L/h. TOF-MS data were collected in the m/z 50–1200 range with a scan time of 0.2 sec. The MS/MS spectra of the metabolites were collected by a collision energy ramp from 20–45 eV. All data including retention time, m/z, and ion intensity were analyzed using the MarkerLynx software 4.1 (Waters Corp. Milford, MA, USA). Data processing was performed by UNIFI 1.8 with a traditional medicine library (Waters Corp. Milford, MA, USA). The compounds were identified using the human metabolome databases (www.hmdb.ca) and the METLIN database (metlin.scripps.edu).
Since UV leads to edema and epidermal cell proliferation, changes in skin and epidermal thickness are commonly used to evaluate UV-induced skin responses [1419]. In this study, we measured skinfold thickness and epidermal thickness to assess the effect of topical treatment with PPF extract on skin responses in UV irradiated mice. The H&E stained skin images of each group are shown in Fig. 1A. We observed that epidermal thickness was significantly increased (P < 0.05) in the UV control group compared to that in the normal control group by 64.2% (Fig. 1B). However, this UV-induced epidermal thickening was significantly inhibited (P < 0.05) by topical treatment with PET or the mixture of PEA and PBT by 22.7% and 15.0%, respectively (Fig. 1B). Skinfold thickness measured using a caliper in the UV control group was also significantly increased (P < 0.05) by 20.4% compared to that in the normal group (Fig. 1C). However, topical treatment with the mixture of PEA and PBT significantly inhibited (P < 0.05) this UV-induced skin thickening by 7.7%, while PET treatment tended to inhibit as well, but without significance (Fig. 1C). These results demonstrate that topical treatment with PPF extract, particularly the mixture of PEA and PBT, could attenuate the UV-induced skin thickening and epidermal thickening in mice.
To investigate whether the topical treatment of PPF extract affects the expression of collagenase expression in the skin tissue of UV-irradiated mice, we analyzed MMP-13 expression, a functional substitute for MMP-1, in mouse skin [12]. UV radiation significantly elevated MMP-13 mRNA expression by 100% (P < 0.05), while this induction was significantly inhibited by topical treatment with PET or the mixture of PEA and PBT by 64.0%, and 92.7%, respectively, when compared to the UV control group (Fig. 2A). Conversely, UV irradiation did not affect typeⅠ collagen mRNA expression. However, the average typeⅠ collagen mRNA expressions in the T-PET group and the T-PM group were much higher than that in the UV control group by 88.5%, and 127.6%, respectively, albeit not significant due to a large deviation within the group (Fig. 2A). Western blot results showed that UV irradiation or topical treatment with PPF extract did not significantly affect MMP-13 or typeⅠ collagen protein expression (Fig. 2B, 2C). These results demonstrate that topical treatment with PET or the mixture of PEA and PBT attenuate the UV-induced MMP-13 expression.
Generally, activities of antioxidant enzymes in the skin, such as catalase, SOD, and GPx, are important parameters to assess the antioxidant capacity in organisms [31]. To investigate whether topical treatment of PPF extract inhibited the UV-induced change of antioxidant capacity in the skin, we measured the activities of catalase, SOD, and GPx in mouse skin after PPF extract treatment with UV-irradiation. Irradiation significantly decreased the activities of SOD and GPx (P < 0.05) as compared with the normal control group by 26.6%, and 49.6%, respectively, while no effect was observed on the catalase activity (Fig. 3). Topical treatment with the mixture of PEA and PBT significantly increased (P < 0.05) the activities of catalase, SOD, and GPx compared with those in the UV control group by 39.5%, 33.0%, and 77.3%, respectively, whereas increase in activity by PET treatment was not significance (Fig. 3). These results demonstrate that topical treatment with PPF extract, particularly the mixture of PEA and PBT, protects the antioxidant enzymes such as catalase, SOD, and GPx in the skin against damage caused by UV irradiation.
