Abstract
BACKGROUND/OBJECTIVES
Helicobacter pylori (H. pylori) colonization of the stomach mucosa and duodenum is the major cause of acute and chronic gastroduodenal pathology in humans. Efforts to find effective anti-bacterial strategies against H. pylori for the non-antibiotic control of H. pylori infection are urgently required. In this study, we used whey to prepare glycomacropeptide (GMP), from which sialic acid (G-SA) was enzymatically isolated. We investigated the anti-bacterial effects of G-SA against H. pylori in vitro and in an H. pylori-infected murine model.
MATERIALS/METHODS
The anti-bacterial activity of G-SA was measured in vitro using the macrodilution method, and interleukin-8 (IL-8) production was measured in H. pylori and AGS cell co-cultures by ELISA. For in vivo study, G-SA 5 g/kg body weight (bw)/day and H. pylori were administered to mice three times over one week. After one week, G-SA 5 g/kg bw/day alone was administered every day for one week. Tumor necrosis factor-α (TNF-α), IL-1β, IL-6, and IL-10 levels were measured by ELISA to determine the anti-inflammatory effects of G-SA. In addition, real-time PCR was performed to measure the genetic expression of cytotoxin-associated gene A (cagA).
RESULTS
G-SA inhibited the growth of H. pylori and suppressed IL-8 production in H. pylori and in AGS cell co-cultures in vitro. In the in vivo assay, administration of G-SA reduced levels of IL-1β and IL-6 pro-inflammatory cytokines whereas IL-10 level increased. Also, G-SA suppressed the expression of cagA in the stomach of H. pylori-infected mice.
CONCLUSION
G-SA possesses anti-H. pylori activity as well as an anti-H. pylori-induced gastric inflammatory effect in an experimental H. pylori-infected murine model. G-SA has potential as an alternative to antibiotics for the prevention of H. pylori infection and H. pylori-induced gastric disease prevention.
Helicobacter pylori (H. pylori) was first discovered in 1982 and was subsequently found to be an important etiological agent for chronic gastritis, gastroduodenal ulcer disease, and gastric malignancy [12]. H. pylori is a Gram-negative spiral-shaped microaerophilic bacterium that colonizes the mucus layer and adheres to the surface epithelium of the stomach [3].
The initial step in H. pylori infection is penetration and adherence in the stomach via binding to mucin and gastric epithelial cells of the gastric antrum through several different adhesion molecules [45]. During H. pylori-gastric epithelial cell interaction, H. pylori injects the cytotoxin-associated gene A (cagA) protein directly into epithelial cells, which induces interleukin-8 (IL-8) production in the host cell. Prolonged IL-8 production during H. pylori infection could result in the recruitment of chronic leucocytes to infected tissues and can also lead to the production of pro-inflammatory cytokines (e.g., tumor necrosis factor-α [TNF-α], IL-6 and IL-1β) by the gastric mucosa from activated inflammatory cells [678]. H. pylori-initiated chronic gastric inflammation is characterized by cagA upregulation in the gastric tissue, which is largely mediated by the activation of nuclear factor-κB (NF-κB) as a transcription factor. The cagA gene is thought to be associated with gastroduodenal disease [910].
Sialic acid (SA, N-acetylneuraminic acid) contains a nine-carbon backbone and is one of the most diverse sugars found on glycan chains of the mammalian cell surface. SA plays an important role in many physiological and pathological processes in the host including infection control and the regulation of innate immunity. Microbes have evolved various strategies to subvert these processes by decorating their surface with sialylated oligosaccharides that mimic those of the host [1112]. Whey contains a high level of glycomacropeptide (GMP), and SA can be enzymatically isolated from whey GMP (G-SA). In this study, we demonstrate preventive effects of G-SA in the control of H. pylori infection in vitro and in vivo.
Synthetic sialic acid (S-SA) was obtained from Marukin Bio, Inc. (Kyoto, Japan) and contained 99.9% SA. G-SA was obtained from Hanilbiomed, Inc. (Gwangju, Korea) and was enzymatically isolated from glycomacropeptide (GMP, Murray Goulburn Co-Operative Co., Ltd, Victoria, Australia). 7% (w/v) NatraPrep GMP containing 7% SA dissolved in the distilled water, and the pH of the solution was adjusted to 7.0, 0.2% protease (Novozymes Korea Ltd, Seoul, Republic of Korea) was added to GMP solution, and the solution was incubated for 1 h at 57℃ for the hydrolysis through enzyme reaction. Concentration of G-SA was determined by high-performance liquid chromatography (HPLC, Agilent Technologies, Santa Clara, CA, USA) analysis. A 7% G-SA solution was used as stock to prepare a 0.05% to 1% concentration gradient that contained final sialic acid concentrations of 0.0035%, 0.007%, 0.0175%, 0.035%, 0.052%, and 0.07%.
