Abstract
BACKGROUND/OBJECTIVES
Re-epithelialization has an important role in skin wound healing. Astaxanthin (ASX), a carotenoid found in crustaceans including shrimp, crab, and salmon, has been widely used for skin protection. Therefore, we investigated the effects of ASX on proliferation and migration of human skin keratinocyte cells and explored the mechanism associated with that migration.
MATERIAL/METHOD
HaCaT keratinocyte cells were exposed to 0.25-1 µg/mL of ASX. Proliferation of keratinocytes was analyzed by using MTT assays and flow cytometry. Keratinocyte migration was determined by using a scratch wound-healing assay. A mechanism for regulation of migration was explored via immunocytochemistry and western blot analysis.
RESULTS
Our results suggest that ASX produces no significant toxicity in human keratinocyte cells. Cell-cycle analysis on ASX-treated keratinocytes demonstrated a significant increase in keratinocyte cell proliferation at the S phase. In addition, ASX increased keratinocyte motility across the wound space in a time-dependent manner. The mechanism by which ASX increased keratinocyte migration was associated with induction of filopodia and formation of lamellipodia, as well as with increased Cdc42 and Rac1 activation and decreased RhoA activation.
CONCLUSIONS
ASX stimulates the migration of keratinocytes through Cdc42, Rac1 activation and RhoA inhibition. ASX has a positive role in the re-epithelialization of wounds. Our results may encourage further in vivo and clinical study into the development of ASX as a potential agent for wound repair.
Cutaneous wound healing is a physiological process involved in inflammation, cell proliferation, and tissue remodeling. The wound repair process requires several types of cells, including endothelial cells, immune cells, fibroblasts, and keratinocytes [1]. Cellular disturbance in any of the wound healing steps results in postponement of tissue repair [2]. During the formation of new tissue, keratinocyte proliferation and migration have important roles in effective re-epithelialization and wound healing [3]. Furthermore, RhoA, Rac, and Cdc42, members of the small monomeric GTPase Rho family, are associated with several biological events and participate in the progression of the cell cycle, as well as in cell growth and differentiation, reorganization of the cytoskeleton, and cell motility [4]. During cellular changes in response to cell migration, activation of Rho protein has been related to focal adhesion formation and actin stress fiber complexes [56]. Rac and Cdc42 have been reported to be associated with the formation of lamellipodia and filopodia [78]. Cdc42, Rac, and RhoA are crucial for the regulation of cell migration and are reported to organize adhesion complex assembly and disassembly [9].
Astaxanthin (3,3′-dihydroxy-β,β′-carotene-4,4′-dione; ASX), is a non-provitamin-A carotenoid classified as a xanthophyll and is commonly found in microalgae, red yeast, salmon, shrimp, lobsters, and crayfish [10]. ASX has several pharmacological effects, including antioxidant [1112], and anti-inflammatory [131415] effects. Moreover, ASX was reported to induce a strong protective action against skin injury [1617] and for wound healing [1819]. Previous studies have shown that ASX induces proliferation and migration in vascular endothelial and smooth muscle cells via activation of the Wnt/β-catenin signaling pathway [20]. Rho GTPase has a major role in noncanonical Wnt signaling pathway involving changes in polarized cell shape and migration [21]. However, the effects of ASX from Litopenaeus vannamei extracts on keratinocytes proliferation and migration, important processes in re-epithelialization and wound healing, have not been elucidated. In the present study, we investigated whether ASX can induce proliferation and migration of human keratinocytes. Moreover, the mechanism associated with the ASX-induced increase in cell migration was determined.
Dulbecco's modified Eagle's medium (DMEM), fetal bovine serum (FBS), L-glutamine, and penicillin/streptomycin were purchased from Gibco BRL Life Technologies (Grand Island, NY, USA). The 3-(4,5-dimethylthiazol-2-yl)-2,5-diphenyltetrazolium bromide (MTT), phalloidin tetramethylrhodamine B isothiocyanate, bovine serum albumin (BSA) and dimethylsulfoxide (DMSO) were obtained from Sigma (St. Louis, MO, USA). Primary antibodies specific to Cdc42, Rac1, and RhoA were obtained from Abcam (Cambridge, UK), while the primary antibodies against β-actin, the secondary antibody goat anti-rabbit IgG/HRP, and propidium iodide (PI) were purchased from Cell Signaling Technology (Danvers, MA, USA). All other reagents used in the experiments were high-grade, commercially available products. ASX was isolated from L. vannamei as described previously [1522]. The extraction yield was 20.39 ± 1.02 mg of ASX per gram of shrimp shell, and identification of ASX was previously reported [152223]. ASX was dissolved in DMSO and applied to cultures at final concentrations of 0.25-1 µg/mL. The final concentration of DMSO in the culture medium was always less than 0.1% (v/v), which was non-toxic to the cell cultures.
The human keratinocyte cell line HaCaT was obtained from the Cell Line Service, Heidelberg, Germany. The cells were maintained at 37℃ in a 5% CO2 atmosphere with 95% humidification in complete DMEM medium containing 10% heat-inactivated FBS, 2 mM L-glutamine, 100 U/mL penicillin and 100 µg/mL streptomycin.
HaCaT cells were grown in 96-well plates at a density of 1 × 104 cells/well in complete DMEM medium. Cells were treated with ASX at concentrations of 0, 10, 100, 250, 500, 750, or 1,000 µg/mL for 24, 48, and 72 h. Cell viability was determined by using a colorimetric MTT assay. Briefly, MTT solution was added to the cell culture at a final concentration of 0.5 mg/mL. After 2 h of incubation, the insoluble formazan was dissolved in the DMSO and the obtained optical density was measured at 570 nm by using a microplate reader (Bio-Tek, Winooski, VT, USA).