H&E stained skin images of each group in the oral treatment experiment are shown in Fig. 4A. We observed that the epidermal thickness significantly increased by 197% (P < 0.05) in the UV control group compared to that in the normal control group (Fig. 4B). However, oral treatment with PET or the mixture of PEA and PBT considerably inhibited (P < 0.05) this UV-induced epidermis thickening by 13.7%, and 22.6%, respectively (Fig. 4B). UV irradiation also increased the skinfold thickness by 54.5% (P < 0.05). However, oral treatment with the mixture of PEA and PBT significantly inhibited the UV-induced skinfold thickening by 9.8% (P < 0.05), while PET treatment reduced the thickening without any significance (Fig. 4C). These results demonstrate that oral administration of PPF extract, particularly the mixture of PEA and PBT, attenuates the UV-induced skin thickness and epidermal hyperplasia in mice.
In the orally treated group, UV irradiation significantly elevated the MMP-13 mRNA expression in mouse skin samples by 106% as compared to the normal control group (P < 0.05) (Fig. 5A). However, oral treatment with PET or mixture of PEA and PBT markedly reduced the UV-induced MMP-13 mRNA expression by 61.9%, and 53.9%, respectively (P < 0.05) (Fig. 5A). On the other hand, UV irradiation did not affect typeⅠ collagen mRNA expression. Interestingly, the average typeⅠ collagen mRNA expressions in the O-PET group and the O-PM group were higher than that in the UV control group by 59.9%, and 82.5%, respectively, albeit not significant (Fig. 5A). Western blotting showed that UV irradiation significantly increased the MMP-13 protein expression by 125% compared to that in the normal control group (P < 0.05), but oral treatment with PET or the mixture of PEA and PBT completely inhibited this induction (P < 0.05). UV irradiation or oral treatment with PPF extract treatment did not affect typeⅠ collagen protein expression (Fig. 5B, 5C). These results demonstrate that oral treatment with PET or the mixture of PEA and PBT attenuates the UV-induced MMP-13 mRNA expression and protein production in mice skin.
In the oral treatment experiment, UV irradiation significantly decreased the activities of SOD and GPx (P < 0.05) in mouse skin compared with that in the normal control group by 27.4%, and 34.7%, respectively, while it was ineffective on catalase activity (Fig. 6A). However, compared to the UV control group, oral treatment significantly increased (P < 0.05) the activities of catalase and SOD with PET treatment (25.6%, and 38.3%, respectively), and mixture of PEA and PBT (42.5%, and 84.6%, respectively). Conversely, the UV-induced reduction of GPx activity remained unaffected by oral treatment with PET or the mixture of PEA and PBT (Fig. 6A). These results demonstrate that oral treatment with PET or the mixture of PEA and PBT protects the antioxidant enzymes in the skin against damage caused by UV irradiation.
We investigated whether oral intake of PPF extract prevents the release of UV-induced pro-inflammatory cytokines in mouse skin since our previous study had revealed that antioxidant and anti-inflammatory activities of PET, PEA, and PBT in skin cells [26]. In this study, UV irradiation significantly increased the IL-1β, IL-6 and TNF-α concentrations in the mouse skin samples by 69.5%, 148.1%, and 97.8%, respectively (Fig. 6B). However, oral treatment of PET significantly reduced (P < 0.05) UV-induced TNF-α by 34.8%, and tended to reduce the IL-1β and IL-6 concentrations, although not significantly. Oral treatment of the mixture of PEA and PBT significantly reduced (P < 0.05) UV-induced IL-1β concentration by 49.1%, and tended to reduce UV-induced IL-6 and TNF-α concentrations without significance (Fig. 6B). These results demonstrate that oral treatment with PET or the mixture of PEA and PBT attenuates the UV-induced pro-inflammatory cytokine production, particularly TNF-α or IL-1β, in mouse skin.
To find a potential photo-protective component in the PPF extract, we analyzed the major phytochemicals present in PET, PEA, and PBT. The HPLC chromatogram is presented in Fig. 7. We identified 13 phenolic compounds (Table 1), many of them common in all samples. This could be because PEA and PBT are sub-fractions of PET, and the extracting solvent for PEA (ethylacetate) and PBT (n-butanol) are not very different in polarity. The common phenolic compounds (peaks 1–7) identified in PET, PEA, and PBT were 4-O-caffeoylquinic acid, cimicifugic acid E, quercetin-3-O-rhamnoside, kaempferol glucoside derivatives, cimicifugic acid B and multiflorin B. Menisdaurin (peak 8) and 6-methyl[2-oxo-3[(2-oxo-chromen-7-yl)oxy]-2H-chromen-7-yl β-D-glucopyranoside (peak 12) were present in PET and PBT, but not in PEA.
