Abstract
BACKGROUND/OBJECTIVES
Artemisinin, a natural product isolated from Gaeddongssuk (artemisia annua L.) and its main active derivative, dihydroartemisinin (DHA), have long been used as antimalarial drugs. Recent studies reported that artemisinin is efficacious for curing diseases, including cancers, and for improving the immune system. Many researchers have shown the therapeutic effects of artemisinin on tumors such as breast cancer, liver cancer and kidney cancer, but there is still insufficient data regarding glioblastoma (GBM). Glioblastoma accounts for 12-15% of brain cancer, and the median survival is less than a year, despite medical treatments such as surgery, radiation therapy, and chemotherapy. In this study, we investigated the anti-cancer effects of DHA and transferrin against glioblastoma (glioblastoma multiforme, GBM).
MATERIALS/METHODS
This study was performed through in vitro experiments using C6 cells. The toxicity dependence of DHA and transferrin (TF) on time and concentration was analyzed by MTT assay and cell cycle assay. Observations of cellular morphology were recorded with an optical microscope and color digital camera. The anti-cancer mechanism of DHA and TF against GBM were studied by flow cytometry with Annexin V and caspase 3/7.
RESULTS
MTT assay revealed that TF enhanced the cytotoxicity of DHA against C6 cells. An Annexin V immune-precipitation assay showed that the percentages of apoptosis of cells treated with TF, DHA alone, DHA in combination with TF, and the control group were 7.15 ± 4.15%, 34.3 ± 5.15%, 66.42 ± 5.98%, and 1.2 ± 0.15%, respectively. The results of the Annexin V assay were consistent with those of the MTT assay. DHA induced apoptosis in C6 cells through DNA damage, and TF enhanced the effects of DHA.
CONCLUSION
The results of this study demonstrated that DHA, the derivative of the active ingredient in Gaeddongssuk, is effective against GBM, apparently via inhibition of cancer cell proliferation by a pharmacological effect. The role of transferrin as an allosteric activator in the GBM therapeutic efficacy of DHA was also confirmed.
Wormwoods have long been used in China for their anti-inflammatory, anti-pyretic, anti-cancer, anti-fungal, and anti-parasitic properties [123]. It was been reported that there are about 40 species of wormwoods in the Korea. Among them, Gaeddongssuk (artemisia annua L.) has been used as an edible plants and fermented beverage for a long time [4]. The active ingredients in Gaaeddongssuk have been identified as artemisinin, cadinene, coumarin, diterpenlactone, and eupatilin. Recent studies have reported that artemisinin is effective against cancers and that it has positive effects on the immune system [5]. Every artemisinin compound (artemisinin, artesunate, artemether, etc.) is metabolized to dihydroartemisinin (DHA) by replacing the C-10 lactone group of artemisinin with hemiacetal. There is also direct synthesis of DHA with the help of the organic chemical process. The use of sodium borohydride can reduce artemisinin to dihydroartemisinin in high production yields by replacing the carbonyl group of artemisinin with hemiacetal [6]. Additionally, DHA-piperaquine combination has been recommended as a first-line treatment of uncomplicated Plasmodium falciparum malaria by the WHO [4].
Many researchers have shown the therapeutic effects of artemisinin on tumors such as breast cancer, liver cancer and kidney cancer, but there is insufficient data regarding glioblastoma (GBM) [7]. Glioblastoma accounts for 12-15% of all brain cancers, and the median survival of patients with GBM is less than a year, despite medical treatments such as surgery, radiation therapy, and chemical treatment [8]. A few groups have reported that artemisinin and its analogues were toxic to brain cancer cells, but research on the treatment of GBM with DHA has been limited to date [910111213].
The artemisinin molecule contains an endoperoxide bridge that reacts with a ferrous iron atom to form free radicals [14]. The free radicals generated by the artemisinin and its derivatives induce macromolecular damage and cell death [9]. The cytotoxic effects of artemisinin are greater against cancer cells than normal cells because cancer cells take up more iron, which is an essential nutrient for cellular growth.
Transferrin is an iron-binding blood plasma glycoprotein [10]. Iron-loaded transferrin is transported into the cell by the transferrin receptor at the surface of the cell membrane [11]. Since transferrin plays a role as an iron transporter, it can improve the anti-cancer effects of artemisinin and its derivatives.
This study was conducted to characterize the anti-cancer effects of DHA and transferrin against glioblastoma (glioblastoma multiforme, GBM). The study was conducted via in vitro experiments and C6 cells. The toxicity dependence of DHA and transferrin (TF) on time and concentration was measured by MTT assay and cell cycle assay. The anti-cancer mechanism of DHA in combination with TF against GBM was studied by flow cytometry with Annexin V and caspase 3/7.
C6 cells belonging to the rat glioblastoma cell line (Korean Cell Line Bank, Seoul, Korea) were cultured in Dulbecco's modified Eagle's medium (DMEM) supplemented with 10% heat-inactivated fetal bovine serum and 1% penicillin/streptomycin (all purchased from Gibco; Thermo Fisher Scientific, Inc., Waltham, MA, USA) at 37℃ in a humidified atmosphere of 5% CO2. The cell lines were seeded at an initial density of 5 × 105 cells/ml in 75 cm2 flasks and passaged every third day.
C6 cells were seeded in a 96-well plate at a density of 1 × 104 cells/100 µl and treated with varying concentrations (10-1,000 µg/ml) of DHA (Sigma-Aldrich, St. Louis, MO, USA). The cells were maintained in the growth medium for 12 and 24 h, after which they were incubated with 0.5 mg/ml MTT (Sigma-Aldrich, St. Louis, MO, USA) at 37℃ for 4 h. The cells were then washed twice with PBS and lysed in 100 µl of DMSO for 5 min. Absorbance was measured at 540 nm in an ELx800uv micro plate reader (Biochrom, Holliston, MA, USA). The resultant data were expressed as the percentage of viable cells relative to untreated controls.
C6 cells were seeded under different conditions on cover glasses in 12 well culture plates (5 × 104/well). After treatment with DHA, TF or DHA and TF (Sigma-Aldrich, St. Louis, MO, USA), samples were incubated for 24 h at a temperature of 37℃ and CO2 concentration of 5%. After incubation they were washed three times with PBS and fixed with 2% formaldehyde and 2% glutaraldehyde (1:1) in PBS for 10 min at room temperature. The cells attached to the cover glass were washed again and mounted on glass slides with mounting medium. The results of observations were recorded with a Magnifier digital color camera (Optronics International, Chelmsford, MA, USA).