HaCaT cells were seeded at 1 × 104 cells/well in 96-well plates in the complete medium. After 24 h of incubation, cells were incubated with ASX at concentrations of 0, 0.125, 0.25, 0.5, 1, 5, or 10 µg/mL for 24, 48, and 72 h at 37℃. The stimulated cells were then exposed to 0.5 mg/mL MTT for 2 h at 37℃. Cell proliferation was determined by using a colorimetric MTT assay.
For cell-cycle analysis, cells were incubated for 72 h in the presence or absence of ASX. Subsequently, the cells were collected, washed with PBS, and fixed in 70% ethanol overnight at 4℃. Thereafter, cells were washed and incubated with PI (50 µg/mL) and RNase (200 µg/mL) at room temperature for 30 min. DNA fluorescence was analyzed by using a flow cytometer (BD Biosciences, San Jose, Ca, USA).
Migration of human skin keratinocyte cells was determined by using a scratch wound-healing assay. Cells (1.5 × 105 cells/well) were grown with complete DMEM containing 0.1% FBS to a 100% confluent monolayer in 24-well plates, and a wound space was made by using a sterile P200 micropipette tip. The wells were then washed with PBS and incubated with ASX at concentrations of 0.25, 0.5, or 1 µg/mL for 0, 9, 24, 48, and 72 h. The wound spaces were examined at 20 × magnification by using a phase-contrast microscope (Olympus IX70, Japan) and wound spaces were assessed from three fields of view. The wound closure area was calculated by using ImageJ 1.41 software (NIH, Bethesda, MD, USA). Quantitative analysis of cell migration was performed using an average wound area from three fields of view, and the percentage of wound area was determined using the following formula: % wound area = (average area at 9, 24, 48 or 72 h) / (average area at 0 h) × 100
HaCaT cells were grown at 5 × 104 cells/well in 6-well plates in the complete medium. After 24 h of incubation, cells were incubated with ASX at concentrations of 0, 0.25, 0.5 or 1 µg/mL for 9 h. Filopodia and lamellipodia formation was determined by using a phalloidin-rhodamine staining assay. After ASX treatment, the cells were fixed with 4% paraformaldehyde in PBS for 10 min and permeabilized with 0.1% Triton-X100 in PBS for 5 min. Thereafter, the cells were washed with PBS, blocked with 0.2% BSA for 30 min, and incubated with 10 µg/mL of phalloidin-rhodamine in PBS for 30 min. Cell morphology was visualized by using a fluorescence microscope (Olympus IX70 with DP50, Shinjuku-ku, Tokyo, Japan).
HaCaT cells (2 × 106 cells/well) were treated with ASX at concentrations of 0.25, 0.5 or 1 µg/mL for 9 h. After ASX treatment, keratinocyte cells were prepared in RIPA buffer [150 mM NaCl, 50 mM Tris-HCl (pH 7.5), 5 mM EDTA-4Na (pH 8.0), 1% Nonidet P-40, 0.1% sodiumdodecylsulfate (SDS), 0.5% sodium deoxycholate, and protease inhibitor cocktail (Sigma)] and incubated at 4℃ for 30 min. The lysate suspension was separated by centrifugation at 14,000 × g for 10 min at 4℃, and the protein concentration in each sample was determined by using the Bradford protein assay (Bio-Rad, Hercules, CA, USA). Fifty micrograms of protein were denatured for 5 min at 95℃ and subjected to 10% SDS-polyacrylamide gel electrophoresis. After separation, the proteins were transferred to nitrocellulose membranes, which were then blocked by using 5% non-fat dry milk in Tris-buffered saline containing 0.1% Tween 20 and incubated with primary antibody at room temperature for 2 h. The membranes were then washed and later incubated with an appropriate HRP-conjugated secondary antibody for 1 h at room temperature. Specific bands were visualized by chemiluminescence (Thermo Scientific, Waltham, MA, USA) and quantified densitometrically by using ImageJ 1.41 software.
To examine the cytotoxicity of ASX, HaCaT cells were incubated with ASX at concentrations of 0, 10, 100, 250, 500, 750, or 1,000 µg/mL for 24, 48, and 72 h. The results showed that treatment with ASX significantly decreased keratinocyte cell viability in a concentration-dependent manner compared to that in the untreated (0 µg/mL) group (Fig. 1). Significantly toxic ASX doses were in the range of 250-1,000 µg/mL, and the half maximal inhibitory concentration (IC50) in HaCaT cells were 942.44 ± 48.45, 659.46 ± 88.88, and 158.59 ± 43.54 µg/mL at 24, 48, and 72 h, respectively.
To investigate the effect of ASX on the proliferative activity of HaCaT cells we used MTT assays. Low concentrations (0.25-10 µg/mL) of ASX significantly increased human skin keratinocyte cell proliferation at 72 h, compared to that in the untreated control cells (Fig. 2A). However, ASX did not significantly affect keratinocyte proliferation at 24 or 48 h. The 0.25, 0.5, and 1 µg/mL ASX doses were the most effective in terms of the promotion of cell proliferation; thus, that concentration range was used for all subsequent experiments.
Fig. 2B and 2C showed the effect of ASX on HaCaT cell-cycle phases after 72 h of treatment. Flow cytometry analysis showed that the 0.25-1 µg/mL concentrations of ASX induced a significant increase in cells in the S phase, while there was a decrease in the G1 phase only in the 1 µg/mL ASX treatment. However, ASX did not induce proliferation of HaCaT cells at 24 and 48 h of treatment (data not shown) corresponding to the proliferative result shown in Fig. 2A. The results suggest that ASX increased the proliferation of keratinocyte cells.
Increased keratinocyte migration is related to a high degree of re-epithelialization and wound closure, thus we examined whether ASX has a beneficial role on the wound healing process. The cell migration of HaCaT was evaluated by a scratch wound-healing assay after treatment with ASX (0.25-1 µg/mL) for 0, 9, 24, 48, and 72 h. Interestingly, the area of the original wound significantly decreased following treatment with ASX at 0.25, 0.5 and 1 µg/mL in both dose- and time-dependent manners. Complete wound closure was observed at 72 h of ASX treatment, whereas, at 72 h, the original wound gap was not completely closed in the untreated control HaCaT monolayer (Fig. 3A and 3B). The results indicate that ASX enhances the migration of keratinocyte cells.