In our recent study, we reported that when ethanol extract of peach flowers was sequentially fractionated with hexane, dichloromethane, ethylacetate, n-butanol and water, PEA contained the highest level of polyphenol (394.6 mg tannic acid/g) and flavonoids (253.7 mg rutin/g), followed by PBT (128.3 mg tannic acid/g, and 93 mg rutin/g, respectively) and PET (78.1 mg tannic acid/g, and 55.3 mg rutin/g, respectively) [25]. The strength of antioxidant activity of these samples highly correlated with the total phenolic content, when valuated by the 2,2-diphenyl-1 picrylhydrazyl (DPPH) and 2,2′-azinobis-3-ethyl-benzothiazoline-6-sulfonic acid (ABTS) radical scavenging activity assays [25]. Interestingly, Liu et al. [32] demonstrated that the contents of both total phenolics and flavonoids decreased during blossoming of peaches. They also reported a significant correlation between the antioxidant capacities and contents of total phenolics and total flavonoids, as well as chlorogenic acid, cinnamic acid and kaempferol-3-O-galactoside [32].
It is well known that UVB irradiation leads to cutaneous edema, hyperplasia, erythema, leukocyte infiltration, dilation of dermal blood vessels and vascular hyperpermeability [19], which together result in epidermal proliferation and skin thickening [12]. Generally, skin photo-damage manifests as skin thickening, reduction in skin elasticity and formation of wrinkles. These are fundamentally associated with alteration and reduction in collagen typeⅠ, the primary component of the dermal layer of skin [18]. In this study, we observed that both topical application and oral administration of the mixture of PEA and PBT significantly attenuated the UV-induced skin and epidermal thickening, implying that this sample treatment protects the skin from photo-damage and possibly formation of wrinkles.
UV-induced MMPs in the skin degrade the ECM components including collagen, and thereby need to be repaired quickly to maintain the normal structural integrity of the dermis. In the absence of perfect repair, MMP-mediated collagen damage is expected to accumulate with each successive UV exposure. Such cumulative collagen damage is likely the major contributor to the phenotype of photoaged human skin [15]. Since collagens provide resiliency and strength to the skin, collagen degradation promotes skin aging. Most collagen fibers in the dermis of human skin are typeⅠ and Ⅲ collagens [233], and UV-induced MMP-1 initiates a cleavage at a single site within its central triple helix. Once cleaved by MMP-1, collagen is further degraded by MMP-3 and MMP-9 [15]. In addition to degradation of the dermal collagen induced by MMP, UV irradiation impairs the ongoing collagen synthesis, primarily through down- regulation of the collagen gene expression. Thus, the balance of MMPs and collagen synthesis is considered to play an important role in maintaining the integrity of ECM in the skin. In the current animal model experiment, PET or the mixture of PEA and PBT inhibited the UV-induced MMP-13 mRNA expression (a major collagenolytic enzyme in mouse skin), but not typeⅠ collagen expression in both topical and oral treatment. It is interesting that the typeⅠ collagen mRNA expression was not significantly suppressed by UV irradiation, but the treatment of PET or the mixture of PEA and PBT tended to increase the average of typeⅠ collagen mRNA expression, albeit not significantly, which may be due to a large deviation within the group. Our results support that the theory that the beneficial effects of PET and the mixture of PEA and PBT might be associated with inhibition of UV-induced epidermal hyperplasia or skin edema and an increase in collagenase production, mainly MMP-13 in mouse skin.
