Abstract
BACKGROUND/OBJECTIVES
Although Korean fermented foods contain large amounts of salt, which is known to exacerbate health problems, these foods still have beneficial effects such as anti-hypertension, anti-cancer, and anti-colitis properties. We hypothesized that ganjang may have different effects on blood pressure compared to same concentrations of salt.
MATERIALS/METHODS
Sprague-Dawley rats were divided into control (CT), NaCl (NC), and ganjang (GJ) groups and orally administered with 8% NaCl concentration for 9 weeks. The systolic blood pressure (SBP), serum chemistry, Na+ and K+ concentrations and renal gene expressions were measured.
RESULTS
The SBP was significantly increased in the NC group compared to the GJ and CT groups. In addition, the Na+ concentration in urine was higher in the GJ and NC groups than the CT group, but the urine volume was increased in the GJ group compared to the other groups. The serum renin levels were decreased in the GJ group compared to the CT group, while the serum aldosterone level was decreased in the GJ group relative to the NC group. The mRNA expression of the renin, angiotensin II type I receptor, and mineralocorticoid receptor were significantly lower in the GJ group compared to other groups. Furthermore, GJ group showed the lowest levels of genes for Na+ transporter in kidney cortex such as Na+/K+ ATPaseα1 (NKAα1), Na+/H+ exchanger 3 (NHE3), Na+/HCO3- co-exchanger (NBC), and carbonic anhydrases II (CAII).
Hypertension is the single largest cause of death and is responsible for 9.4 million of deaths in worldwide [1]. It is defined as systolic blood pressure (SBP) over 140 mmHg and/or a diastolic blood pressure (DBP) over 90 mmHg [12] . Blood pressure is regulated by the renin-angiotensin-aldosterone system (RAAS), which is involved in regulating blood volume and systemic vascular resistance. RAAS is activated by low sodium concentration and glomerular filtration pressure. RAAS plays a role in reabsorption of water, blood vessel contraction, and Na+ reabsorption via angiotensin II and aldosterone [3]. Dietary salt intake is an important factor in the development of hypertension [4]. When the plasma sodium concentration increases, intracellular fluid is osmotically transferred to the extracellular space, stimulating the thirst and resulting in an increase of extracellular fluid volume. Generally, when thirst is stimulated, water consumption increases, resulting in increased total body fluid and blood pressure [56]. Consequently, the WHO recommends a salt intake less than 5 g per day. Therefore, the Korea Food & Drug Administration (KDA) not only reduced the daily recommended salt intake, but also the daily allowance of Korean fermented food containing a large amount of salt (kimchi 1 serving size: 40 g (Na+: 312.08 mg)). Ganjang (traditional Korean soy sauce) is widely used in sauces as a seasoning in Korea, and salt-free or low-salt ganjang was reported to have anti-colitis [7], anti-cancer [8], and anti-hypertension [910] properties. A recent ganjang study used the peptide from the stem of salt-free/low-salt ganjang. To our knowledge, a study using high-salt ganjang has not been reported.
Therefore, the biological effects of ganjang as the regulation system of blood pressure were investigated in an animal model.
Ganjang was manufactured by the Sunchang Sauce Corporation (Sunchang-gun, Jeollabuk-do, Korea) as fig. 1. Ganjang was matured for 6 months. The salinity of ganjang and salt water (NaCl; Samchun, Pyeongtaek-si, Gyeonggi-do, Korea) was adjusted to 8% [1112] by Mohr's method [13]. The amount of sodium and potassium ingested by the animal was shown in Table 2. The control group orally administrated the same amount of distilled water (DW) as control.
Five weeks male Sprague-Dawley rats (Central laboratory animal Co., Seoul, Korea) were housed in semi-SPF facilities with temperature (25 ± 2℃) and humidity controlled under 12-hr light/dark cycle conditions. Rats were randomly divided into 3 groups according to similar body weight and SBP; Control (CT), NaCl (NC), and Ganjang (GJ) after 1 week of adaptation (n = 8). The diet was given to rodent diet (Research Diets, Inc. New Brunswick, NJ, USA) and animals were orally administrated (10 mL/kg body weigh/day) for 9 weeks. SBP was measured every week in conscious rats using tail-cuff method (BP-2000, Visitech Systems, Inc., Apex, NC, USA) after 6 hours of oral administration. In last 4 weeks, rats were housed in metabolic cages for 24 hours per week, and dietary intake and water intake were measured. Furthermore, urine and feces were collected for analysis. The rats were anesthetized at the end of 9 wk experimental period. Blood was collected and centrifuged at 4,000 rpm for 15 min at 4℃, and organ tissues were harvested. The serum and all tissues were stored at -70℃ until use. All animal protocols were approved by the Institutional Animal Care and Use Committees of Chonbuk National University (CBNU 2016-0030).