Cell preparation for the experiments can be classified into two stages. The first stage is a common process for all experiments in flow cytometry, and the second is specific to the Annexin V assay and Caspase 3/7 assay.
The processes for the common stage: C6 cells were seeded and incubated on cover glass in 12 well culture plates (5 × 104/well) with different media (DHA, TF, or DHA-TF combination). Samples were then washed with PBS and harvested after various times.
Sample preparation for cell cycle assay: C6 cells prepared by the common stage were fixed in 70% (v/v) ethanol for 1 hr at 4℃. The fixed cells were then centrifuged at 1,000 rpm over 3 min at room temperature, after which the supernatant was decanted. The pellet was then washed with PBS and re-suspended in 200 µl of cell cycle reagent. Finally, the re-suspended sample was kept at room temperature for 30 min in the dark.
Sample preparation for Annexin V assay: C6 cells prepared in the common stage were incubated in 100 mL Annexin V and dead cell reagent for 20 min at room temperature in the dark.
Sample preparation for Caspase 3/7 assay: 5 µl caspase-3/7 reagent working solution was added to C6 cells prepared by the common stage, then incubated for 30 min at 37℃ under 5% CO2. After the first incubation, 150 µl caspase 7-AAD working solution was added to the cells, and the solution was kept at room temperature for 5 min in the dark.
DHA and TF efficacy at inhibiting C6 cell proliferation was measured by MTT assay for samples incubated in DHA-TF containing medium for 12 h and 24 h each. MTT results are shown in Fig. 1. DHA suppressed the proliferation of C6 cells in both a dose- and time-dependent manner. Moreover, DHA exerted synergetic cytotoxicity against C6 cells when applied with TF.
PI labeling was used to quantify the percentage of C6 cells in various phases. The amount of bound dye correlates with the DNA content. DNA fragmentation in apoptotic cells translates into fluorescence intensity in the sub-G0/G1 phase, and is lower than that of G0/G1 cells (Fig. 2). The cell cycle assay revealed that the proportion of C6 cells in the sub-G0/G1 phase increased to 29.6% after incubation with 200 µM of DHA for 24 h, while it was 0.5% for the control group. Moreover, the proportion of cells in the sub-G0/G1 phase increased to 35.8% for cells incubated with 200 µM of DHA in combination with 200 µM of TF. These findings indicate that DHA induced apoptosis in C6 cells is caused by DNA damage, and that TF enhances the effects of DHA.
The percentage of apoptotic cells was determined by FITC-labeled Annexin V and PI double-staining flow cytometry (Fig. 3). At 24 h after administration, the percentages of apoptosis for cells treated with TF, DHA, DHA in combination with TF, and the control group were 7.15 ± 4.15%, 34.3 ± 5.15%, 66.42 ± 5.98%, and 1.2 ± 0.15%, respectively.
In accordance with the Annexin V assay, the compatible morphological changes were observed (Fig. 4). Increased numbers of cells with damaged morphology were found in the DHA treatment group, while more damage was observed in the DHA-TF combined treatment group.
As shown in Fig. 5, the results of the caspase 3/7 immune-precipitation assay were consistent with those of the Annexin V assay. The percentage of apoptotic cells in the TF, DHA, DHA in combination with TF, and the control group were 8.75 ± 2.9%, 52.5 ± 5.7%, 68.6 ± 4.78%, and 6.15 ± 2.8%, respectively.
Many research groups have reported that artemisinin and its derivatives can inhibit the growth of cells associated with melanoma, breast, lung, liver, and central nervous system cancers, including some anticancer drug-resistant cell lines [1215161718]. The results of this study indicated that DHA treatment in combination with holotransferrin destroys brain cancer cells more effectively than treatment with only DHA. Decreased viability of incubated C6 cells was observed at higher DHA and or TF concentrations, and the critical concentration was reduced when the exposure time was longer. The synergetic effect of holotransferrin in C6 cell death appears to depend on the exposure time, because the difference in cell viability between the DHA sample and DHA-TF sample was larger with longer exposure. The difference between the two groups was due to increased intracellular Fe2+ levels in C6 cells in response to transferrin pretreatment. Kim et al. reported that malignant brain cancers overexpress transferrin receptor, which binds transferrin and mediates more cellular iron uptake than normal brain cells [9]. Artemisinin and its derivatives carry an endoperoxide group and generate toxic free radicals in the presence of iron [13]. These toxic free radicals damage cellular macromolecules including DNA, leading to mitotic inhibition. In this study, the fraction of C6 cells in the sub-G0/G1 phase of DHA increased and DHA inhibited mitosis in C6 cells. The results of the Annexin V and caspase 3/7 immune-precipitation assay were consistent with those of the cell cycle assay.
In the present study, the efficacy of a combined treatment with DHA and TF toward inhibition of C6 cells proliferation in vitro was investigated. The results indicated that holotransferrin may synergistically enhance the anticancer effects of DHA via iron metabolism in brain cancer cells. Iron is essential for cell survival. Moreover, most cancer cells have larger numbers of iron-attracting transferrin receptors on their cell surface than normal cells [11]. Therefore, cancer cells contain more iron than normal cells. DHA reacts with a large amount of iron in cancer cells to form free radicals, acting as an effective anti-cancer drug. The findings in the present study demonstrate that DHA can inhibit mitosis of C6 cells in a time and dose-dependent manner in vitro and that holotransferrin may synergistically enhance the anti-cancer effects of DHA. These findings indicate that the DHA-TF combination may be useful in treating chemical drug-resistant brain cancer.
Figures and Tables
Fig. 1
Effects of dihydroartemisinin (DHA) and holotransferrin (TF) on proliferation of C6 cells.
DHA and TF efficacy in the inhibition of C6 cell proliferation were measured by MTT assay after 12 or 24 hrs from administration. It is shown that the inhibition efficacy of the combination of DHA and TF depends on exposure time as well as the concentration. P-value by paired t-test of DHA and DHA+TF.

