Abstract
BACKGROUND/OBJECTIVES
Resveratrol, a natural polyphenol, has multiple functions in cellular responses including apoptosis, survival, and differentiation. It also participates in the regulation of inflammatory response and oxidative stress. MicroRNA-Let-7A (miR-Let7A), known as a tumor suppressor miRNA, was recently reported to play a crucial role in both inflammation and apoptosis. Therefore, we examined involvement of miR-Let7A in the modulation of inflammation and cell survival/apoptosis regulated by resveratrol.
MATERIALS/METHODS
mRNA expression of pro-/anti-inflammatory cytokines and sirtuin 1 (SIRT1), and protein expression of apoptosis signal-regulating kinase 1 (ASK1), p-ASK1, and caspase-3 and cleaved caspase-3 were measured, and cell viability and Hoechst/PI staining for apoptosis were observed in Lipopolysaccharide (LPS)-stimulated human THP-1 macrophages with the treatment of resveratrol and/or miR-Let7A overexpression.
RESULTS
Pre-treatment with resveratrol (25-200 µM) resulted in significant recovery of the reduced cell viabilities under LPS-induced inflammatory condition and in markedly increased expression of miR-Let7A in non-stimulated or LPS-stimulated cells. Increased mRNA levels of tumor necrosis factor-α and interleukin (IL)-6 induced by LPS were significantly attenuated, and decreased levels of IL-10 and brain-derived neurotrophic factor were significantly restored by resveratrol and miR-Let7A overexpression, respectively, or in combination. Decreased expression of IL-4 mRNA by LPS stimulation was also significantly increased by miR-Let7A overexpression co-treated with resveratrol. In addition, decreased SIRT1 mRNA levels, and increased p-ASK1 levels and PI-positive cells by LPS stimulation were significantly restored by resveratrol and miR-Let7A overexpression, respectively, or in combination.
Resveratrol is a natural compound with multiple functions in cellular responses including apoptosis, survival, and differentiation, and acts in therapeutic roles against metabolic disorders including obesity and cardiovascular pathological processes [123]. It promotes expression of antioxidant genes and plays a role as a scavenger for reactive oxygen species (ROS) in aortic smooth muscle cells (SMCs) [456]. Resveratrol also suppresses the activity of pro-inflammatory cytokines/chemokines [7], inhibits the secretion of interleukin (IL)-1, interferon-γ, tumor necrosis factor (TNF)-α, IL-6, and monocyte chemoattractant protein-1 (MCP-1) [8910], blocks the migration of monocytes and suppresses foam cell formation by reducing ROS generation and MCP-1 expression [1011].
Recent studies have suggested an association of microRNAs (miRNAs) with inflammatory processes [121314] and demonstrated that resveratrol controls expression of several miRNAs (i.e. miR-21, miR-181B, miR-663, miR-155, and miR-34A) in the inflammatory response, thereby providing a beneficial effect on SMCs and monocytes in patients with coronary artery disease [1516]. Among the miRNAs, miRNA-Let-7A (miR-Let7A) was known to be involved in the inflammation process, particularly in atherosclerosis by participating in NF-κB and angiogenesis signaling [17181920], and it was also reported to play crucial roles in cellular apoptosis [17181920]. According to Song et al. [17], miR-Let7A significantly attenuated phosphorylation of apoptosis signal-regulating kinase 1 (p-ASK1) which causes apoptosis through non-transcription- or transcription-dependent mechanisms [21]. ASK1, a kinase of the mitogen-activated protein kinase kinase kinase (MAPKKK) family [22], participates in numerous cellular responses including apoptosis and cell survival [23]. It is activated in response to cellular stresses such as endoplasmic reticulum stress and oxidative stress [2425]. In addition, the SIRT gene was reported to enhance cellular longevity [26] and reduce the incidence of cardiovascular disease and diabetes [2728]. Of all the sirtuins, SIRT1 (sirtuin 1, also known as NAD-dependent deacetylase sirtuin-1 or mammalian silent information regulator-2) specifically deacetylates key histone residues related to the regulation of multiple non-histone protein targets including p53, FOXO1/3, PGC-1α, and NF-κB [29]. Several studies demonstrated that the activity of SIRT1 is athero-protective by blocking NF-κB in macrophages [3031] and could regulate numerous cellular responses including DNA repair, neurogenesis, mitochondrial biogenesis, glucose and insulin homeostasis, and cellular stress responses [30]. According to the studies, resveratrol has anti-atherogenic effects through SIRT1 activation [3233].
However, the role of resveratrol in association with specific miRNA, particularly miR-Let7A in macrophages, has not been fully studied. Therefore, we examined involvement of miR-Let7A in the modulation of inflammation and cell survival/apoptosis regulated by resveratrol.
Human monocyte leukemia cells (THP-1 cells) (ATCC, Manassas, VA, USA) were cultured at 37℃ with 5% CO2 in RPMI 1640 medium (Hyclone Laboratories, South Logan, UT, USA) supplemented with 10% (v/v) fetal bovine serum (Hyclone Laboratories), 2 mM of L-glutamine, and 100 units/ml of penicillin/streptomycin (Hyclone Laboratories). For differentiation to adherent THP-1 derived macrophages, cells were plated at 1×106 cells/plate with 5 ng/ml of phorbol 12-myristate 13-acetate (PMA, Sigma-Aldrich, St. Louis, MO, USA) for 48 hrs [34], followed by stimulation with 1 µg/ml of lipopolysaccharides (LPS) (Sigma-Aldrich) for 4 hrs. THP-1 cells were treated with resveratrol (Sigma-Aldrich) at various concentrations (25, 50, 100, and 200 µM). NQDI-1, an apoptosis signal-regulating kinase 1 (ASK1) inhibitor, was purchased from Tocris Bioscience (TOCRIS Bioscience, Bristol, UK). THP-1 cells were pretreated with ASK1 inhibitor (600 nM) for 2 hrs to inhibit ASK1 activation.