Serum rennin, angiotensin II, and aldosterone concentrations were analyzed using an ELISA kit (Rat Renin ELISA Kit, MyBioSource, San Diego, CA, USA; Angiotensin II ELISA Kit, and Aldosterone ELISA Kit, Enzo Life Sciences, Inc., Farmingdale, NY, USA).
RNA was extracted from tissues using the Trizol method. The RNA synthesized to cDNA using high-capacity cDNA Reverse Transcription Kit (Applied Biosystem, Vilnius, Lithuania) with reverse transcription PCR (MWG Biotech Inc., Ebersberg, Germany). The cDNA analyzed as relative gene (Table 1) expression using SYBR Green (TOYOBO, Osaka, Japan) and Real-Time PCR (Applied Biosystems, Foster City, CA, USA).
Na+, K+, and Ca2+ concentrations in urine and feces were measured using inductively coupled plasma-mass spectroscopy (ICP-MS; 7500A, Agilent Technologies, Germantown, MD, USA). Samples were analyzed in the Center for University-Wide Research Facilities (CURF) at Chonbuk National University.
SBP in the NC group was higher than in the CT and GJ groups after oral administration of the diets (Fig. 2). There was a trend of decrease in SBP of GJ compared to other groups. SBP in the NC group increased 2 mmHg from 151.9 ± 13.65 mmHg to 153.47 ± 10.91 mmHg, while those in the CT and GJ groups decreased 10 mmHg from 150.87 ± 19.10 mmHg to 140.57 ± 11.10 mmHg and 19 mmHg from 155.3 ± 15.90 mmHg to 136.87 ± 11.57 mmHg, respectively Thus, on average, the SBP in the NC group was 13 mmHg higher than in the CT group and 14 mmHg in the GJ group during oral administration.
Urine volume results from the 24 hour metabolic cage test were increased in GJ group compared to CT and NC, but body weight, food intake and water intake were not significantly different among groups (Table 2). However, ion contents in urine and feces were significantly different. In urine, Na+ was higher in the NC (6,828.67 ± 1,671.46 ppm) and GJ (6,206.0 ± 777.02 ppm) groups than in the CT group (1,060.83 ± 370.65 ppm); however, the results were not significantly different between the NC and GJ groups. The levels of K+ and Ca2+ ions were not significantly different among the 3 groups. Conversely, in feces, Na+ was not significantly different, but the levels of K+ and Ca2+ ions were decreased the GJ group than other groups (Table 3). Serum Na+ was higher in the NC group (137.00 ± 0.00 mEq/L) than in the GJ group (136.33 ± 1.37 mEq/L) and CT group (135.50 ± 0.84 mEq/L). In addition, K+ was highest in the GJ group (4.83 ± 0.74 mEq/L), followed by the CT group (4.40 ± 0.37 mEq/L) and the NC group (4.08 ± 0.12 mEq/L) (Table 4).
Serum renin level was highest in the CT group (29.58 ± 9.91 pg/mL), followed by the NC group (25.2 ± 5.58 pg/mL) and GJ group (17.23 ± 2.50 pg/mL). Aldosterone level was highest in the NC group (877.73 ± 274.76 pg/mL), followed by the CT group (696.38 ± 134.52 pg/mL) and GJ group (546.15 ± 251.59 pg/mL). The serum rennin and aldosterone levels were lowest in the GJ group. However, serum angiotensin II level was not significantly different among the groups (Fig. 3).
Renin mRNA expression was relatively high in the CT group (1.03 ± 0.32), followed by the NC group (0.60 ± 0.30) and the GJ group (0.34 ± 0.08). Mineralocorticoid receptor (MR) expression was significantly lower in the GJ group compared with the other groups (CT, 1.00 ± 0.13; NC, 0.70 ± 0.16; GJ, 0.43 ± 0.07). Similarly, angiotensin II type 1 receptor (AT1 receptor) gene expression was higher in the CT group (1.02 ± 0.25) than in the NC group (0.65 ± 0.04) and GJ group (0.48 ± 0.07); expression in the NC and GJ groups was not significantly different. However, angiotensin I converting enzyme (ACE) gene expression was not significantly different among the groups (Fig. 4).
Na+ transporter gene expression showed similar results in urine and feces based on ion analysis. The GJ group was lower in Na+/K+ ATPaseα1 (NKAα1), Na+/H+ exchanger 3 (NHE3), Na+/HCO3- (bicarbonate) co-exchanger (NBC) and carbonic anhydrases II (CAII) than the NC group. The CT group also showed lower expression than the NC group in most of the sodium transporters except NKAα1. However, Na-Ca exchanger (NCX) gene expression was not significantly different among the groups (Fig. 5).