H. pylori Sydney strain (SS)-1 was kindly provided by the Helicobacter pylori Korean Type Culture Collection (HpKTCC, Jinju, Republic of Korea) and was cultured in Brucella broth (Difco Laboratories, Detroit, MI, USA) with 10% fetal bovine serum (FBS, Hyclone Laboratories, Logan, Utah, USA) with antibiotics (20 µg/mL vancomycin, 20 µg/mL trimethoprim) and was incubated for 72 h under a microaerobic environment.
The AGS human gastric adenocarcinoma cell line (KCLB 21739) was purchased from the Korean Cell Line Bank (KCLB, Seoul, Republic of Korea). The cells were cultured in RPMI-1640 medium (Gibco, Carlsbad, CA, USA) supplemented with 10% fetal bovine serum (FBS), 2 mM L-glutamine, 100 U/mL penicillin, and 100 µg/mL streptomycin (Sigma-Aldrich, St. Louis, MO, USA) at 37℃ in an atmosphere of 5% CO2. For co-culture of H. pylori and AGS cells, AGS cells were seeded in 24-well cell culture plates at 2 × 105 cells per well. H. pylori and AGS co-culture experiments were performed at a multiplicity of infection (MOI) of 100 and were co-cultured for 2 h, 4 h, and 6 h in the absence or presence of SA in a CO2 incubator. After each time point, the culture supernatants were collected and stored at -20℃ until use.
Cell viability was assessed using 3-(4,5-dimethylthiazol-2yl)-2,5-diphenyl-tetrazolium bromide (MTT) (Sigma-Aldrich, St. Louis, MO, USA). AGS cells were cultured with SA at various concentrations. After incubation for 24 h, 20 µL of MTT solution (5 mg/mL in phosphate buffer saline (PBS) as stock solution) was added to each well, and the cells were incubated at 37℃ for 4 h. The supernatants were removed, and dimethyl sulfoxide (DMSO) was then added to each well. The plates were analyzed at 560 nm to determine the percentage of viable cells.
H. pylori were grown in Brucella broth under a microaerobic environment for 72 h and collected in mid-log phase. H. pylori (50 µL of 1 × 104 CFU/mL) were serially diluted with SA into 2 mL of Brucella broth. After incubation for 24 h, 50 µL of each diluted suspension was spread onto Brucella agar, and the plates were incubated for 72 h under microaerobic conditions. Viability was evaluated by determining the number of colony forming units (CFU), and the results are presented on a logarithmic scale.
The Experimental Animal Ethics Committee of Dankook University (No DKU14-036) approved the experimental procedures in this study. Specific pathogen-free (SPF) male ICR mice (20-22 g, 5-weeks-old) were purchased from Orient Bio (Daejeon, Republic of Korea). All the animals were housed in an SPF environment and had free access to sterile neutral water and standard mouse feed. Thirty-two mice were randomly divided into 4 groups of 8 mice each. The mice in the experimental group were treated with S-SA or G-SA 5 g/kg body weight (bw)/day and 1 × 109 CFU of H. pylori three times per week; after one week, SA only was administered every day for one week. Uninfected control mice received distilled water only.
The expression levels of TNF-α, IL-1β, IL-6, and IL-10 in serum were measured using an enzyme-linked immunosorbent assay (ELISA, R&D Systems, Minneapolis, MN, USA) according to the manufacturer's instructions. The assay was performed in triplicate.
Small pieces of gastric tissue from the antrum and stomach were homogenized in lysis buffer including 1% β-mercaptoethanol. Total mRNA was isolated from the gastric tissue lysate using the PureLink™ RNA mini kit (Ambion Life Technologies, Carlsbad, CA, USA), and cDNA was synthesized using the iScript™ cDNA synthesis kit (Bio-Rad, Hercules, CA, USA). To quantify mRNA expression, real-time polymerase chain reaction (PCR) was performed using a LightCycler System (Jena Bioscience, Jena, Germany). The cDNA was amplified for 40 cycles of denaturation (95℃ for 30 s), annealing (58℃ for 30 s), and extension (72℃ for 45 s) using the following primers: GAPDH forward primer 5′-GTC-TTC-ACC-ACC-ATG-GAG-AAG-GCT-3′, reverse primer 5′-CAT-GCC-AGT-GAG-CTT-CCC-GTT-CA-3′; and cagA forward primer 5′-GCT-TAC-CGC-CTG-AAG-CTA-GG-3′, reverse primer 5′-CCTTTC-TCA-CCA-CCT-GCT-ATG-3′. Concomitantly, amplification of control mouse GAPDH was performed on the same samples to assess RNA integrity.