THP-1 cells (2×105 cells/mL) were seeded in 96-well plates for monitoring all experimental conditions, including resveratrol pre-treatment (3 hrs) and LPS treatment (4 hrs). Next, 100 µL of 3-(4, 5-dimethylthiazol-2-yl)-2, 5-diphenyltetrazolium bromide (MTT) (Sigma-Aldrich) solution (5 mg/mL in PBS) was added to each well. After incubation for 1 hr 30 min, medium was removed, and dimethyl sulfoxide (DMSO) was added to each well to solubilize the purple formazan product of the MTT reaction. The supernatant from each well was analyzed using a microplate reader at 570 nm. All experiments were repeated six times. Cell viability in medium of non-treated cells was considered 100%.
miRNA-Let7A (miR-Let7A) (Ambion, Austin, TX, USA) was applied according to the manufacturer's protocol. Two kinds of miRNA were used in this study; Let-7A mimic (cat#, 4464066; sassy ID, MC10050) and Let-7A inhibitor (cat#, 4464084; assay ID, MH 10050). The THP-1 cells were cultured for 2 days and treated with miR-Let-7A. The cells were then treated with resveratrol with or without Let-7A inhibitor for 48 hrs, followed by treatment with LPS (1 µg/ml) at 4 hrs before sampling.
For quantitative analysis of miR-Let7A, reverse transcription-PCR was first performed using the Taqman Micro RNA Reverse Transcription kit (Applied Biosystems, Waltham, MA, USA) according to the manufacturer's instructions with total RNA of 10 ng. PCR reactions were then performed according to the manufacturer's instructions to quantitate the expression levels of miRNA-Let7A using TaqMan Universal PCR Master Mix, No Amp Erase UNG, and Taqman microRNA assay (Applied Biosystems). PCR amplification was performed in ABI 7500 Real Time PCR (Bio Rad, Philadelphia, PA, USA) at 95℃ for 10 min, followed by 40 cycles of 95℃ for 15 sec and 60℃ for 60 sec. The PCR incubation profile was extended to 40 cycles for miR-Let7A. PCR reactions were performed in triplicate. MiR-Let7A expression was normalized to the expression of U6.
Quantitative real time-PCR (qPCR) was performed to examine the mRNA expression of TNF-α and IL-6 in THP-1 cells under LPS-induced inflammation conditions. Total cellular RNA was extracted from THP-1 cells using Trizol reagent (Invitrogen, Carlsbad, CA, USA) according to the manufacturer's instructions. Poly (A) was added using poly (A) polymerase (Ambion). One Step SYBR® Prime Script TM RT-PCR Kit II (Takara, Otsu, Shiga, Japan) was used for qPCR. PCR was performed using the following primers (5' to 3'); TNF-α (F): CAA GGG ACA AGG CTG CCC CG, (R): GCA GGG GCT CTT GAC GGC AG, IL-6 (F): AAC GAT GAT GCA CTT GCA GA, (R): CTC TGA AGG ACT CTG GCT TTG, β-actin (F): TCT GGC ACC ACA CCT TCT A, (R): AGG CAT ACA GGG ACA GCA C. Denaturing was performed at 95℃ for 3 min; 40 cycles of 95℃ for 20 sec; annealing at 60℃ for 20 sec, and extension at 72℃ for 20 sec. At each extension step at 72℃, fluorescence was detected at 585 nm. The expression of each factor was assessed using an ABI prism 7500 Real-Time PCR System (Life Technologies Corporation, CA, USA) and analyzed with comparative Ct quantification. β-actin was amplified as an internal control. The values were presented by relative quantity (RQ).
To examine the expression of brain-derived neurotrophic factor (BDNF), IL-10, IL-4, SIRT1 (sirtuin 1, also known as NAD-dependent deacetylase sirtuin-1 or mammalian silencing information regulator-2) in THP-1 cells, reverse transcription-PCR was performed using each primer. Briefly, samples were lysed with Trizol reagent (Invitrogen), and total RNA was extracted according to the manufacturer's protocol. PCR was performed using the following thermal cycling conditions: 10 min at 95℃; 40 cycles of denaturing at 95℃ for 15 sec, annealing at 63℃ for 30 sec, and elongation at 72℃ for 30 sec; final extension at 72℃ for 10 min; and holding at 4℃. PCR was performed using the following primers (5' to 3'): BDNF (F): TGC CGC AAA CAT GTC TAT GAG G, (R): GCT GTG ACC CAC TCG CTA ATA C, IL-10 (F): GCC AAG CCT TAT CGG AAA TG, (R): CAC CCA GGG AAT TCA AAT GC, IL-4 (F):AGG TTT CCT GCG TCA AGA, (R):TTC AAG GAT CGT CTG GAT GC, SIRT1 (F): TGT TTC CTG TGG GAT ACC TGA, (R): TGA AGA ATG GTC TTG GGT CTT T, β-actin (F): TCT GGC ACC ACA CCT TCT A, (R): AGG CAT ACA GGG ACA GCA C. PCR products were electrophoresed in 1.5% agarose gels and stained with ethidium bromide.
After treatment with LPS and miR-Let7A transfection, cells were washed rapidly with ice-cold PBS, scraped, and collected. THP-1 cell pellets were lysed with ice-cold RIPA buffer (Sigma-Aldrich, St. Louis, MO, USA), and the lysates were centrifuged at 13,000 rpm for 1 hr 30 min at 4℃ to produce whole-cell extracts. Protein content was quantified using the BCA method (Pierce, Rockford, IL, USA). Protein (35 µg) was separated on a 10% SDS-polyacrylamide gel and transferred onto a polyvinylidene difluoride membrane. After blocking with 5% BSA prepared in TBS/Tween (20 nM Tris (pH 7.2), 150 mM NaCl, 0.1% Tween 20) for 1 hr at room temperature, immunoblots were incubated overnight at 4℃ with primary antibodies specifically for detection of caspase-3 (1:1000, Santa Cruz Biotechnology, Santa Cruz, CA, USA), cleaved caspase-3 (1:1000, Santa Cruz Biotechnology), ASK1 (1:1000; Santa Cruz Biotechnology), phosphorylated ASK1 (p-ASK1; 1:1000; Santa Cruz Biotechnology), or β-actin (1:1000; Cell Signaling, Danvers, MA, USA). Blots were then incubated with horseradish peroxidase-linked anti-mouse or -rabbit IgG antibodies (Abcam, Cambridge, MA, USA) for 1 hr at room temperature. Enhanced chemiluminescence was performed by ECL (Pierce).
