Abstract
The leukocyte recruitment and transmigration across the endothelial barrier into the vessel wall are crucial steps in atherosclerosis. Leukocyte trafficking on the endothelium is elicited by induction of endothelial adhesion molecules, and its transmigration is mediated by degradation of basement membrane proteins through enzymatic activity of matrix metalloproteinases (MMP). The current study investigated whether resveratrol, a polyphenol present in grapes and red wine, was capable of inhibiting leukocyte adhesion to tumor necrosis factor (TNF)-α-activated endothelium. It was found that resveratrol inhibited the TNF-α-activated endothelial expression of vascular cell adhesion molecule-1 in a dose-dependent manner. In addition, resveratrol hampered THP-1 monocyte adhesion to activated endothelial cells. This study further examined whether resveratrol interfered with transendothelial migration of leukocytes. The MMP-2 gelatinolytic activity of endothelial cells was enhanced by TNF-α, which was attenuated by an addition of ≥25 µM resveratrol. In addition, 25 µM resveratrol mitigated the MMP-9 activity of THP-1 cells, followed by a marked inhibition of transendothelial migration. These results demonstrated that resveratrol suppressed monocyte adhesion and migration induced by TNF-α through modulating expression of adhesion molecules and gelatinolytic activity of MMP. These findings suggest that dietary resveratrol may be therapeutic agent for inhibiting leukocyte recruitment into the subendothelium during inflammatory atherosclerosis.
Circulating leukocytes adhere to endothelial cells and undergo transmigration through the vessel wall into the extracellular matrix. Leukocyte extravasation requires the secretion of matrix-degrading enzymes, matrix metalloproteinases (MMP), which allow leukocytes to penetrate through the basement membrane. MMP can be divided into four categories based on substrate preference: collagenases, gelatinases, stromelysins and membrane-associated MMP. MMP-2 and MMP-9 preferentially degrade denatured gelatin and native collagen type IV which are one among the main components of basal membranes. Transmigration and metastasis of macrophages and tumor cells require disruption of basement membrane, so that MMP-2 and MMP-9 have been suggested to play a critical role in such processes (Galis et al., 1994; Nagaoka & Hirota, 2000; Saarialho-Kere et al., 1993).
The vascular endothelial cells are an important target of pro-inflammatory cytokine TNF-α (Pober & Cotran, 1990; Ross, 1993), in which it modulates endothelial expression of many genes involved in cell adhesion, thrombosis and inflammatory responses leading to atherosclerosis (Krasinski et al., 2000). Adhesion molecules such as vascular cell adhesion molecule-1 (VCAM-1) and intercellular cell adhesion molecule-1 (ICAM-1) are shown to play an important role in the induction of inflammation (Berk et al., 2001; Nakashima et al., 1998). The VCAM-1 induction of is a common feature in inflammatory environments and occurs in the early development of atherosclerosis (Schonbeck et al., 2000).
Several epidemiological studies have shown that red wine consumption is inversely related to cardiovascular disease (Aviram M & Fuhrman B, 2002; Belleville, 2002). Subsequently, multiple experiments have been conducted to demonstrate the pathophysiological activities of various components present in red wine. Resveratrol, a polyphenol subsisting in multiple plants including grapes and mulberries, is thought to be as a potential protective component (Bertelli et al., 1995; Hao & He, 2004). There is growing evidence that resveratrol can prevent or delay the onset of various cancers, heart diseases, ischemic and chemically induced injuries, pathological inflammation and viral infections (Baur & Sinclair, 2006; Bradamante et al., 2004; Shankar et al., 2007). In a recent report (de Lange et al., 2007), red wine inhibited platelet functions through content of polyphenolic grape extract that stimulated the inhibitory receptor, platelet endothelial cell adhesion molecule-1 (PECAM-1), thereby attenuating platelet activation. In addition, resveratrol suppressed monocyte chemotactic protein-1 binding activity of THP-1 cells and its receptor CCR2 mRNA, indicating that these inhibitory effects of resveratrol on chemokine receptor binding and expression may contribute, in part, to its cardiovascular protective activity in vivo (Cullen et al., 2007).
On the basis of the literature evidence that resveratrol is a protective component preventing the onset of pathological inflammation, the present study assessed the anti-inflammatory activity of resveratrol with respect to VCAM-1 expression in TNF-α-exposed human umbilical vein endothelial cells (HUVEC). Furthermore, the leukocyte extravasation was investigated by measuring gelatinolytic activity of MMP and monocyte migration.