Cell movement involves the generation of cell protrusions formation such as filopodia and lamellipodia. The effect of ASX on the formation of filopodia and lamellipodia in human skin keratinocyte was determined by using a phalloidin-rhodamine staining assay. The cells were treated with different concentrations of ASX (0.25-1 µg/mL) for 9 h. As shown in Fig. 4A, ASX treatment enhanced filopodia and lamellipodia formation in human skin keratinocyte cells. As ASX was also shown to promote keratinocyte migration across the wound space (Fig. 3), we sought to determine the underlying mechanism. Time-course analysis (1, 3, 6, 9, 12, and 24 h) of cells treated with ASX revealed that ASX increased the expressions of Rac1 and RhoA with the highest expressions observed at 9 h (data not shown). Subsequently, the cells were cultured in the presence or absence of 0.25-1 µg/mL of ASX for 9 h. The expression levels of migration-related proteins, including Cdc42, Rac1, and RhoA, were examined by using western blotting. As shown in Fig. 4B and 4C, ASX significantly increased the expression levels of Cdc42 and Rac1 but decreased RhoA expression. These results indicate that ASX enhances the formation of cellular filopodia and lamellipodia via activation of Cdc42 and Rac1.
Descriptions of the pharmaceutical activities of components of marine organisms have been reported in recent decades. Beneficial effects of marine carotenoids have been shown, especially with regard to astaxanthin, zeaxanthin, and fucoxanthin, which are the most abundant marine carotenoids [24], and such carotenoids have been shown to possess strong antioxidant activity [1112] and skin protection ability [1617]. Epithelial cell proliferation and migration are major events in the formation of epithelium. During wound re-epithelialization and healing, keratinocytes move toward the wound surface and fill the wound space [1325]. Proliferation and migration of keratinocytes are essential for tissue epithelization [26]. In the present study, we demonstrated that ASX promotes keratinocyte proliferation and migration. In addition, we showed that ASX-mediated keratinocyte stimulation is associated with the Cdc42, Rac1, and RhoA pathways. These results suggest mechanistic support for suggesting a potential utilization of ASX as a candidate for wound repair treatment. In our study, we found that a 72 h ASX treatment induced keratinocyte proliferation by increasing the proportion of cells in the S phase with a corresponding decline in the G1 phase. In previous studies, ASX significantly stimulated cultured human brain microvascular endothelial cells (HBMECs), rat aortic smooth muscle cells (RASMCs), and neural progenitor cell proliferation [2027]. Recently, ASX reportedly promoted the expression of cyclin D1 in HBMECs and RASMCs via the Wnt/β-catenin signaling pathway [20]. Our study demonstrates that ASX slightly induced keratinocyte proliferation after 72 h of treatment but did not affect proliferation at 24 and 48 h, suggesting that keratinocyte proliferation induced by ASX might not be a major pathway for ASX to promote skin re-epithelialization and wound repair.
Keratinocyte migration is a mechanism associated with the formation and disassembly of cell adhesion sites and cytoskeletal reorganization [6]. Cell migration is regulated by various signaling molecules, including the Rho family (Cdc42, Rac1, and RhoA) [4]. Herein, we demonstrated for the first time that ASX isolated from L. vannamei promoted keratinocyte migration in dose- and time-dependent manners. An increase in migration of keratinocytes after ASX treatment is advantageous for the initiation of wound closure, and augmentation of cell migration due to ASX is consistent with the results in a study of cultured HBMEC and RASMC [20]. The Rho family has a crucial role in the control of cell-cycle progression, cell growth and differentiation, cytoskeletal reorganization, and cell migration [28]. In addition, Rho family GTPases, including RhoA, Cdc42, and Rac1, have been suggested as pivotal factors in the regulation of cell polarization, protrusion, and adhesion during directional migration [729], and significant increases in Cdc42, Rac1, and RhoA activities are accompanied by a high rate of cell motility [3031]. During the migration process, the cell extends by forming protrusions, including large lamellipodia, small filopodia, and associated structures through extracellular matrix deposition [32]. In a migrating cell, Rac1 and Cdc42 are responsible for controlling actin polymerization to form lamellipodia and cell membrane protrusions in the front of the motile cell [83334]. In motile cells, formation of focal adhesion complexes and actin stress fibers has been associated with the activation of RhoA protein [56]. Our study revealed that ASX treatment increased cell protrusions (filopodia and lamellipodia), contributed to activation of Rac1 and Cdc42 and inhibited RhoA. These results supports those in a previous study reporting astrocytoma motility was modulated by the activation of Rac1 and correlated with RhoA inhibition [35]. Moreover, similar results were found in human promyelocytic leukemia HL-60 [36] and HaCaT cells [37], suggesting that multiple pseudopods develop after RhoA inhibition and Rac1 and Cdc42 activation, supporting our observation that ASX induces the formation of lamellipodia and actin polymerization through activation of Rac1 and inhibition of RhoA. Additionally, ASX enhances filopodia formation via Cdc42 activation.
The results of this pioneer study demonstrate the involvement of ASX in cutaneous wound closure via stimulation of keratinocyte migration and proliferation. The underlying mechanism for the positive effect of ASX on cell migration involves inhibition of RhoA and activation of Rac1 and Cdc42. Further in vivo and clinical studies are warranted to elucidate these pathways further as well as to develop ASX as a therapeutic agent for wound repair.
ACKNOWLEDGMENTS
We would to express a gratitude to Miss Somporn Sretrirutchai for the assistance with flow cytometry. Moreover, thanks to Dr. Brian Hodgson for assistance with the English.
Notes
References
1. Gurtner GC, Werner S, Barrandon Y, Longaker MT. Wound repair and regeneration. Nature. 2008; 453:314–321. PMID: 18480812.