THP-1 cells were washed three times with PBS, and permeabilized for 15 min, followed by incubation with the primary antibodies overnight at 4℃. The following primary antibodies were used: anti-goat p-ASK1 (1:200, Santa Cruz Biotechnology). The primary antibody was then removed, and the cells were washed three times for 3 min with PBS. THP-1 cells were incubated with FITC-conjugated donkey anti-goat IgG (1:200, Jackson Immunoresearch, West Grove, PA, USA) for 1 hr 30 min at room temperature. THP-1 cells were washed again three times for 3 min with PBS, followed by counterstaining with 1 µg/ml of 4',6-diamidino-2-phenylindole (DAPI, 1:200, Invitrogen) for 10 min at room temperature. Imaging of THP-1 cells was performed using a Zeiss LSM 700 confocal microscope (Carl Zeiss, Thornwood, NY, USA)).
Hoechst 33258/propidium iodide (PI) staining was performed for apoptosis. Hoechst 33258 dye (Sigma aldrich) was added to the cell culture medium (2 µg/ml), followed by incubation of the samples at 37.5℃ for 30 min. PI (Sigma Aldrich) solution was added (2 µg/ml) before observation of cells using a Zeiss LSM 700 confocal microscope (Carl Zeiss). PI-positive cells were counted as dead cells.
Statistical analyses were performed using SPSS ver22 (IBM Corp., Armonk, NY, USA), and data were expressed as the mean ± SEM. The differences among the groups were determined by one-way analysis of variance (ANOVA) followed by Bonferroni multiple comparison tests. Each experiment included at least 3 replicates per condition. A P-value less than 0.05 was considered statistically significant.
In the MTT assay to examine THP-1 cell viability, THP-1 cell viabilities were significantly increased by pre-treatment with resveratrol (25 µM, 50 µM, 100 µM, and 200 µM) compared with normal (non-stimulated) and LPS-stimulated cells. The reduced cell viability under LPS induced inflammatory condition was markedly increased by pre-treatment with resveratrol (25 µM, 50 µM, 100 µM, and 200 µM) which were similarly recovered up to that of normal cells (Fig. 1). Based on the results, 25 µM resveratrol was used in all the following experiments.
TaqMan assay was performed to examine association of miR-Let7A expression with resveratrol. miR-Let7A expression in resveratrol treated THP macrophages was 7 times higher than that in normal (non-treated) and LPS-stimulated cells (all for P < 0.01) (Fig. 2). Cells stimulated with LPS showed slightly reduced miR-Let7A expression compared with the normal control, but without statistical significance. The reduced miR-Let7A levels in LPS-stimulated cells were significantly recovered, rather more increased by resveratrol treatment compared with those in the normal control (all for P < 0.05). On the other hand, the reduced miR-Let7A expression in LPS-stimulated cells appeared to be slightly recovered, and increased by the miR-Let7A overexpression, but without statistical significance.
Quantitative real time-PCR and reverse transcription PCR were performed to examine mRNA expression of cytokines regulated by resveratrol with miR-Let7A. The mRNA levels of TNF-α and IL-6 as pro-inflammatory cytokines in THP-1 macrophages were markedly increased by LSP stimulation compared with those in non-stimulated normal cells (P < 0.01 for both) (Fig. 3). Increased mRNA levels of TNF-α and IL-6 induced by LPS were significantly attenuated by treatment with resveratrol. Their increased levels were also attenuated by miR-Let7A overexpression separately or in co-treatment with resveratrol (Fig. 3). On the other hand, the mRNA levels of BDNF as the neurotrophic factor, and IL-4 and IL-10 as anti-inflammatory cytokines were evidently reduced by LPS stimulation, but the mRNA levels of BDNF and IL-10 were markedly increased by resveratrol treatment compared with those in non-stimulated normal cells (Fig. 4). Decreased mRNA levels of BDNF and I-10 by LPS stimulation were significantly increased by resveratrol treatment separately or in co-treatment with miR-Let7A overexpression. However, decreased mRNA levels of IL-4 by LPS stimulation were significantly increased only by the co-treatment of resveratrol and miR-Let7A overexpression (Fig. 4).
To examine the association of the inflammatory responses controlled by resveratrol and miR-Let7A overexpression with apoptosis, Hoechst/PI staining was performed with confocal microscopic analysis and caspase-3 pathways (caspase-3/cleaved caspase-3) were observed by Western blots (supplementary Fig. S1 and S2). In Fig. S1, many PI positive cells (apoptotic death cells, red dots) were observed upon stimulation by LPS, which were not observed in non-treated normal cells or resveratrol-only treated cells. In addition, PI positive cells observed under the LPS-stimulated condition were significantly decreased by treatment with resveratrol. On the other hand, in the LPS-stimulated condition, miR-Let7A overexpression-only or co-treated with resveratrol showed a much lower amount of PI positive cells compared with those treated with resveratrol only. As shown in Fig. S2, increased production of cleaved caspase-3 (the active form of apoptosis) by LPS-stimulation was attenuated by resveratrol treatment (upper panel) and by miR-Let7A overexpression (lower panel). In addition, LPS-induced production of cleaved caspase-3 was decreased by miR-Let7A overexpression co-treated with resveratrol.