Dietary sodium can increase blood Na+ concentration and extracellular fluid volume. Therefore, sodium intake is closely associated with blood pressure [5614]. Additionally, a long-term high-salt diet induced renal injury and alteration of the renal cytokine genes followed by hypertension in normal rats [15]. In present study, blood pressure was significantly different in ganjang and sodium water despite the same concentration of NaCl in the groups. Therefore, ganjang may affect the RAAS differently compared to salt water and have a weaker negative effect on kidneys. High consumption of salt-fermented vegetables (kimchi) has not shown to be associated with increased risk of hypertension in adults [16]. Fermented rice bran decreased SBP in stroke-prone spontaneously hypertensive rats [17]. The metabolic syndrome related hypertension was improved by fermented red ginseng [18]. Microorganisms, dietary fiber, and phenolic compounds can be effective in the prevention of lifestyle-related diseases. The fermented milk and its peptide by lactic acid bacteria had hypotensive effect by inhibiting ACE activity, hydrolysis peptides, and vasodilator [1920].
In present study, we investigated whether the same concentrations of salts in ganjang and salt itself may have different effects in hypertension-induced rats. As shown in Table 2, the amounts of sodium and potassium intake were different in NC and GJ groups supplemented with same concentration salts. Interestingly, NC had 92 mg/kg BW/day more sodium intake than GJ group. Reduced intake of sodium and increased intake of potassium can be important factors in preventing hypertension [21]. Therefore, the reduced sodium contents in ganjang compared to table salt may be responsible for the decreased blood pressure in GJ group.
Based on the results of ion contents in urine and feces, Na+ ion excretion was lower in CT group urine, and K+ and Ca2+ ion excretion were significantly higher in CT group feces. K+ and Ca2+ ions are exchanged ions during Na+ reabsorption, indicating high Na+ reabsorption activity in the CT group. Na+ ion excretion in feces was not significantly different between the GJ and NC groups, although K+ and Ca2+ ion excretion were significantly higher in NC group feces, indicating higher Na+ reabsorption activity in the NC group compared with the GJ group. K+ and Ca2+ were exchanged Na+ by NKAα1 and NCX that was controlled by RAAS. NKAα1 has the central role in sodium reabsorption [22] and Ca2+ entry mode of NCX is triggered by the salt-dependent hypertension [23]. Additionally, it was reported to urinary sodium excretion is significantly associated with blood pressure [24]. The sodium excretion in the hypertensive people was higher than normal people [25]. Although serum Na+ was increased in GJ group than CT group, but urinary volume significantly increased the GJ group compared to CT group. The lower RAAS activity and increased excretion of Na+ in GJ group can improve the blood pressure.
With regard to serum hormone concentrations, renin level was lower in the GJ group than in the NC group, although SBP was lower in the CT group. This result is considered because renin is activated when plasma sodium level is low and blood flow is decreased [26]. Renin was activated and catalyzed angiotensinogen into angiotensin I when the prorenin form bound to the kidney renin receptor. Additionally, the RAAS was instigated by rennin binding with rennin receptor [27]. A high concentration of renin was not correlated with high enzymatic activity. However, similar tendencies of renal rennin mRNA expression, rennin secretion, and plasma rennin activity [28]. The renin mRNA abundance was tended to be suppressed by the high-NaCl diet [29]. Renin and renal renin mRNA levels were lower in the NC and GJ groups than the CT group, although not significantly. We hypothesized that a significant difference occurred when catalyzing from a high angiotensinogen level to angiotensin I and high angiotensin II level. However, ACE mRNA and angiotensin II levels were not significantly different. The ACE mRNA and ACE activity showed similar tendencies [3]. However, the aldosterone level was not significantly higher in the NC group than in the CT group. In contrast, angiotensin receptor type 1 (receptor of angiotensin II) was significantly higher in the CT group than in the NC and GJ groups. Angiotensin II activates AT receptors; AT1 receptors mainly mediate the stimulation of aldosterone secretion and angiotensin II, but type 2 receptors (AT2 receptors) also activate stimulation of aldosterone secretion by local release of catecholamines [30]. The activated AT2 receptor was considered a potential variable for aldosterone secretion. The aldosterone increased the expression of MR and AT1 receptors, thus affecting not only angiotensin II, but also aldosterone levels [31].