UVA and UVB mainly stimulate the production of ·O2− through the activation of NADPH oxidase and respiratory chain reactions. Several ROS elimination systems have developed in mammalian tissues to protect cells. SOD catalyzes the dismutation of ·O2− into O2 and H2O2, and catalase breaks down H2O2 into O2 and H2O. The combination of SOD and catalase completely scavenges ·O2− initiating ROS. GPx also breaks down H2O2 in the presence of reduced glutathione, and decomposes lipid hydroperoxides into their corresponding alcohols [11]. These enzymes are able to efficiently scavenge the ROS and thereby protect skin from damage in the normal state. However, excessive and chronic exposure to UV radiation can overwhelm the cutaneous antioxidant capacity leading to oxidative damage, thereby resulting in skin photo-aging [31]. The present animal study revealed that UV irradiation induced a notable decrease in the antioxidant enzyme activities such as SOD and GPx in mouse skin. Subsequently, topical application and oral administration with PET or the mixture of PEA and PBT prevented the UV-induced decrease of the antioxidant capacity in the skin through the modulation of catalase, SOD, and/or GPx activities. It might therefore be suggested that PET and the mixture of PEA and PBT prevent the direct damage of antioxidant enzymes in the skin caused by UV irradiation, and/or mitigate the consumption of antioxidant enzymes through the scavenging of ROS generated by UV irradiation.
UV radiation stimulates an inflammatory response that results in erythema, edema, and an influx of inflammatory cells such as neutrophils and lymphocytes. Macrophages that infiltrate into the epidermis and dermis secrete cytokines, which exert immunosuppressive properties, to help resolve the UV-induced inflammatory response [34]. UV-induced ROS stimulates the production of pro-inflammatory mediators, such as TNF-α, IL-1β, IL-6, IL-8, IL-10, and PGE2 in keratinocytes. These cytokines stimulate the epidermal keratinocytes and dermal fibroblasts, up-regulate MMPs and degrade dermal collagen and elastic fibers that lead to the formation of wrinkles [343536]. In the present study, we examined the effect of oral treatment with PET or the mixture of PEA and PBT on IL-1β, IL-6 and TNF-α productions in UV-irradiated mouse skin. The mixture of PEA and PBT significantly inhibited UV-induced IL-1β release, while PET significantly inhibited UV-induced TNF-α release. It is known that when the skin is exposed to UV, IL-1α and IL-1β from the stratum corneum initiate the inflammatory response [31]. Since UV induced inflammation further activates the transcription of MMPs [14], the anti-inflammatory effect of PET and the mixture of PEA and PBT may contribute to the suppression of MMP-13 expression.
Since numerous phenolic phytochemicals are known to have antioxidant properties, it is anticipated that they would protect the skin cells against oxidative damage caused by ROS which is rapidly generated by UV irradiation [13]. In several studies, in vitro or in vivo studies involving treatment with epigallocatechin-gallate from tea, flavanol-rich cocoa, resveratrol from grapes, green tea polyphenol or extract of teas markedly attenuated the UVB-induced skin photoaging via reduction of oxidative stress, sunburns, erythema, pro-inflammatory cytokine production and expression of MMPs [23337]. However, there is a paucity of reports on the analysis of biochemical components in peach flowers. Four kaempferol glycoside derivatives were reported to be isolated from PPF ethanol extract [22]. Recently, high levels of cyanidin-based and pelargonidin-based compounds have been identified in the pink petals of peach flowers, but are absent in the white petals [38]. In the full-flowering stage, chlorogenic acid was the most predominant component, accounting for 62.08-71.09% of the total amount of identified phenolic compounds in peach flowers, followed by kaempferol-3-galactoside, kaempferol-4-glucoside, and quercetin-3-rhamnoside [32].