Western blot and immunofluorescence analysis were performed to examine the role of resveratrol in association with miR-Let7A on p-ASK1, which is involved in cellular apoptosis and survival signaling. As shown in Fig. 5, phosphorylation of ASK1 in LPS-stimulated cells was significantly attenuated by miR-Let7A overexpression, and markedly attenuated by resveratrol treatment separately or in combination with miR-Let7A overexpression. As shown in Fig. 6, LPS-stimulated THP-1 macrophages showed more fluorescent intensity, indicating increased phosphorylation of ASK1 in the nucleus compared to normal THP-1 cells. LPS-induced phosphorylation of ASK1 was attenuated by both resveratrol treatment and miR-Let7A overexpression. Consequently, resveratrol may boost the suppressive effect of miR-Let7A on ASK1 phosphorylation.
Reverse transcription-PCR was performed to examine the role of resveratrol in association with miR-Let7A on SIRT1 expression which enhances cellular longevity. Resveratrol significantly increased mRNA levels of SIRT1 in THP-1 macrophages compared with those in the normal control (Fig. 7). On the other hand, the mRNA levels of SIRT1 in THP-1 cells stimulated by LPS were slightly reduced compared with those in the normal control. Reduced mRNA levels of SIRT1 in LPS-stimulated THP-1 cells were significantly recovered and increased by resveratrol treatment separately or in the co-treatment of miR-Let7A overexpression.
The aim of the current study was to examine involvement of miR-Let7A in the modulation of inflammation and cell survival/apoptosis regulated by resveratrol. Results of this study show that resveratrol markedly increased miR-Let7A expression in THP-1 macrophages under normal and inflammatory conditions, and that the regulatory effect of resveratrol on the inflammatory process and cellular apoptosis/survival was associated with miR-Let7A. It suggested for the first time that miR-Let7A may be involved in the inflammatory response and cell survival/apoptosis modulated by resveratrol in human THP-1 macrophages.
In this study, the mRNA expression of IL-4, IL-10, and BDNF was increased and that of TNF-α and IL-6 was attenuated by treatment with resveratrol in both the inflammatory condition and in the normal condition. Our previous study and several other studies reported on the beneficial effect of resveratrol in promoting expression of IL-10 and BDNF, and suppressing TNF-α expression in various cells (i.e. microglia, vascular endothelium, and vascular SMCs) as well as in hypoxic brain injury [353637]. IL-10, which has anti-inflammatory and immunosuppressive effects, was reported to inhibit the secretion of TNF-α, the pro-inflammatory cytokine in microglia [3839]. In addition, the expression of BDNF participating in the survival of neuronal cells [26] was reported to show association with NF-κB signaling, one of the main pathways for inflammatory response [37]. Interestingly, we found that miR-Let7A expression was markedly increased by the treatment of non-stimulated normal cells with resveratrol, and slightly reduced miR-Let7A expression in LPS-stimulated cells was also significantly recovered by resveratrol treatment, which showed a greater increase compared with that in the normal control. In particular, anti-inflammatory roles of miR-Let7A in the mRNA levels of TNF-α, IL-6, IL-4, IL-10, and BDNF were boosted by treatment with resveratrol under the inflammation condition. Based on the previous reports and current results, our study indicates that resveratrol attenuates the expression of pro-inflammatory cytokines and promotes the expression of anti-inflammatory cytokines and neurotrophic factor in LPS-stimulated macrophages, particularly involving miR-Let7A.
Our results also suggest the involvement of miR-Let7A in the modulation of apoptosis regulated by resveratrol under inflammatory condition. Hoechst/PI staining indicated that many PI positive cells observed in the LPS-stimulated condition were significantly decreased by treatment with resveratrol, and more dramatically decreased by miR-Let7A overexpression and/or co-treatment with resveratrol. In addition, increased production of cleaved caspase-3, the active form of apoptosis [41] by LPS-stimulation, was attenuated by treatment with resveratrol and miR-Let7A overexpression, respectively, or in combination. Therefore, we assumed that miR-Let7A may be involved in apoptosis, particularly in the casepase-3 dependent pathway, which is regulated by resveratrol. Based on the above result, we additionally investigated the role of resveratrol in association with miR-Let7A on p-ASK1 and SIRT1 mRNA expression, which are involved in cellular apoptosis and survival signaling [232425262728]. Phosphorylation of ASK1 in LPS-stimulated THP-1 cells was significantly attenuated by miR-Let7A overexpression or resveratrol treatment, and the activation of ASK1 was more significantly attenuated by both resveratrol treatment and miR-Let7A overexpression than only by miR-Let7A overexpression. From our data, we assumed that the activation of ASK1 would be controlled by miR-Let7A, which was boosted by resveratrol treatment. As stated above, the SIRT gene was reported to enhance cellular longevity [21] and reduce the incidence of cardiovascular disease and diabetes [2728]. In our study, resveratrol promoted SIRT1 expression in THP-1 cells, and boosted the beneficial role of miR-Let7A in SIRT1 expression under the LPS-induced inflammatory condition.