The viability of G-SA-treated AGS cells was significantly decreased by 75% (P < 0.05), whereas S-SA had no effect on AGS cells (Fig. 1). Therefore, S-SA and G-SA treatments between 0.05 and 0.5% were used in the subsequent experiments.
The anti-bacterial activity of G-SA against H. pylori was evaluated using the macrodilution method. H. pylori cultures were incubated for 24 h in the presence of the G-SA at concentrations ranging from 0.1 to 0.5%. G-SA significantly decreased the number of colonies in 0.5% G-SA (P < 0.05) that contained a final concentration of 0.035% SA compared to 0.5% S-SA that contained 0.5% SA (Fig. 2). These results suggest that G-SA possess anti-bacterial activity similar to that of S-SA.
In this study, we evaluated the effect of G-SA on H. pylori-induced IL-8 production from AGS cells for different time periods. We found that H. pylori with 2 to 6 h of 0.5% G-SA exposure retained the ability to induce IL-8 secretion, similar to unexposed cells (Fig. 3). The H. pylori-induced IL-8 production in 0.5% S-SA-treated cells was not different compared with H. pylori control, whereas in 0.5% G-SA-treated cells was strongly inhibited by > 95% compared with H. pylori control during the infection period (2 to 6 h). Thus, H. pylori-induced IL-8 production was more strongly inhibited by G-SA than S-SA. G-SA might inhibit IL-8 production in the H. pylori-infected gastric epithelium through its anti-bacterial activity against H. pylori. One possible explanation is that G-SA directly inhibits the growth of colonized H. pylori on AGS cells during the short infection period (2 to 6 h) and indirectly reduces bacterial provocation of host cell inflammation. This possibility is supported by our results in the antibacterial susceptibility test of G-SA against H. pylori (Fig. 2).
After administration of G-SA, normal physical conditions and clinical signs were observed in all groups during the experimental period. All mice were weighed before oral H. pylori infection on day 0 and after oral H. pylori infection day 21. There was no significant difference in total body weight gain or average daily food intake between the G-SA group and sham group over the experimental period (Table 1). However, the S-SA group was observed to be cachexic and lost approximately 13% body weight compared to the sham group.
The levels of the pro-inflammatory cytokines TNF-α, IL-1β, and IL-6 were significantly increased in the sham group compared with the normal control group (P < 0.05) (Fig. 4). Both IL-1β and IL-6 levels decreased significantly in the S-SA and G-SA groups compared with the sham group (P < 0.05). There was no significant between-group difference in these parameters in the S-SA and G-SA groups; however, TNF-α level was not significantly different among any of the sham or SA-administrated groups. In addition, anti-inflammatory cytokine IL-10 was significantly increased in the G-SA group compared with the sham group. These findings suggest that G-SA reduces severe gastropathy in H. pylori-infected mice, and that G-SA action is independent of pro-inflammatory cytokine downregulation and/or anti-inflammatory cytokine upregulation.
We determined whether G-SA affects the expression of the cagA virulence factor gene in the gastric antrum of H. pylori-infected mice by real-time PCR. As shown in Fig. 5, cagA expression was significantly increased in the sham group compared with that in the normal control group (P < 0.05). In contrast, a significant decrease in cagA expression was observed in the G-SA group compared with that of the sham group (P < 0.05). These results suggest that G-SA suppresses the expression of this major pathogenic virulence factor in H. pylori.
Non-antibiotic therapies, including phytochemicals, probiotics, and antioxidants, have been increasingly investigated as potential alternatives for the treatment of H. pylori [1314]. Despite many studies of H. pylori treatment, the ideal regimen for treating the infection has not been found.
Yang JC et al. [15] showed that SA alone did not demonstrate anti-H. pylori effects, whereas treatment with catechins/SA led to synergistic anti-H. pylori activity. In contrast, we evaluated the in vitro efficacy of SA isolated from the GMP of whey for the treatment of H. pylori. We confirmed that H. pylori growth was significantly inhibited by 0.5% G-SA that contained 0.014% SA. According to our results, the anti-bacterial activity of SA isolation from GMP derived from the whey of milk on H. pylori is novel.