2. Wadman M. Scar prevention: the healing touch. Nature. 2005; 436:1079–1080. PMID: 16121148.
3. Haase I, Evans R, Pofahl R, Watt FM. Regulation of keratinocyte shape, migration and wound epithelialization by IGF-1- and EGF-dependent signalling pathways. J Cell Sci. 2003; 116:3227–3238. PMID: 12829742.


4. Enciso JM, Konecny CM, Karpen HE, Hirschi KK. Endothelial cell migration during murine yolk sac vascular remodeling occurs by means of a Rac1 and FAK activation pathway in vivo. Dev Dyn. 2010; 239:2570–2583. PMID: 20737513.


5. Burridge K, Chrzanowska-Wodnicka M. Focal adhesions, contractility, and signaling. Annu Rev Cell Dev Biol. 1996; 12:463–518. PMID: 8970735.


6. Sepp KJ, Auld VJ. RhoA and Rac1 GTPases mediate the dynamic rearrangement of actin in peripheral glia. Development. 2003; 130:1825–1835. PMID: 12642488.


7. Nobes CD, Hall A. Rho GTPases control polarity, protrusion, and adhesion during cell movement. J Cell Biol. 1999; 144:1235–1244. PMID: 10087266.


8. Hsu CL, Muerdter CP, Knickerbocker AD, Walsh RM, Zepeda-Rivera MA, Depner KH, Sangesland M, Cisneros TB, Kim JY, Sanchez-Vazquez P, Cherezova L, Regan RD, Bahrami NM, Gray EA, Chan AY, Chen T, Rao MY, Hille MB. Cdc42 GTPase and Rac1 GTPase act downstream of p120 catenin and require GTP exchange during gastrulation of zebrafish mesoderm. Dev Dyn. 2012; 241:1545–1561. PMID: 22911626.