In this study, we measured only mRNA levels of pro- and anti-inflammatory cytokines which are regulated by resveratrol and/or miR-Let7A, not their protein levels. If we had measured both mRNA and proteins levels of cytokines, we would have more concrete results for the conclusion. Despite the limitation, this study shows for the first time that resveratrol may upregulate miR-Let7A expression, and the up-regulated miR-Let7A may be involved in the modulation of LPS-induced inflammatory response and cell survival/apoptosis which can be regulated by resveratrol. In the future, the manipulation between miRNAs and resveratrol should be further-studied to elucidate the understanding of the cellular protective mechanism in macrophages.
Figures and Tables
Fig. 1
Effect of resveratrol on cell viability of THP-1 macrophages under LPS induced inflammatory condition.
The cell viability of THP-1 in all groups was measured using the MTT assay. The cell viability in LPS (1 µg/ml) stimulation was approximately 60%, whereas the resveratrol treatment groups showed higher cell viability (over 120%) compared to the normal group. Under LPS stimulated inflammatory condition, the cell viability of resveratrol-pretreated THP-1 cells (in all concentrations) was increased by over 30 % compared with that of only LPS treated THP-1 cells. Data are expressed as mean ± SEM and each experiment included 6 repeats per condition. * P < 0.05, ** P < 0.001 compared with normal control; φ P < 0.05 compared with LPS only stimulated cells
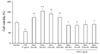
Fig. 2
Effect of resveratrol on miR-Let-7A expression in THP-1 macrophages under LPS induced inflammatory condition.
Expression of miR-Let7A was measured in all groups using Tagman real time-PCR. Data are expressed as mean ± SEM and each experiment included 3 repeats per condition. * P < 0.05, ** P < 0.001 compared with normal control; φP < 0.05 compared with LPS only stimulated cells
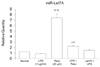
Fig. 3
Resveratrol modulates mRNA expression of pro-inflammatory cytokines in cooperation with miR-Let7A.
The mRNA levels of TNF-α and IL-6 in THP-1 cells were measured using quantitative real time PCR. Data are expressed as mean ± SEM and each experiment included 3 repeats per condition. * P < 0.05, ** P < 0.001 compared with normal control; φ P < 0.05 compared with LPS only stimulated cells; φφ P < 0.05 compared with LPS stimulated and miR Let7A overexpressed cells; Normal: non-treated control cells, Let7A (miR-Let7A mimic): Let7A overexpression, Resv: resveratrol (25 µM), LPS: lipopolysaccharide (1 µg/ml)
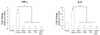
Fig. 4
Resveratrol modulates mRNA expression of BDNF and anti-inflammatory cytokines in cooperation with miR-Let7A.
The mRNA levels of BDNF, IL-4, and IL-10 in THP-1 cells were measured using reverse transcription PCR. Data are expressed as mean ± SEM and each experiment included 3 repeats per condition. * P < 0.05, ** P < 0.001 compared with normal control; φ P < 0.05 compared with LPS only stimulated cells; φφ P < 0.05 compared with LPS stimulated and miR Let7A overexpressed cells; Normal: non-treated control cells, Let7A (miR-Let7A mimic): Let7A overexpression, Resv: resveratrol (25 µM), LPS: lipopolysaccharide (1 µg/ml)
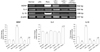
Fig. 5
Resveratrol attenuates the activation of ASK1 in cooperation with miR-Let7A.
Western blot analysis was performed for identification of apoptotic signaling in macrophages by resveratrol co-treated with or without miR-Let7A. Data were expressed as the mean ± SEM. and each experiment included 3 repeats per condition. φ P < 0.05 compared with LPS only stimulated cells.; Normal: non-treated cells, Let7A (miR-Let7A mimic): Let7A overexpression, Resv: resveratrol (25 µM), LPS: lipopolysaccharide (1 µg/ml)
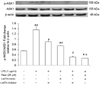
Fig. 6
Inhibitory effect of resveratrol in cooperation with miR-Let7A on the activation of ASK1 in THP-1macrophages.
Immunofluorescence staining was performed to check the activation of ASK1 (p-ASK1). Confocal microscopy analysis was performed to visualize the activation of ASK1 (p-ASK1). THP-1 cells were pretreated with resveratrol or/and Let7A mimic separately or following stimulation with LPS. p-ASK1 is represented by green staining, nuclear DNA is indicated by DAPI staining (blue color), and the combined images are presented; Normal: non-treated cells, Let7A (miR-Let7A mimic): Let7A overexpression, Resv: resveratrol (25 µM), LPS: lipopolysaccharide (1 µg/ml)
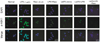
Fig. 7
Resveratrol stimulates SIRT1 pathways in cooperation with miR-Let7A.
The mRNA levels of SIRT1 in THP-1 cells were measured using reverse transcription PCR. Data are expressed as mean ± SEM and each experiment included 3 repeats per conditions. * P < 0.05, ** P < 0.001 compared with normal control; φ P < 0.05 compared with LPS only stimulated cells; φφ P < 0.05 compared with LPS stimulated and miR Let7A overexpressed cells; Normal: non-treated cells, Let7A (miR-Let7A mimic): Let7A overexpression, Resv: resveratrol (25 µM), LPS: lipopolysaccharide (1 µg/ml)
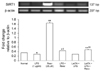
References
1. Ghiringhelli F, Rebe C, Hichami A, Delmas D. Immunomodulation and anti-inflammatory roles of polyphenols as anticancer agents. Anticancer Agents Med Chem. 2012; 12:852–873.