In this study, we identified 13 phytochemical compounds in the PPF extract, including 4-O-caffeoylquinic acid, cimicifugic acid E and B, quercetin-3-O-rhamnoside and kaempferol glycoside derivatives. Chlorogenic acid is 3-O-caffeoylquinic acid, and isomers of chlorogenic acid include the caffeoyl ester at other hydroxyl sites on the quinic acid ring: 4-O-caffeoylquinic acid and 5-O-caffeoylquinic acid. Chlorogenic acid is known to possess antioxidant, anticancer, anti-inflammatory, and immunomodulatory properties in diverse systems. It is worth mentioning the reports where chlorogenic acid dramatically inhibited the proteolytic activity of MMP-9, which degrades typeⅣ collagen [39]. Cimifugic acids was also reported to prevent collagen degradation by collagenases under pathologic conditions, wound healing, or inflammation [40]. Quercetin is the most common flavonoid in nature, and is mainly present in the glycoside form. In several studies, quercitrin (quercetin 3-Orhamnoside) was revealed to protect against UV-induced oxidative stress, inflammatory response or cell death [34142]. In a recent study, we observed that quercitrin treatment at 40 and 100 µM prior to UV irradiation significantly inhibited UV-induced MMP-1 expression and down-regulation of typeⅠ collagen in HDFs (not published). Maini et al., [43] demonstrated that when quercetin and kaempferol were applied topically to artificial skin mimic tissue (EpiDerm) prior to UVB exposure, these flavonoids significantly decreased UVB-induced cyclobutane thymine dimer formation, thereby reducing direct DNA damage caused by UVB irradiation. From these results and reports, it could be suggested that the preventive effect of PPF extract against skin photoaging could be the result of the cooperative interactions of several phenolic compounds having antioxidant and collagen-protective activities, rather than any one compound.
Overall, this study showed that both topical and oral treatment with PET and the mixture of PEA and PBT prevented UV-induced skin damage in a very similar pattern, regardless of the route of administration. Particularly, the mixture of PEA and PBT showed higher preventive activity against UV-induced skin thickening and a decrease of antioxidant enzyme activity, which might be closely related to the difference in antioxidant activity strength. Our previous study had demonstrated that PEA and PBT had higher phenolic content and antioxidant activity than PET [26].
We could therefore conclude that topical application and oral administration of PET or the mixture of PEA and PBT attenuates the UV-induced epidermal thickening, MMP-13 expression, and pro-inflammatory cytokine production, possibly through their potent antioxidant activity to reduce the oxidative stress. These findings support the potential significance of PPF extract as an effective and natural product for prevention and treatment of skin aging.
Figures and Tables
Fig. 1
Effects of topical treatment of Prunus persica Flos (PPF) extract on UV-induced epidermal thickening and skin thickening in mice.
Representative images of histological observation by H&E staining of mouse dorsal skin (200x)(A). Epidermal thickness (B) and average skinfold thickness (C) of different groups at the end of the experiment. Each bar represents the mean ± SD (n = 6). Means sharing the same alphabet letter on the bar are not significantly different at P < 0.05 by ANOVA and Duncan's multiple range test. Normal control group (T-NC): vehicle treatment (ethanol:polyethylene glycol=3:7, v/v), UV control group (T-UV): UV-irradiation + vehicle treatment, PET group (T-PET): UV irradiation + PPF ethanol extract, PM group (T-PM): UV irradiation + the mixture of ethylacetate fraction and butanol fraction from PPF ethanol extract. Dorsal skin was irradiated with UV 3 times a week and sample (2 mg/mouse) was topically applied 5 times/week for 9 weeks.
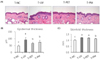
Fig. 2
Effects of topical treatment of Prunus persica Flos (PPF) extract on expression of MMP-13 and typeⅠ collagen in UV-irradiated mouse skin.
Relative mRNA expression of MMP-13 and collagen-1 in mouse dorsal skin in different groups, measured by real-time PCR (A). Protein expression of MMP-13 and typeⅠ collagen was determined by Western blot (B) and the band was quantified (C). Each bar represents the mean ± SD (n = 6). Means sharing the same alphabet letter on the bar are not significantly different at P < 0.05 by ANOVA and Duncan's multiple range test. NS: not significant. Normal control group (T-NC): vehicle treatment (ethanol:polyethylene glycol=3:7, v/v), UV control group (T-UV): UV-irradiation + vehicle treatment, PET group (T-PET): UV irradiation + PPF ethanol extract, PM group (T-PM): UV irradiation + the mixture of ethylacetate fraction and butanol fraction from PPF ethanol extract. The dorsal skin was irradiated with UV 3 times a week, and sample (2 mg/mouse) was topically applied 5 times/week for 9 weeks.