Fig. 2
Effects of dihydroartemisinin (DHA) and holotransferrin (TF) on the cell cycle of C6 cells.
Analysis of cell cycle assay with PI labeling was accomplished to quantify the percentage of C6 cells by phases. DNA fragmentation in apoptotic cells translates into the fluorescence intensity in sub-G0/G1 phase, and it is lower than that of G0/G1 cells. P-value by t-test of DHA and DHA+TF.
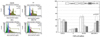
Fig. 3
Flow cytometry analysis of Annexin V in dihydroartemisinin (DHA) and/or holotransferrin (TF) treated C6 cells.
Because the decrement in cell proliferation came with the induction of apoptosis, we investigated DHA-induced proliferation inhibition of C6 cells. The percentage of apoptotic cells was determined by FITC-labeled annexin V and PI double-staining flow cytometry. P-value by t-test of DHA and DHA+TF.
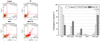
References
1. Huang L, Liu JF, Liu LX, Li DF, Zhang Y, Nui HZ, Song HY, Zhang CY. Antipyretic and anti-inflammatory effects of Artemisia annua L. Zhongguo Zhong Yao Za Zhi. 1993; 18:44–48. 63–64.
2. Juteau F, Masotti V, Bessière JM, Dherbomez M, Viano J. Antibacterial and antioxidant activities of Artemisia annua essential oil. Fitoterapia. 2002; 73:532–535.