The pathogenesis of H. pylori is contributed by many virulence factors, including cagA gene expression. H. pylori infection with cagA-positive strains might result in severe gastric inflammation and gastric cancer [16]. The initial step in H. pylori infection is penetration and adherence in the stomach, followed by cagA translocation into the epithelial cells [45]. cagA affects expression of the chemotactic cytokine IL-8 in gastric epithelial cells, and IL-8 plays an important role in recruiting and activating neutrophils. Several studies have found that gastric mucosal levels of cagA and IL-8 correlate with histological severity in patients with H. pylori-induced gastritis [17]. Thus, cagA and IL-8 may be components of the inflammatory cascade.
In the present study, we used a mouse model infected with H. pylori SS-1 [18] to examine the preventive effects of G-SA on cagA-mediated inflammation by H. pylori. We found that cagA mRNA level was significantly increased in the stomach of the sham group compared with that of the normal control group. However, the administration of G-SA significantly decreased cagA mRNA expression level in the stomach compared with that in the sham group.
H. pylori leads to increased production of IL-8 by epithelial cells when in close contact with the gastric mucosa [19]. We investigated the ability of H. pylori to induce inflammation by evaluating the IL-8 secretion from AGS cells infected with H. pylori (MOI of 100) exposed to G-SA for different time periods. We found that G-SA repressed IL-8 secretion in the epithelial cells. In addition, G-SA suppressed IL-8 secretion to lower levels compared with S-SA. Simon PM et al. [20] demonstrated that SA-containing oligosaccharides (3'-sialyllactose) inhibited the ability of H. pylori to bind to epithelial cells in vitro. According to these findings, we suggest that the reduced IL-8 production could be attributed to the G-SA-induced inhibition of bacterial binding to the gastric epithelium through control of cagA gene expression.
During H. pylori infection, upregulation of cagA-mediated IL-8 produced pro-inflammatory cytokines (TNF-α, IL-6, and IL-1β) in the gastric mucosa, which was derived from activated inflammatory cells via the inflammatory NF-κB and mitogen-activated protein kinase (MAPK) signaling pathways [678]. Several studies have also demonstrated that TNF-α, IL-6, and IL-1β play important roles in the progression of pyloric gastritis in H. pylori-infected gerbils [21]. We found that the production of pro-inflammatory cytokines TNF-α, IL-1β, and IL-6 was significantly increased in the sham group compared with that in the normal control group. IL-1β and IL-6 levels were significantly decreased in the G-SA group compared with those in the sham group. However, TNF-α level was not significantly different in the G-SA group compared with that in the sham group. Several reports demonstrated reverse role of TNF-α in immunity. For example, In the H. pylori-induced gastritis in in vivo animal model system, Yamamoto T et al. [22] demonstrated no effect on the grade of gastritis in TNF-α-deficient mice compared with isogenic wild-type controls. Also, Thalmaier U et al. [23] reported that there was no detectable difference in the grade or activity of gastritis in TNF-R1-deficient mice when they were compared with wild-type mice. Thus, gastric inflammation in H. pylori infection seems to be independent of the function of the TNF-α. Our results are in agreement with their results. We are expecting no change in TNF-α by independent pathway in H. pylori-induced in in vivo mice model.
IL-10 is a potent anti-inflammatory cytokine with a variety of biological effects, including inhibition of other pro-inflammatory cytokines and chemokines in H. pylori-induced gastritis [2425]. Berg DJ et al. [26] have reported that H. pylori infection is supported by the recent observation of the rapid development of severe hyperplastic gastritis in Helicobacter felis-infected IL-10 (-/-) knockout mice. IL-10 was significantly increased in the G-SA group compared with that in the sham group, indicating that the enhanced IL-10 level reduce local immune responses against mucosal infection by H. pylori.