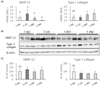
Fig. 3
Effects of topical treatment of Prunus persica Flos (PPF) extract on the activities of antioxidant enzymes in UV-irradiated mouse skin.
Catalase, Cu,Zn-superoxide dismutase (SOD) and glutathione-peroxidase (GPx) activities were measured in mouse skin. Each bar represents the mean ± SD (n = 6). Means sharing the same alphabet letter on the bar are not significantly different at P < 0.05 by ANOVA and Duncan's multiple range test. Normal control group (T-NC): vehicle treatment (ethanol:polyethylene glycol=3:7, v/v), UV control group (T-UV): UV-irradiation + vehicle treatment, PET group (T-PET): UV irradiation + PPF ethanol extract, PM group (T-PM): UV irradiation + the mixture of ethylacetate fraction and butanol fraction from PPF ethanol extract. Dorsal skin was UV irradiated 3 times a week, and sample (2 mg/mouse) was topically applied 5 times/week for 9 weeks.
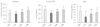
Fig. 4
Effects of oral treatment of Prunus persica Flos (PPF) extract on UV-induced epidermal and skin thickening in the mice.
Representative images of histological observation by H&E staining of mouse dorsal skin (200x)(A). Epidermal thickness (B) and average skinfold thickness (C) of different groups measured at the end of the experiment. Each bar represents the mean ± SD (n = 6). Means sharing the same alphabet letter on the bar are not significantly different at P < 0.05 by ANOVA and Duncan's multiple range test. Normal control group (O-NC): vehicle treatment (2.5% DMSO), UV control group (O-UV): UV irradiation + vehicle treatment, PET group (O-PET): UV irradiation + PPF ethanol extract treatment, PM group (O-PM): UV irradiation + treatment with the mixture of ethylacetate fraction and butanol fraction from PPF ethanol extract. UV irradiated to the mouse dorsal skin 3 times a week, and sample (100 mg/kg BW) was administered orally 5 times a week for 10 weeks.
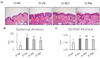
Fig. 5
Effects of oral treatment of Prunus persica Flos (PPF) extract on expression of MMP-13 and typeⅠ collagen in UV-irradiated mouse skin.
Relative mRNA expression of MMP-13 and typeⅠ collagen in mouse dorsal skin in different groups measured by real-time PCR (A). Western blot (B) and quantifications (C) of protein expression of MMP-13 and typeⅠ collagen. Each bar represents the mean ± SD (n = 6). Means sharing the same alphabet letter on the bar are not significantly different at P < 0.05 by ANOVA and Duncan's multiple range test. NS: not significant. Normal control group (O-NC): vehicle treatment (2.5% DMSO), UV control group (O-UV): UV irradiation + vehicle treatment, PET group (O-PET): UV irradiation + PPF ethanol extract treatment, PM group (O-PM): UV irradiation + treatment with the mixture of ethylacetate fraction and butanol fraction from PPF ethanol extract. Mouse dorsal skin was UV irradiated 3 times a week, and sample (100 mg/kg BW) was administered orally 5 times a week for 10 weeks.
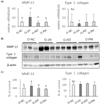
Fig. 6
Effects of oral treatment of Prunus persica Flos (PPF) extract on the activities of antioxidant enzymes and pro-inflammatory cytokine concentrations in UV-irradiated mouse skin.
Catalase, Cu,Zn-superoxide dismutase (SOD) and glutathione-peroxidase (GPx) activities (A) and IL-1β, IL-6 and TNF-α concentrations (B) were measured in mouse skin. Each bar represents the mean ± SD (n = 6). Means sharing the same alphabet letter on the bar are not significantly different at P < 0.05 by ANOVA and Duncan's multiple range test. Normal control group (O-NC): vehicle treatment (2.5% DMSO), UV control group (O-UV): UV irradiation + vehicle treatment, PET group (O-PET): UV irradiation + PPF ethanol extract treatment, PM group (O-PM): UV irradiation + treatment with the mixture of ethylacetate fraction and butanol fraction from PPF ethanol extract. Mouse dorsal skin was UV irradiated 3 times a week, and sample (100 mg/kg BW) was administered orally 5 times a week for 10 weeks.
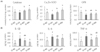
ACKNOWLEDGEMENTS
The authors thank the Ministry of Science, ICT and Future Planning, Republic of Korea.
Notes
References
1. Bowden GT. Prevention of non-melanoma skin cancer by targeting ultraviolet-B-light signaling. Nat Rev Cancer. 2004; 4:23–35.