2. Zhang XH, Huang B, Choi SK, Seo JS. Anti-obesity effect of resveratrol-amplified grape skin extracts on 3T3-L1 adipocytes differentiation. Nutr Res Pract. 2012; 6:286–293.


3. Kang NE, Ha AW, Kim JY, Kim WK. Resveratrol inhibits the protein expression of transcription factors related adipocyte differentiation and the activity of matrix metalloproteinase in mouse fibroblast 3T3-L1 preadipocytes. Nutr Res Pract. 2012; 6:499–504.


4. Floreani M, Napoli E, Quintieri L, Palatini P. Oral administration of trans-resveratrol to guinea pigs increases cardiac DT-diaphorase and catalase activities, and protects isolated atria from menadione toxicity. Life Sci. 2003; 72:2741–2750.


5. Leonard SS, Xia C, Jiang BH, Stinefelt B, Klandorf H, Harris GK, Shi X. Resveratrol scavenges reactive oxygen species and effects radical-induced cellular responses. Biochem Biophys Res Commun. 2003; 309:1017–1026.


6. Li Y, Cao Z, Zhu H. Upregulation of endogenous antioxidants and phase 2 enzymes by the red wine polyphenol, resveratrol in cultured aortic smooth muscle cells leads to cytoprotection against oxidative and electrophilic stress. Pharmacol Res. 2006; 53:6–15.