In summary, during H. pylori infection, bacteria first attach to surface mucous cells of the gastric mucosa, an attachment facilitated by binding of the H. pylori adhesion molecule, sialic acid-binding adhesin (SabA) via SA [27]. Recently, H. pylori draws special attention, because its SabA is involved in its binding to oral and gastric mucins [27], which contained O-glycans with terminal α1,4-linked GlcNAc residues (including SA) and secreted from gland mucous cell [28]. Lee H et al. [29] reported that α1,4-GlcNAc-capped O-glycans suppress H. pylori growth. In addition, a combination of SA and catechins in the diet was shown to prevent H. pylori infection to decrease the bacterial load and inflammation in mouse stomach [15]. We suggest that inhibition of H. pylori binding to gastric epithelial cells through inhibition of SabA adhesion on H. pylori by G-SA (containing SA) isolation from GMP derived from the whey of milk. Thus, supplementation of G-SA could serve as potent anti-adhesion components against H. pylori. However, the anti-adhesion effect of G-SA needs a further study. G-SA may be used as a possible tool for the management of H. pylori infection.
Figures and Tables
![]() | Fig. 1Effects of G-SA on AGS cell viability.Cells were incubated with various concentrations of SA for 24 h, after which cell viability was measured using MTT assay. Data are presented as growth rate of cells cultured in the presence of SA compared to the percentage of untreated control cells, taken as 100%. Data are expressed as mean ± SD (n = 3). Significant differences were determined using Duncan's multiple range test at P < 0.05. S-SA, synthetic sialic acid; G-SA, enzymatically isolated sialic acid from GMP.
|
![]() | Fig. 2The anti-bacterial activity of G-SA was evaluated by the macrodilution method, and the results are shown on a logarithmic scale.The experiments were carried out using three different SA concentrations (0.1%, 0.25%, 0.5%). Data are expressed as mean ± SD (n = 8). Significant differences were determined using Duncan's multiple range test at P < 0.05. S-SA, synthetic sialic acid; G-SA, enzymatically isolated sialic acid from GMP.
|
![]() | Fig. 3Effect of G-SA on H. pylori adhesion to host human epithelial cellsAGS cells were infected with H. pylori SS1 inocula exposed to SA for (A) 2 h, (B) 4 h, and (C) 6 h at a multiplicity of infection (MOI) of 100:1 (H. pylori:AGS). Supernatants from H. pylori infected-AGS cells were collected and used to determine IL-8 concentration as evaluated by ELISA. Data are expressed as mean ± SD (n = 3). Significant differences were determined using Duncan's multiple range test atP < 0.05. # P < 0.05 compared with unexposed cells. *P < 0.05 compared with H. pylori inoculation control. S-SA, synthetic sialic acid; G-SA, enzymatically isolated sialic acid from GMP.
|
![]() | Fig. 4Effects of G-SA on pro-inflammatory cytokine levels in the serum of H. pylori-infected mice(A) TNF-α, (B) IL-1β, (C) IL-6, and (D) IL-10 concentrations were determined by enzyme-linked immunosorbent assay (ELISA). Normal control (NC), no infection; Sham, experimental H. pylori infection; S-SA, experimental H. pylori infection + synthetic sialic acid (5 g/kg bw/day); G-SA, experimentally H. pylori infection + enzymatically isolated sialic acid from GMP (5 g/kg bw/day). Data are expressed as mean ± SD (n = 8). Significant differences were determined using Duncan's multiple range test at P < 0.05. #P <0.05 compared with NC group. *P < 0.05 compared with sham group.
|
![]() | Fig. 5Effect of G-SA on cagA expression in H. pylori-infected gastric tissue.The mRNA level of cagA was determined by real-time PCR. NC, normal control, no infection; Sham, experimental H. pylori infection; S-SA, experimental H. pylori infection + synthetic sialic acid (5 g/kg bw/day); G-SA, experimentally H. pylori infection + enzymatically isolated sialic acid from GMP (5 g/kg bw/day). Data are expressed as mean ± SD (n = 8). Significant differences were determined using Duncan's multiple range test at P < 0.05. # P < 0.05 compared with NC group. * P < 0.05 compared with sham group.
|
Table 1
Body weight change in H. pylori-infected ICR mice receiving G-SA treatment.
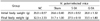
Normal control (NC), no infection; Sham, experimental H. pylori infection; S-SA, experimental H. pylori infection + synthetic sialic acid (5 g/kg bw/day); G-SA, experimental H. pylori infection + enzymatically isolated sialic acid from GMP (5 g/kg bw/day). Data are expressed as mean ± SD (n = 8). Significant differences were determined using Duncan's multiple range test at P < 0.05.
Notes
References
1. Suerbaum S, Michetti P. Helicobacter pylori infection. N Engl J Med. 2002; 347:1175–1186.
2. Venerito M, Vasapolli R, Rokkas T, Malfertheiner P. Helicobacter pylori and gastrointestinal malignancies. Helicobacter. 2015; 20:Suppl 1. 36–39.