9. Rottner K, Hall A, Small JV. Interplay between Rac and Rho in the control of substrate contact dynamics. Curr Biol. 1999; 9:640–648. PMID: 10375527.


10. Kidd P. Astaxanthin, cell membrane nutrient with diverse clinical benefits and anti-aging potential. Altern Med Rev. 2011; 16:355–364. PMID: 22214255.
11. Naguib YM. Antioxidant activities of astaxanthin and related carotenoids. J Agric Food Chem. 2000; 48:1150–1154. PMID: 10775364.


12. Ranga Rao A, Baskaran V, Sarada R, Ravishankar GA. In vivo bioavailability and antioxidant activity of carotenoids from microalgal biomass: a repeated dose study. Food Res Int. 2013; 54:711–717.
13. Choi SK, Park YS, Choi DK, Chang HI. Effects of astaxanthin on the production of NO and the expression of COX-2 and iNOS in LPS-stimulated BV2 microglial cells. J Microbiol Biotechnol. 2008; 18:1990–1996. PMID: 19131704.
14. Kishimoto Y, Tani M, Uto-Kondo H, Iizuka M, Saita E, Sone H, Kurata H, Kondo K. Astaxanthin suppresses scavenger receptor expression and matrix metalloproteinase activity in macrophages. Eur J Nutr. 2010; 49:119–126. PMID: 19784539.


15. Kuedo Z, Sangsuriyawong A, Klaypradit W, Tipmanee V, Chonpathompikunlert P. Effects of astaxanthin from Litopenaeus vannamei on carrageenan-induced edema and pain behavior in mice. Molecules. 2016; 21:382. PMID: 27007359.


16. Camera E, Mastrofrancesco A, Fabbri C, Daubrawa F, Picardo M, Sies H, Stahl W. Astaxanthin, canthaxanthin and β-carotene differently affect UVA-induced oxidative damage and expression of oxidative stress-responsive enzymes. Exp Dermatol. 2009; 18:222–231. PMID: 18803658.


17. Hama S, Takahashi K, Inai Y, Shiota K, Sakamoto R, Yamada A, Tsuchiya H, Kanamura K, Yamashita E, Kogure K. Protective effects of topical application of a poorly soluble antioxidant astaxanthin liposomal formulation on ultraviolet-induced skin damage. J Pharm Sci. 2012; 101:2909–2916. PMID: 22628205.


18. Mizuta M, Hirano S, Hiwatashi N, Tateya I, Kanemaru S, Nakamura T, Ito J. Effect of astaxanthin on vocal fold wound healing. Laryngoscope. 2014; 124:E1–E7. PMID: 23686840.


19. Praveenkumar R, Gwak R, Kang M, Shim TS, Cho S, Lee J, Oh YK, Lee K, Kim B. Regenerative astaxanthin extraction from a single microalgal (Haematococcus pluvialis) cell using a gold nano-scalpel. ACS Appl Mater Interfaces. 2015; 7:22702–22708. PMID: 26397314.


20. Xu Y, Zhang J, Jiang W, Zhang S. Astaxanthin induces angiogenesis through Wnt/β-catenin signaling pathway. Phytomedicine. 2015; 22:744–751. PMID: 26141761.


21. Schlessinger K, Hall A, Tolwinski N. Wnt signaling pathways meet Rho GTPases. Genes Dev. 2009; 23:265–277. PMID: 19204114.


22. Sangsuriyawong A, Limpawattana M, Siriwan D, Kaewnern P, Klaypradit W. Effects of phospholipid concentration and mixing methods on properties of astaxanthin extract-loaded liposomes. J Agric. 2016; 32:421–433.
23. Taksima T, Limpawattana M, Klaypradit W. Astaxanthin encapsulated in beads using ultrasonic atomizer and application in yogurt as evaluated by consumer sensory profile. LWT Food Sci Technol. 2015; 62:431–437.
24. Gammone MA, Riccioni G, D'Orazio N. Marine carotenoids against oxidative stress: effects on human health. Mar Drugs. 2015; 13:6226–6246. PMID: 26437420.


25. Santoro MM, Gaudino G. Cellular and molecular facets of keratinocyte reepithelization during wound healing. Exp Cell Res. 2005; 304:274–286. PMID: 15707592.


27. Kim JH, Nam SW, Kim BW, Choi W, Lee JH, Kim WJ, Choi YH. Astaxanthin improves stem cell potency via an increase in the proliferation of neural progenitor cells. Int J Mol Sci. 2010; 11:5109–5119. PMID: 21614195.