2. Fernández-García E. Skin protection against UV light by dietary antioxidants. Food Funct. 2014; 5:1994–2003.


3. Kim M, Park YG, Lee HJ, Lim SJ, Nho CW. Youngiasides A and C isolated from Youngia denticulatum inhibit UVB-induced MMP expression and promote typeⅠ procollagen production via repression of MAPK/AP-1/NF-kB and activation of AMPK/Nrf2 in HaCat cells and human dermal fibroblasts. J Agric Food Chem. 2015; 63:5428–5438.


4. Yang HM, Ham YM, Yoon WJ, Roh SW, Jeon YJ, Oda T, Kang SM, Kang MC, Kim EA, Kim D, Kim KN. Quercitrin protects against ultraviolet B-induced cell death in vitro and in an in vivo zebrafish model. J Photochem Photobiol B. 2012; 114:126–131.


5. Zhan JY, Wang XF, Liu YH, Zhang ZB, Wang L, Chen JN, Huang S, Zeng HF, Lai XP. Andrographolide sodium bisulfate prevents UV-induced skin photoaging through inhibiting oxidative stress and inflammation. Mediators Inflamm. 2016; 3271451.


6. Kim J, Lee CW, Kim EK, Lee SJ, Park NH, Kim HS, Kim HK, Char K, Jang YP, Kim JW. Inhibition effect of Gynura procumbens extract on UVB- induced matrix-metalloproteinase expression in human dermal fibroblasts. J Ethnopharmacol. 2011; 137:427–433.


7. Chiang HM, Chen HC, Lin TJ, Shih IC, Wen KC. Michelia alba extract attenuates UVB-induced expression of matrix metalloproteinases via MAP kinase pathway in human dermal fibroblasts. Food Chem Toxicol. 2012; 50:4260–4269.


8. Hwang E, Park SY, Lee HJ, Lee TY, Sun ZW, Yi TH. Gallic acid regulates skin photoaging in UVB-exposed fibroblast and hairless mice. Phytother Res. 2014; 28:1778–1788.


9. Quan T, Qin Z, Xia W, Shao Y, Voorhees JJ, Fisher GJ. Matrix-degrading metalloproteinases in photoaging. J Investig Dermatol Symp Proc. 2009; 14:20–24.


10. Hong YF, Lee HY, Jung BJ, Jang S, Chung DK, Kim H. Lipoteichoic acid isolated from Lactobacillus plantarum down-regulates UV-induced MMP-1 expression and up-regulates typeⅠ procollagen through the inhibition of reactive oxygen species generation. Mol Immunol. 2015; 67:248–255.


12. Park JE, Pyun HB, Woo SW, Jeong JH, Hwang JK. The protective effect of Kaempferia parviflora extract on UVB-induced skin photoaging in hairless mice. Photodermatol Photoimmunol Photomed. 2014; 30:237–245.


13. Yoo HG, Lee BH, Kim W, Lee JS, Kim GH, Chun OK, Koo SI, Kim DO. Lithospermum erythrorhizon extract protects keratinocytes and fibroblasts against oxidative stress. J Med Food. 2014; 17:1189–1196.


14. Bae JS, Han M, Shin HS, Kim MK, Shin CY, Lee DH, Chung JH. Perilla frutescens leaves extract ameliorates ultraviolet radiation-induced extracellular matrix damage in human dermal fibroblasts and hairless mice skin. J Ethnopharmacol. 2017; 195:334–342.


15. Fisher GJ, Kang S, Varani J, Bata-Csorgo Z, Wan Y, Datta S, Voorhees JJ. Mechanisms of photoaging and chronological skin aging. Arch Dermatol. 2002; 138:1462–1470.


16. Lim JY, Kim OK, Lee J, Lee MJ, Kang N, Hwang JK. Protective effect of the standardized green tea seed extract on UVB-induced skin photoaging in hairless mice. Nutr Res Pract. 2014; 8(4):398–403.


17. Katiyar SK, Korman NJ, Mukhtar H, Agarwal R. Protective effects of silymarin against photocarcinogenesis in a mouse skin model. J Natl Cancer Inst. 1997; 89:556–566.


18. Bae JY, Choi JS, Kang SW, Lee YJ, Park J, Kang YH. Dietary compound ellagic acid alleviates skin wrinkle and inflammation induced by UV-B irradiation. Exp Dermatol. 2010; 19:e182–e190.


19. Divya SP, Wang X, Pratheeshkumar P, Son YO, Roy RV, Kim D, Dai J, Hitron JA, Wang L, Asha P, Shi X, Zhang Z. Blackberry extract inhibits UVB-induced oxidative damage and inflammation through MAP kinases and NF-κB signaling pathways in SKH-1 mice skin. Toxicol Appl pharmacol. 2015; 284:92–99.


20. Patwardhan J, Bhatt P. Ultraviolet-B protective effect of flavonoids from Eugenia caryophylata on human dermal fibroblast cells. Pharmacogn Mag. 2015; 11:S397–S406.
21. Lee JY, An BJ. Anti-oxidant and anti-inflammation activities of Prunus persica Flos. J Appl Biol Chem. 2010; 53:162–169.


22. Kim YH, Yang HE, Park BK, Heo MY, Jo BK, Kim HP. The extract of the flowers of Prunus persica, a new cosmetic ingredient, protects against solar ultraviolet-induced skin damage in vivo. J Cosmet Sci. 2002; 53:27–34.
23. Li C, Wang MH. Antioxidant activity of peach blossom extracts. J Korean Soc Appl Biol Chem. 2011; 54:46–53.