3. Cover TL, Blaser MJ. Helicobacter pylori infection, a paradigm for chronic mucosal inflammation: pathogenesis and implications for eradication and prevention. Adv Intern Med. 1996; 41:85–117.
4. Borén T, Falk P, Roth KA, Larson G, Normark S. Attachment of Helicobacter pylori to human gastric epithelium mediated by blood group antigens. Science. 1993; 262:1892–1895.


5. Kobayashi Y, Okazaki K, Murakami K. Adhesion of Helicobacter pylori to gastric epithelial cells in primary cultures obtained from stomachs of various animals. Infect Immun. 1993; 61:4058–4063.


6. Bodger K, Crabtree JE. Helicobacter pylori and gastric inflammation. Br Med Bull. 1998; 54:139–150.
7. Huang J, O'Toole PW, Doig P, Trust TJ. Stimulation of interleukin-8 production in epithelial cell lines by Helicobacter pylori. Infect Immun. 1995; 63:1732–1738.


8. Harris PR, Mobley HL, Perez-Perez GI, Blaser MJ, Smith PD. Helicobacter pylori urease is a potent stimulus of mononuclear phagocyte activation and inflammatory cytokine production. Gastroenterology. 1996; 111:419–425.


9. Jones KR, Whitmire JM, Merrell DS. A tale of two toxins: Helicobacter pylori CagA and VacA modulate host pathways that impact disease. Front Microbiol. 2010; 1:115.


10. Kaji T, Ishihara S, Ashizawa N, Hamamoto N, Endo H, Fukuda R, Adachi K, Watanabe M, Nakao M, Kinoshita Y. Adherence of Helicobacter pylori to gastric epithelial cells and mucosal inflammation. J Lab Clin Med. 2002; 139:244–250.


11. Varki NM, Varki A. Diversity in cell surface sialic acid presentations: implications for biology and disease. Lab Invest. 2007; 87:851–857.