28. Hodge RG, Ridley AJ. Regulating Rho GTPases and their regulators. Nat Rev Mol Cell Biol. 2016; 17:496–510. PMID: 27301673.


29. Fukata M, Nakagawa M, Kaibuchi K. Roles of Rho-family GTPases in cell polarisation and directional migration. Curr Opin Cell Biol. 2003; 15:590–597. PMID: 14519394.
30. Li J, Zhang S, Soto X, Woolner S, Amaya E. ERK and phosphoinositide 3-kinase temporally coordinate different modes of actin-based motility during embryonic wound healing. J Cell Sci. 2013; 126:5005–5017. PMID: 23986484.


31. Zhang Z, Yang M, Chen R, Su W, Li P, Chen S, Chen Z, Chen A, Li S, Hu C. IBP regulates epithelial-to-mesenchymal transition and the motility of breast cancer cells via Rac1, RhoA and Cdc42 signaling pathways. Oncogene. 2014; 33:3374–3382. PMID: 23975422.


33. Raftopoulou M, Hall A. Cell migration: Rho GTPases lead the way. Dev Biol. 2004; 265:23–32. PMID: 14697350.


34. Zhao X, Guan JL. Focal adhesion kinase and its signaling pathways in cell migration and angiogenesis. Adv Drug Deliv Rev. 2011; 63:610–615. PMID: 21118706.


35. Salhia B, Rutten F, Nakada M, Beaudry C, Berens M, Kwan A, Rutka JT. Inhibition of Rho-kinase affects astrocytoma morphology, motility, and invasion through activation of Rac1. Cancer Res. 2005; 65:8792–8800. PMID: 16204049.


36. Xu J, Wang F, Van Keymeulen A, Herzmark P, Straight A, Kelly K, Takuwa Y, Sugimoto N, Mitchison T, Bourne HR. Divergent signals and cytoskeletal assemblies regulate self-organizing polarity in neutrophils. Cell. 2003; 114:201–214. PMID: 12887922.


37. Petpiroon N, Suktap C, Pongsamart S, Chanvorachote P, Sukrong S. Kaempferol-3-O-rutinoside from Afgekia mahidoliae promotes keratinocyte migration through FAK and Rac1 activation. J Nat Med. 2015; 69:340–348. PMID: 25783411.