7. Leiro J, Alvarez E, Arranz JA, Laguna R, Uriarte E, Orallo F. Effects of cis-resveratrol on inflammatory murine macrophages: antioxidant activity and down-regulation of inflammatory genes. J Leukoc Biol. 2004; 75:1156–1165.


8. Wang MJ, Huang HM, Hsieh SJ, Jeng KC, Kuo JS. Resveratrol inhibits interleukin-6 production in cortical mixed glial cells under hypoxia/hypoglycemia followed by reoxygenation. J Neuroimmunol. 2001; 112:28–34.


9. Zhu J, Yong W, Wu X, Yu Y, Lv J, Liu C, Mao X, Zhu Y, Xu K, Han X, Liu C. Anti-inflammatory effect of resveratrol on TNF-alpha-induced MCP-1 expression in adipocytes. Biochem Biophys Res Commun. 2008; 369:471–477.


10. Bruner-Tran KL, Osteen KG, Taylor HS, Sokalska A, Haines K, Duleba AJ. Resveratrol inhibits development of experimental endometriosis in vivo and reduces endometrial stromal cell invasiveness in vitro. Biol Reprod. 2011; 84:106–112.


11. Park DW, Baek K, Kim JR, Lee JJ, Ryu SH, Chin BR, Baek SH. Resveratrol inhibits foam cell formation via NADPH oxidase 1-mediated reactive oxygen species and monocyte chemotactic protein-1. Exp Mol Med. 2009; 41:171–179.


12. Kotsinas A, Sigala F, Garbis SD, Galyfos G, Filis K, Vougas K, Papalampros A, Johnson EE, Chronopoulos E, Georgakilas AG, Gorgoulis VG. MicroRNAs determining inflammation as novel biomarkers and potential therapeutic targets. Curr Med Chem. 2015; 22:2666–2679.


13. Hartmann P, Schober A, Weber C. Chemokines and microRNAs in atherosclerosis. Cell Mol Life Sci. 2015; 72:3253–3266.


14. Yang Y, Yang L, Liang X, Zhu G. MicroRNA-155 promotes atherosclerosis inflammation via targeting SOCS1. Cell Physiol Biochem. 2015; 36:1371–1381.


15. Tili E, Michaille JJ, Adair B, Alder H, Limagne E, Taccioli C, Ferracin M, Delmas D, Latruffe N, Croce CM. Resveratrol decreases the levels of miR-155 by upregulating miR-663, a microRNA targeting JunB and JunD. Carcinogenesis. 2010; 31:1561–1566.


16. Tomé-Carneiro J, Larrosa M, Yáñez-Gascón MJ, Dávalos A, Gil-Zamorano J, Gonzálvez M, García-Almagro FJ, Ruiz Ros JA, Tomás-Barberán FA, Espín JC, García-Conesa MT. One-year supplementation with a grape extract containing resveratrol modulates inflammatory-related microRNAs and cytokines expression in peripheral blood mononuclear cells of type 2 diabetes and hypertensive patients with coronary artery disease. Pharmacol Res. 2013; 72:69–82.


17. Song J, Lee JE. ASK1 modulates the expression of microRNA Let7A in microglia under high glucose in vitro condition. Front Cell Neurosci. 2015; 9:198.


18. Song J, Oh Y, Lee JE. miR-Let7A modulates autophagy induction in LPS-activated microglia. Exp Neurobiol. 2015; 24:117–125.


19. Kumar M, Sahu SK, Kumar R, Subuddhi A, Maji RK, Jana K, Gupta P, Raffetseder J, Lerm M, Ghosh Z, van Loo G, Beyaert R, Gupta UD, Kundu M, Basu J. MicroRNA let-7 modulates the immune response to Mycobacterium tuberculosis infection via control of A20, an inhibitor of the NF-kappaB pathway. Cell Host Microbe. 2015; 17:345–356.