12. Vimr ER, Kalivoda KA, Deszo EL, Steenbergen SM. Diversity of microbial sialic acid metabolism. Microbiol Mol Biol Rev. 2004; 68:132–153.


13. Carson CF, Riley TV. Non-antibiotic therapies for infectious diseases. Commun Dis Intell Q Rep. 2003; 27:Suppl. S143–S146.
14. Kamiji MM, de Oliveira RB. Non-antibiotic therapies for Helicobacter pylori infection. Eur J Gastroenterol Hepatol. 2005; 17:973–981.


15. Yang JC, Shun CT, Chien CT, Wang TH. Effective prevention and treatment of Helicobacter pylori infection using a combination of catechins and sialic acid in AGS cells and BALB/c mice. J Nutr. 2008; 138:2084–2090.


16. Wang YC. Medicinal plant activity on Helicobacter pylori related diseases. World J Gastroenterol. 2014; 20:10368–10382.
17. Ando T, Kusugami K, Ohsuga M, Shinoda M, Sakakibara M, Saito H, Fukatsu A, Ichiyama S, Ohta M. Interleukin-8 activity correlates with histological severity in Helicobacter pylori-associated antral gastritis. Am J Gastroenterol. 1996; 91:1150–1156.
18. Lee A, O'Rourke J, De Ungria MC, Robertson B, Daskalopoulos G, Dixon MF. A standardized mouse model of Helicobacter pylori infection: introducing the Sydney strain. Gastroenterology. 1997; 112:1386–1397.


19. Shimoyama T, Crabtree JE. Bacterial factors and immune pathogenesis in Helicobacter pylori infection. Gut. 1998; 43:Suppl 1. S2–S5.
20. Simon PM, Goode PL, Mobasseri A, Zopf D. Inhibition of Helicobacter pylori binding to gastrointestinal epithelial cells by sialic acid-containing oligosaccharides. Infect Immun. 1997; 65:750–757.


21. Kudo T, Lu H, Wu JY, Ohno T, Wu MJ, Genta RM, Graham DY, Yamaoka Y. Pattern of transcription factor activation in Helicobacter pylori-infected Mongolian gerbils. Gastroenterology. 2007; 132:1024–1038.


22. Yamamoto T, Kita M, Ohno T, Iwakura Y, Sekikawa K, Imanishi J. Role of tumor necrosis factor-alpha and interferon-gamma in Helicobacter pylori infection. Microbiol Immunol. 2004; 48:647–654.


23. Thalmaier U, Lehn N, Pfeffer K, Stolte M, Vieth M, Schneider-Brachert W. Role of tumor necrosis factor alpha in Helicobacter pylori gastritis in tumor necrosis factor receptor 1-deficient mice. Infect Immun. 2002; 70:3149–3155.


24. Bodger K, Wyatt JI, Heatley RV. Gastric mucosal secretion of interleukin-10: relations to histopathology, Helicobacter pylori status, and tumour necrosis factor-alpha secretion. Gut. 1997; 40:739–744.


25. Bodger K, Bromelow K, Wyatt JI, Heatley RV. Interleukin 10 in Helicobacter pylori associated gastritis: immunohistochemical localisation and in vitro effects on cytokine secretion. J Clin Pathol. 2001; 54:285–292.


26. Berg DJ, Lynch NA, Lynch RG, Lauricella DM. Rapid development of severe hyperplastic gastritis with gastric epithelial dedifferentiation in Helicobacter felis-infected IL-10(-/-) mice. Am J Pathol. 1998; 152:1377–1386.
27. Lindén SK, Wickström C, Lindell G, Gilshenan K, Carlstedt I. Four modes of adhesion are used during Helicobacter pylori binding to human mucins in the oral and gastric niches. Helicobacter. 2008; 13:81–93.


28. Nakayama J. Helicobacter pylori growth assay for glycans. In : Taniguchi N, Suzuki A, Ito Y, Narimatsu H, Kawasaki T, Hase S, editors. Experimental Glycoscience. Tokyo: Springer;2008. p. 227–229.