Aldosterone regulates the transfer of Na+ and K+ in distal nephrons and stimulates expression of transporters by binding to MR, a ligand-dependent transcription factor [32]. The mRNA expression of MR was higher in the CT group than in the NC group, and that of the NC group was higher than that of the GJ group. These results indicate that MR is affected by aldosterone and AT1 receptor expression [33]. The gene expression of MR and AT1 receptors was similar to their protein expression [3435]. Angiotensin II and aldosterone stimulate Na+ reabsorption transporter expression through AT1 receptor and MR [323637].
Na+ reabsorption transporters were directly/indirectly regulated by AT1 receptor and MR. NKAα1 was significantly higher in the CT group than in the NC group and was significantly lower in the GJ group than in the NC group. Similar results were observed with the AT1 receptor and MR gene expression. NBC and NHE3 were also regulated by the AT1 receptor and interacted with CAII. These results show similar tendencies with CAII gene expression. NCX was not significantly different among the groups, but the results showed a similar tendency to the Ca2+ excretion in feces. To conclude, under prolonged high sodium intake, renin, aldosterone, AT1 receptor, and MR were similarly and/or more activated compared to conditions of normal sodium intake. The Na+ transporters increased, enhancing Na+ reabsorption and causing hypertension. However, in the case of ganjang, when intake with same sodium concentration compare to high sodium intake group (NC group), renin, aldosterone, and MR were significantly lower than in the NC group. The Na+ transporters in the GJ decreased, which decreased K+ and Ca2+ excretion in feces, resulting in decreased Na+ reabsorption compared with the NC group. Further study is warranted to investigate the bioactive component which is responsible for antihypertension effects of ganjang and its underlying mechanisms. Our results suggest that consumption of ganjang may have lower risk of hypertension than table salt (Fig. 6).
Figures and Tables
Fig. 2
Systolic blood pressure for experimental period. Points are mean ± SD (n = 8). Values with different letters indicate statistical difference (P < 0.05) by Duncan's multiple range test (a > b). CT, Control; NC, NaCl group; GJ, ganjang group.
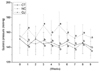
Fig. 3
Renin, angiotensin II, and aldosterone level in serum.
Values are shown as the means ± SD. Values with different letters within a column are significantly different from each other at P < 0.05 by Duncan's multiple range test (a > b). CT, Control; NC, NaCl group; GJ, ganjang group.
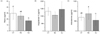
Fig. 4
RASS relative gene expression in kidney cortex.
Values are shown as the means ± SD. Values with different letters within a column are significantly different from each other at P < 0.05 by Duncan's multiple range test (a > b > c). CT, Control; NC, NaCl group; GJ, ganjang group. ACE, angiotensin converting enzyme; AT1 receptor, angiotensin II type 1 receptor; MR, mineralocorticoid receptor.
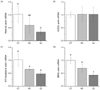
Fig. 5
Sodiumtransporter gene expression in kidney cortex.
Values are shown as the means ± SD. Values with different letters within a column are significantly different from each other at P < 0.05 by Duncan's multiple range test (a > b > c). CT, Control; NC, NaCl group; GJ, ganjang group. NKAα1, Na+/K+ ATPaseα1; NBC, Na+/HCO3- co-exchanger; CAII, carbonic anhydrases II; NHE3, Na+/H+ exchanger 3; NCX, Na+/Ca2+ exchanger.
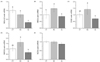
Fig. 6
Diagram of the effect of ganjang compared to table salt.
When compared to table salt intake, renin and aldosterone secretion was decreased with ganjang. MR, NKAα1, NBC, NHE3, and CAII mRNA were also decreased, indicating a decrease of Na+ reabsorption. Therefore, the results from this study showed that the probability of hypertension is lower in a diet including ganjang compared to a high-salt diet, evident by down regulation of sodium transporters in the GJ group compared to the NC group. CT, Control; NC, NaCl group; GJ, ganjang group; ACE, angiotensin converting enzyme; AT1 receptor, angiotensin II type 1 receptor; MR, mineralcorticoid receptor; NKAα1, Na+/K+ ATPaseα1; NBC, Na+/HCO3- co-exchanger; NHE3, Na+/H+ exchanger 3; CAII, carbonic anhydrases II; NCX, Na+/Ca2+ exchanger.
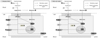
Table 2
Body weight, daily intake, and urine volume