3. Habib M, Waheed I. Evaluation of anti-nociceptive, anti-inflammatory and antipyretic activities of Artemisia scoparia hydromethanolic extract. J Ethnopharmacol. 2013; 145:18–24.


4. Sen R, Bandyopadhyay S, Dutta A, Mandal G, Ganguly S, Saha P, Chatterjee M. Artemisinin triggers induction of cell-cycle arrest and apoptosis in Leishmania donovani promastigotes. J Med Microbiol. 2007; 56:1213–1218.


5. Gao X, Luo Z, Xiang T, Wang K, Li J, Wang P. Dihydroartemisinin induces endoplasmic reticulum stress-mediated apoptosis in HepG2 human hepatoma cells. Tumori. 2011; 97:771–780.


6. Chaturvedi D, Goswami A, Saikia PP, Barua NC, Rao PG. Artemisinin and its derivatives: a novel class of anti-malarial and anti-cancer agents. Chem Soc Rev. 2010; 39:435–454.


7. Chertok B, Moffat BA, David AE, Yu F, Bergemann C, Ross BD, Yang VC. Iron oxide nanoparticles as a drug delivery vehicle for MRI monitored magnetic targeting of brain tumors. Biomaterials. 2008; 29:487–496.


8. Stupp R, Mason WP, van den Bent MJ, Weller M, Fisher B, Taphoorn MJ, Belanger K, Brandes AA, Marosi C, Bogdahn U, Curschmann J, Janzer RC, Ludwin SK, Gorlia T, Allgeier A, Lacombe D, Cairncross JG, Eisenhauer E, Mirimanoff RO. European Organisation for Research and Treatment of Cancer Brain Tumor and Radiotherapy Groups. National Cancer Institute of Canada Clinical Trials Group. Radiotherapy plus concomitant and adjuvant temozolomide for glioblastoma. N Engl J Med. 2005; 352:987–996.


9. Kim SJ, Kim MS, Lee JW, Lee CH, Yoo H, Shin SH, Park MJ, Lee SH. Dihydroartemisinin enhances radiosensitivity of human glioma cells in vitro. J Cancer Res Clin Oncol. 2006; 132:129–135.


10. Chen H, Sun B, Pan S, Jiang H, Sun X. Dihydroartemisinin inhibits growth of pancreatic cancer cells in vitro and in vivo. Anticancer Drugs. 2009; 20:131–140.


11. Wu ZP, Gao CW, Wu YG, Zhu QS, Yan Chen, Xin Liu, Chuen Liu. Inhibitive effect of artemether on tumor growth and angiogenesis in the rat C6 orthotopic brain gliomas model. Integr Cancer Ther. 2009; 8:88–92.


12. Lai H, Singh NP. Selective cancer cell cytotoxicity from exposure to dihydroartemisinin and holotransferrin. Cancer Lett. 1995; 91:41–46.


13. Singh NP, Lai HC. Artemisinin induces apoptosis in human cancer cells. Anticancer Res. 2004; 24:2277–2280.
14. van Agtmael MA, Eggelte TA, van Boxtel CJ. Artemisinin drugs in the treatment of malaria: from medicinal herb to registered medication. Trends Pharmacol Sci. 1999; 20:199–205.


15. Tan W, Lu J, Huang M, Li Y, Chen M, Wu G, Gong J, Zhong Z, Xu Z, Dang Y, Guo J, Chen X, Wang Y. Anti-cancer natural products isolated from Chinese medicinal herbs. Chin Med. 2011; 6:27–42.


16. Chen HH, Zhou HJ, Fang X. Inhibition of human cancer cell line growth and human umbilical vein endothelial cell angiogenesis by artemisinin derivatives in vitro. Pharmacol Res. 2003; 48:231–236.


17. Singh NP, Lai H. Selective toxicity of dihydroartemisinin and holotransferrin toward human breast cancer cells. Life Sci. 2001; 70:49–56.


18. Singh NP, Lai HC. Synergistic cytotoxicity of artemisinin and sodium butyrate on human cancer cells. Anticancer Res. 2005; 25 6B:4325–4331.