Fig. 1
Cytotoxicity effect of ASX on human skin keratinocyte cells.
Cells were exposed to 10-1,000 µg/mL of ASX for 24, 48, and 72 h. Cell viability was determined by MTT assay. The results were presented as a percentage of the control mean. Data are expressed as mean ± SEM values (n = 4). #P < 0.01, *P < 0.001 versus an untreated control.
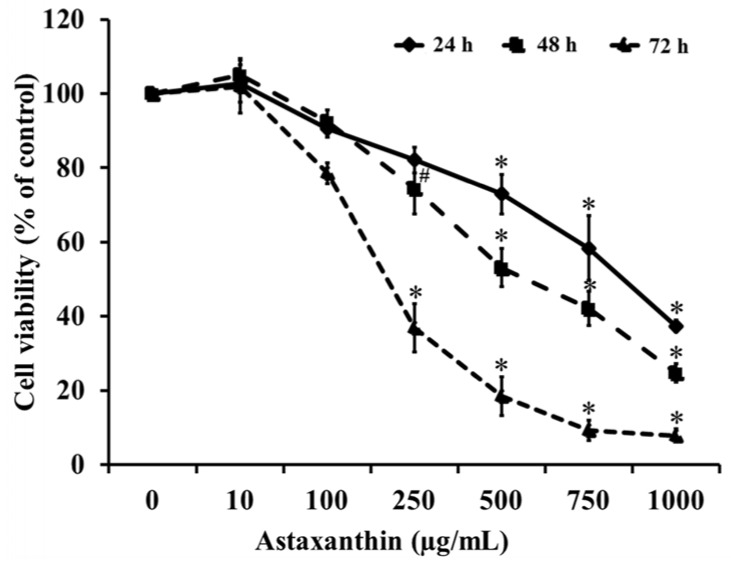
Fig. 2
Proliferative effects of ASX on human skin keratinocyte cells.
(A) HaCaT cells were treated with various concentrations (0.125-10 µg/mL) of ASX for 24, 48, and 72 h. Cell proliferation was determined by MTT assay. Cells were incubated with 0.25-1 µg/mL of ASX for 72 h. (B) Percentage values of cell-cycle cell-phase distribution. (C) Distribution of cells in each phase as detected by flow cytometry and PI. Data are expressed as mean ± SEM values (n = 4). †P < 0.05, *P < 0.001 compared to a control group.
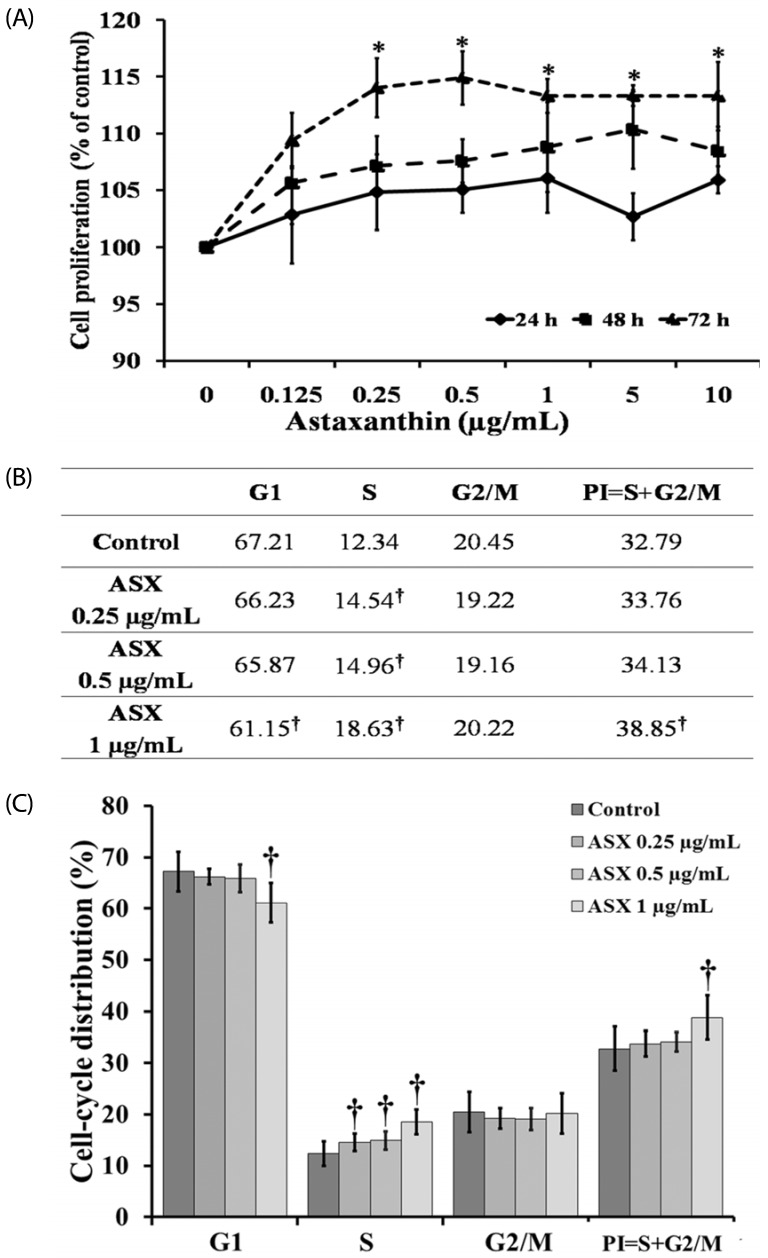
Fig. 3
Effect of ASX on the migration of skin keratinocyte cells.