1) These are amount of additional sodium and potassium ingested by oral administration.
2) CT, Control; NC, NaCl group; GJ, ganjang group
3) Values are shown as the means ± SD (n = 8). Values with different letters within a column are significantly different from each other at P < 0.05 by Duncan's multiple range test (a > b). NS, Not significant.
References
2. Chobanian AV, Bakris GL, Black HR, Cushman WC, Green LA, Izzo JL Jr, Jones DW, Materson BJ, Oparil S, Wright JT Jr, Roccella EJ. Joint National Committee on Prevention, Detection, Evaluation, and Treatment of High Blood Pressure. National Heart, Lung, and Blood Institute. National High Blood Pressure Education Program Coordinating Committee. Seventh report of the joint national committee on prevention, detection, evaluation, and treatment of high blood pressure. Hypertension. 2003; 42:1206–1252.


3. Schunkert H, Ingelfinger JR, Hirsch AT, Pinto Y, Remme WJ, Jacob H, Dzau VJ. Feedback regulation of angiotensin converting enzyme activity and mRNA levels by angiotensin II. Circ Res. 1993; 72:312–318.


4. Trepiccione F, Zacchia M, Capasso G. The role of the kidney in salt-sensitive hypertension. Clin Exp Nephrol. 2012; 16:68–72.