20. Hulsmans M, Holvoet P. MicroRNA-containing microvesicles regulating inflammation in association with atherosclerotic disease. Cardiovasc Res. 2013; 100:7–18.


21. Tobiume K, Matsuzawa A, Takahashi T, Nishitoh H, Morita K, Takeda K, Minowa O, Miyazono K, Noda T, Ichijo H. ASK1 is required for sustained activations of JNK/p38 MAP kinases and apoptosis. EMBO Rep. 2001; 2:222–228.


22. Ichijo H, Nishida E, Irie K, ten Dijke P, Saitoh M, Moriguchi T, Takagi M, Matsumoto K, Miyazono K, Gotoh Y. Induction of apoptosis by ASK1, a mammalian MAPKKK that activates SAPK/JNK and p38 signaling pathways. Science. 1997; 275:90–94.


23. Takeda K, Hatai T, Hamazaki TS, Nishitoh H, Saitoh M, Ichijo H. Apoptosis signal-regulating kinase 1 (ASK1) induces neuronal differentiation and survival of PC12 cells. J Biol Chem. 2000; 275:9805–9813.


24. Goldman EH, Chen L, Fu H. Activation of apoptosis signal-regulating kinase 1 by reactive oxygen species through dephosphorylation at serine 967 and 14-3-3 dissociation. J Biol Chem. 2004; 279:10442–10449.


25. Hwang JR, Zhang C, Patterson C. C-terminus of heat shock protein 70-interacting protein facilitates degradation of apoptosis signal-regulating kinase 1 and inhibits apoptosis signal-regulating kinase 1-dependent apoptosis. Cell Stress Chaperones. 2005; 10:147–156.


26. Ralser M, Michel S, Breitenbach M. Sirtuins as regulators of the yeast metabolic network. Front Pharmacol. 2012; 3:32.


27. Bordone L, Guarente L. Calorie restriction, SIRT1 and metabolism: understanding longevity. Nat Rev Mol Cell Biol. 2005; 6:298–305.


28. Haigis MC, Guarente LP. Mammalian sirtuins--emerging roles in physiology, aging, and calorie restriction. Genes Dev. 2006; 20:2913–2921.


29. Morris BJ. Seven sirtuins for seven deadly diseases of aging. Free Radic Biol Med. 2013; 56:133–171.
30. Stein S, Lohmann C, Schäfer N, Hofmann J, Rohrer L, Besler C, Rothgiesser KM, Becher B, Hottiger MO, Borén J, McBurney MW, Landmesser U, Lüscher TF, Matter CM. SIRT1 decreases Lox-1-mediated foam cell formation in atherogenesis. Eur Heart J. 2010; 31:2301–2309.


31. Stein S, Schäfer N, Breitenstein A, Besler C, Winnik S, Lohmann C, Heinrich K, Brokopp CE, Handschin C, Landmesser U, Tanner FC, Lüscher TF, Matter CM. SIRT1 reduces endothelial activation without affecting vascular function in ApoE-/- mice. Aging (Albany, NY). 2010; 2:353–360.


32. Kulkarni SS, Cantó C. The molecular targets of resveratrol. Biochim Biophys Acta. 2015; 1852:1114–1123.


33. Baur JA, Sinclair DA. Therapeutic potential of resveratrol: the in vivo evidence. Nat Rev Drug Discov. 2006; 5:493–506.


34. Park EK, Jung HS, Yang HI, Yoo MC, Kim C, Kim KS. Optimized THP-1 differentiation is required for the detection of responses to weak stimuli. Inflamm Res. 2007; 56:45–50.


35. Bachis A, Colangelo AM, Vicini S, Doe PP, De Bernardi MA, Brooker G, Mocchetti I. Interleukin-10 prevents glutamate-mediated cerebellar granule cell death by blocking caspase-3-like activity. J Neurosci. 2001; 21:3104–3112.


36. Lee B, Moon SK. Resveratrol inhibits TNF-alpha-induced proliferation and matrix metalloproteinase expression in human vascular smooth muscle cells. J Nutr. 2005; 135:2767–2773.


37. Song J, Cheon SY, Jung W, Lee WT, Lee JE. Resveratrol induces the expression of interleukin-10 and brain-derived neurotrophic factor in BV2 microglia under hypoxia. Int J Mol Sci. 2014; 15:15512–15529.


38. Pestka S, Krause CD, Sarkar D, Walter MR, Shi Y, Fisher PB. Interleukin-10 and related cytokines and receptors. Annu Rev Immunol. 2004; 22:929–979.


39. Sawada M, Suzumura A, Hosoya H, Marunouchi T, Nagatsu T. Interleukin-10 inhibits both production of cytokines and expression of cytokine receptors in microglia. J Neurochem. 1999; 72:1466–1471.