HaCaT cells were treated with various concentrations (0, 0.25, 0.5 or 1 µg/mL) of ASX for 0, 9, 24, 48, and 72 h. (A) Cell migration was visualized via phase-contrast microscopy. (B) The percentage wound closure area was measured by comparing the change in wound area to that of the control. Data are expressed as mean ± SEM values of four independent experiments. Difference among groups was evaluated by one-way ANOVA. *P < 0.001 compared to time 0, **P < 0.001 compared to a control.
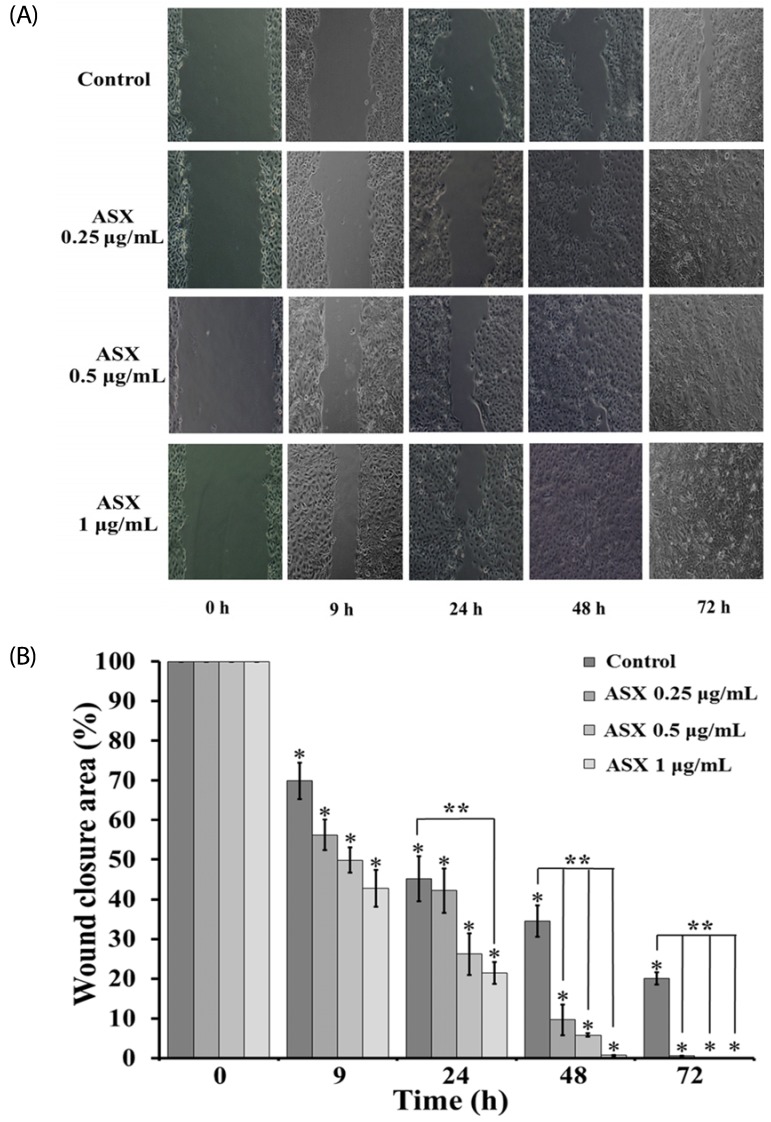
Fig. 4
The formation of filopodia and lamellipodia, and the relative blot density of Cdc42, Rac1, and RhoA in response to ASX treatment.
(A) HaCaT cells were stained with phalloidin-rhodamine. (B) ASX-treated cells were subjected to western blotting after 9 h of ASX exposure, and expression levels of Cdc42, Rac1 and RhoA were assessed. (C) Relative levels of Cdc42, Rac1 and RhoA in HaCaT cells after treatment with ASX (0.25-1 µg/mL) for 9 h. Densitometric analysis results for the indicated proteins are presented as mean ± SEM values of three independent experiments, #P < 0.01, *P < 0.001 compared to a control.
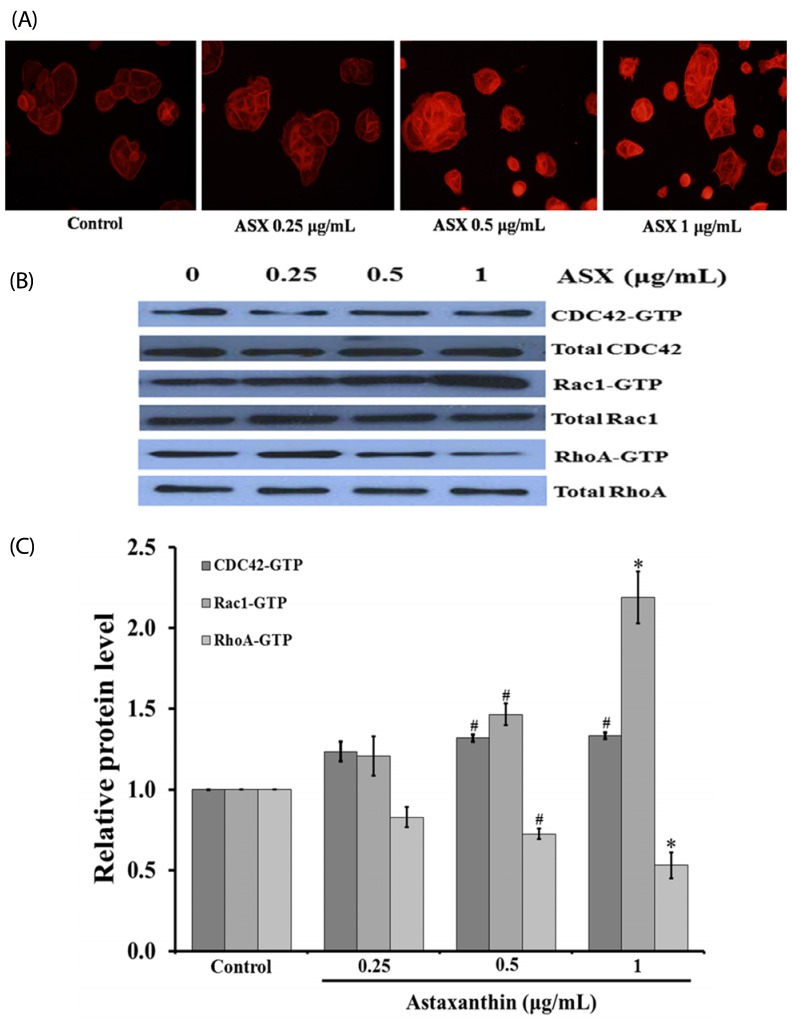