5. de Wardener HE, He FJ, MacGregor GA. Plasma sodium and hypertension. Kidney Int. 2004; 66:2454–2466.


6. Meneton P, Jeunemaitre X, de Wardener HE, MacGregor GA. Links between dietary salt intake, renal salt handling, blood pressure, and cardiovascular diseases. Physiol Rev. 2005; 85:679–715.


7. Song JL, Choi JH, Seo JH, Lim YI, Park KY. Anti-colitic effects of kanjangs (fermented soy sauce and sesame sauce) in dextran sulfate sodium-induced colitis in mice. J Med Food. 2014; 17:1027–1035.


8. Lee HJ, Lee KW, Kim KH, Kim HK, Lee HJ. Antitumor activity of peptide fraction from traditional Korean soy sauce. J Microbiol Biotechnol. 2004; 14:628–630.
9. Matsui T, Zhu XL, Shiraishi K, Ueki T, Noda Y, Matsumoto K. Antihypertensive effect of salt-free soy sauce, a new fermented seasoning, in spontaneously hypertensive rats. J Food Sci. 2010; 75:H129–H134.


10. Nakahara T, Sugimoto K, Sano A, Yamaguchi H, Katayama H, Uchida R. Antihypertensive mechanism of a peptide-enriched soy sauce-like seasoning: the active constituents and its suppressive effect on renin-angiotensin-aldosterone system. J Food Sci. 2011; 76:H201–H206.


11. Maia DR, Lopes KL, mann JC, Furukawa LN. High maternal sodium intake alters sex-specific renal renin-angiotensin system components in newborn Wistar offspring. J Dev Orig Health Dis. Forthcoming 2016.


12. Lei GT, Wu QH. Effects of oxidative stress and gender differences in SD rats with high-salt hypertension via acute short-term cold exposure. Heart. 2010; 96:A40.
13. Korkmaz D. Precipitation titration: “Determination of Chloride by the Mohr Method”. Methods. 2001; 2:1–6.
14. Rhee MY, Kim JH, Na SH, Chung JW, Bae JH, Nah DY, Gu N, Kim HY. Elevation of heart-femoral pulse wave velocity by short-term low sodium diet followed by high sodium diet in hypertensive patients with sodium sensitivity. Nutr Res Pract. 2016; 10:288–293.


15. Gu JW, Young E, Pan ZJ, Tucker KB, Shparago M, Huang M, Bailey AP. Long-term high salt diet causes hypertension and alters renal cytokine gene expression profiles in Sprague-Dawley rats. Beijing Da Xue Xue Bao. 2009; 41:505–515.
16. Song HJ, Park SJ, Jang DJ, Kwon DY, Lee HJ. High consumption of salt-fermented vegetables and hypertension risk in adults: a 12-year follow-up study. Asia Pac J Clin Nutr. 2017; 26:698–707.
17. Alauddin M, Shirakawa H, Koseki T, Kijima N, Ardiansyah , Budijanto S, Islam J, Goto T, Komai M. Fermented rice bran supplementation mitigates metabolic syndrome in stroke-prone spontaneously hypertensive rats. BMC Complement Altern Med. 2016; 16:442.


18. Kho MC, Lee YJ, Park JH, Kim HY, Yoon JJ, Ahn YM, Tan R, Park MC, Cha JD, Choi KM, Kang DG, Lee HS. Fermented red ginseng potentiates improvement of metabolic dysfunction in metabolic syndrome rat models. Nutrients. 2016; 8:E369.


19. Yahya MA, Alhaj OA, Al-Khalifah AS. Antihypertensive effect of fermented skim camel (Camelus dromedarius) milk on spontaneously hypertensive rats. Nutr Hosp. 2017; 34:416–421.