24. Lee JY, An BJ. Antioxidant and anti-inflammatory effects of fractions from Pruni persicae Flos. Korean J Herbol. 2012; 27:55–63.


25. Kwak CS, Choi HI. In vitro antioxidant and anti-inflammatory activities of ethanol extract and sequential fractions of floweres of Prunus persica in LPS-stimulated RAW 264.7 macrophages. J Korean Soc Food Sci Nutr. 2015; 44:1439–1449.


26. Kwak CS, Yang J. Prevention effect of prunus persica flos extract from reactive oxygen species generation and matrix metalloproteinases production induced by UVB irradiation in human skin cells. Asian J Beauty Cosmet. 2016; 14:179–190.


27. Livak KJ, Schmittgen TD. Analysis of relative gene expression data using real-time quantitative PCR and the 2(-Delta Delta C(T)) Method. Methods. 2001; 25:402–408.


28. Aebi H. Catalase in vitro. Methods Enzymol. 1984; 105:121–126.
29. Marklund S, Marklund G. Involvement of the superoxide anion radical in the autoxidation of pyrogallol and a convenient assay for superoxide dismutase. Eur J Biochem. 1974; 47:469–474.


30. Tappel AL. Glutathione peroxidase and hydroperoxides. Methods Enzymol. 1978; 52:506–513.
31. Wang XF, Huang YF, Wang L, Xu LQ, Yu XT, Liu YH, Li CL, Zhan JY, Su ZR, Chen JN, Zeng HF. Photo-protective activity of pogostone against UV-induced skin premature aging in mice. Exp Gerontol. 2016; 77:76–86.


32. Liu JC, Jiao ZG, Yang WB, Zhang CL, Liu H, Lv ZZ. Variation in phenolics, flavonoids, antioxidant and tyrosinase inhibitory activity of peach blossoms at different developmental stages. Molecules. 2015; 20:20460–20472.


33. Lee KO, Kim SN, Kim YC. Anti-wrinkle effects of water extracts of teas in hairless mouse. Toxicol Res. 2014; 30:283–289.


34. Song JH, Bae EY, Choi G, Hyun JW, Lee MY, Lee HW, Chae S. Protective effect of mango (Mangifera indica L.) against UVB-induced skin aging in hairless mice. Photodermatol Photoimmunol Photomed. 2013; 29:84–89.


35. Rhodes LE, Belgi G, Parslew R, McLoughlin L, Clough GF, Friedmann PS. Ultraviolet-B-induced erythema is mediated by nitric oxide and prostaglandin E2 in combination. J Invest Dermatol. 2001; 117:880–885.


36. Pillai S, Oresajo C, Hayward J. Ultraviolet radiation and skin aging: roles of reactive oxygen species, inflammation and protease activation, and strategies for prevention of inflammation-induced matrix degradation - a review. Int J Cosmet Sci. 2005; 27:17–34.


37. Vayalil PK, Mittal A, Hara Y, Elmets CA, Katiyar SK. Green tea polyphenols prevent ultraviolet light-induced oxidative damage and matrix metalloproteinases expression in mouse skin. J Invest Dermatol. 2004; 122:1480–1487.


38. Hassani D, Liu HL, Chen YN, Wan ZB, Zhuge Q, Li SX. Analysis of biochemical compounds and differentially expressed genes of the anthocyanin biosynthetic pathway in variegated peach flowers. Genet Mol Res. 2015; 14:13425–13436.


39. Jin UH, Lee JY, Kang SK, Kim JK, Park WH, Kim JG, Moon SK, Kim CH. A phenolic compound, 5-caffeoylquinic acid (chlorogenic acid), is a new type and strong matrix metalloproteinase-9 inhibitor: isolation and identification from methanol extract of Euonymus alatus. Life Sci. 2005; 77:2760–2769.


40. Kusano A, Seyama Y, Nagai M, Shibano M, Kusano G. Effects of fukinolic acid and cimicifugic acids from Cimicifuga species on collagenolytic activity. Biol Pharm Bull. 2001; 24:1198–1201.


41. Yin Y, Li W, Son YO, Sun L, Lu J, Kim D, Wang X, Yao H, Wang L, Pratheeshkumar P, Hitron AJ, Luo J, Gao N, Shi X, Zhang Z. Quercitrin protects skin from UVB-induced oxidative damage. Toxicol Appl Pharmacol. 2013; 269:89–99.