20. Beltrán-Barrientos LM, Hernández-Mendoza A, Torres-Llanez MJ, González-Córdova AF, Vallejo-Córdoba B. Invited review: fermented milk as antihypertensive functional food. J Dairy Sci. 2016; 99:4099–4110.


21. Geleijnse JM, Kok FJ, Grobbee DE. Blood pressure response to changes in sodium and potassium intake: a metaregression analysis of randomised trials. J Hum Hypertens. 2003; 17:471–480.


22. Féraille E, Doucet A. Sodium-potassium-adenosinetriphosphatase-dependent sodium transport in the kidney: hormonal control. Physiol Rev. 2001; 81:345–418.


23. Iwamoto T, Kita S, Zhang J, Blaustein MP, Arai Y, Yoshida S, Wakimoto K, Komuro I, Katsuragi T. Salt-sensitive hypertension is triggered by Ca2+ entry via Na+/Ca2+ exchanger type-1 in vascular smooth muscle. Nat Med. 2004; 10:1193–1199.


24. Iwamoto T. Vascular Na+/Ca2+ exchanger: implications for the pathogenesis and therapy of salt-dependent hypertension. Am J Physiol Regul Integr Comp Physiol. 2006; 290:R536–R545.
25. Huang F, Yu P, Yuan Y, Li Q, Lin F, Gao Z, Chen F, Zhu P. The relationship between sodium excretion and blood pressure, urine albumin, central retinal arteriolar equivalent. BMC Cardiovasc Disord. 2016; 16:194.


26. Shao W, Seth DM, Prieto MC, Kobori H, Navar LG. Activation of the renin-angiotensin system by a low-salt diet does not augment intratubular angiotensinogen and angiotensin II in rats. Am J Physiol Renal Physiol. 2013; 304:F505–F514.


27. Ichihara A, Kaneshiro Y, Takemitsu T, Sakoda M, Itoh H. The (pro) renin receptor and the kidney. Semin Nephrol. 2007; 27:524–528.
28. Schricker K, Holmer S, Hamann M, Riegger G, Kurtz A. Interrelation between renin mRNA levels, renin secretion, and blood pressure in two-kidney, one clip rats. Hypertension. 1994; 24:157–162.


29. Tank JE, Henrich WL, Moe OW. Regulation of glomerular and proximal tubule renin mRNA by chronic changes in dietary NaCl. Am J Physiol. 1997; 273:F892–F898.
30. Mazzocchi G, Gottardo G, Macchi V, Malendowicz LK, Nussdorfer GG. The AT2 receptor-mediated stimulation of adrenal catecholamine release may potentiate the AT1 receptor-mediated aldosterone secretagogue action of angiotensin-II in rats. Endocr Res. 1998; 24:17–28.


31. Tsai CF, Yang SF, Chu HJ, Ueng KC. Cross-talk between mineralocorticoid receptor/angiotensin II type 1 receptor and mitogen-activated protein kinase pathways underlies aldosterone-induced atrial fibrotic responses in HL-1 cardiomyocytes. Int J Cardiol. 2013; 169:17–28.


32. Thomas W, McEneaney V, Harvey BJ. Aldosterone-induced signalling and cation transport in the distal nephron. Steroids. 2008; 73:979–984.


33. Jaffe IZ, Mendelsohn ME. Angiotensin II and aldosterone regulate gene transcription via functional mineralocortocoid receptors in human coronary artery smooth muscle cells. Circ Res. 2005; 96:643–650.


34. Yang BC, Phillips MI, Mohuczy D, Meng H, Shen L, Mehta P, Mehta JL. Increased angiotensin II type 1 receptor expression in hypercholesterolemic atherosclerosis in rabbits. Arterioscler Thromb Vasc Biol. 1998; 18:1433–1439.


35. Han F, Ding J, Shi Y. Expression of amygdala mineralocorticoid receptor and glucocorticoid receptor in the single-prolonged stress rats. BMC Neurosci. 2014; 15:77.