M199 medium chemicals, RPMI 1640 medium chemicals, Resveratrol and 3-(4, 5-dimetylthiazol-yl)-diphenyl tetrazolium bromide (MTT) were obtained from Sigma-Aldrich Co. (St. Louis, MO), as were all other reagents, unless specifically stated elsewhere. Collagenase was purchased from Worthington Biochemicals (Lakewood, NJ). Fetal bovine serum (FBS), penicillin-streptomycin, trypsin-EDTA, bovine brain extract, human epidermal growth factor and hydrocortisone were purchased from Cambrex Corporation (East Rutherford, NJ). Antibodies against human VCAM-1, human β-actin and human VCAM-1 were purchased from Santa Cruz Biotechnology (Santa Cruz, CA). Horseradish peroxidase-conjugated goat anti-rabbit IgG was provided as a secondary antibody by the Jackson ImmunoResearch Laboratory (West Grove, PA). Human monocytic leukemic cell line THP-1 was obtained from American Type Culture Collection (Rockville, MD). TNF-α was obtained from Roche Molecular Biochemicals (Mannheim, Germany).
HUVEC were isolated from umbilical cords using collagenase as described elsewhere (Choi et al., 2005). Cultures were maintained at 37℃ humidified atmospheres of 5% CO2 in air. Cells were cultured in 25 mM HEPES-buffered M199 containing 10% FBS, 2 mM glutamine, 100 U/mL penicillin, 100 µg/mL streptomycin supplemented with 0.75 mg/mL human epidermal growth factor and 0.075 mg/mL hydrocortisone. Cells were passaged at confluence and used within 10 passages. Endothelial cells were confirmed by their cobblestone morphology and uptake of fluorescent 1.1'-dioctadecyl-3,3,3',3'-tetramethylindocarbocyanine perchlorate-labeled acetylated LDL.
HUVEC were plated at 90-95% confluence in all experiments. In experiments for resveratrol dose responses to TNF-α-induced VCAM-1 expression and MMP-2 activity, cells were incubated with 1-50 µM resveratrol overnight and subsequently exposed to 10 ng/mL TNF-α for 6 h. It has been shown by using high-performance liquid chromatography that concentrations of trans-resveratrol in human plasma ranged 15.0-4,000 ng/mL with intra-assay variability of 1.9-3.7% and inter-assay variability of 2.5-4.0% (Zhu et al., 1999).
HUVEC were grown in 25 mM HEPES-buffered at a density of 1.0 × 105 cells on 4-well glass chamber slides. Human monocytic THP-1 cells were labeled with 5 µM calcein-AM (Molecular Probes Inc., Eugene, OR) in RPMI 1640 medium containing 10% FBS. Cells were pre-treated with 10-50 µM resveratrol overnight prior to the 6 h exposure to 10ng/mL TNF-α. In the co-culture models, the labeled THP-1 cells were seeded at a density of 5.0 × 105 cells onto near-confluent monolayer of HUVEC treated with the resveratrol and/or TNF-α and were incubated for 2 h. After the co-cultured cells were thoroughly washed, photograph images were obtained at 485 nm excitation and 538 nm emission using a SPOT II digital camera-attached fluorescence microscope with Spot II data acquisition software (Diagnostic Instrument, Livingston, Scotland).
For the VCAM-1 induction by TNF-α, whole cell extracts were prepared from resveratrol-treated and TNF-α-exposed HUVEC in 1 M Tris-HCl (pH 6.8) lysis buffer containing 10% SDS, 1% β-glycerophosphate, 0.1 M Na3VO4, 0.5 M NaF and protease inhibitor cocktail. Cell lysates with equal amounts of total protein were fractionated by electrophoresis on 8% SDS-PAGE gels and transferred onto a nitrocellulose membrane. Nonspecific binding was blocked by soaking the membrane in TBS-T buffer [0.5 M Tris-HCl (pH 7.5), 1.5 M NaCl, and 0.1% Tween 20] containing 5% nonfat dry milk for 3 h. The membrane was incubated for 3 h with a primary polyclonal rabbit antihuman VCAM-1 antibody after three washes with TBS-T buffer, the membrane was then incubated for 1 h with horseradish peroxidase-conjugated goat anti-rabbit IgG. The protein level of VCAM-1 was determined by using Supersignal West Pico chemiluminescence detection reagents (Pierce Biotech. Inc., Rockford, IL) and Konica X-ray film (Konica Co., Tokyo, Japan). Incubation with monoclonal mouse β-actin antibody was also performed for the comparative control.
Culture medium of HUVEC or THP-1 cells was mixed in the ratio of 5 to1 with sample buffer and electrophoresed in 10% SDS-PAGE containing 1 mg/mL gelatin. Gels were renaturated by exchanging SDS for 2.5% Triton X-100, followed by 20 h incubation at 37℃ in the activation buffer (50 mM Tris, pH 7.5, 10 mM CaCl2, 0.2 M NaCl and 0.05% Brij). Gels were subsequently stained with Coomassie blue staining solution (0.1% Coomassie R250; 45% MeOH; 2% acetic acid) for 1 h, followed by being destained with 30% MeOH and 10% acetic acid.
The experimental models for the monocyte migration employed 24-transwell inserts with pore sizes of 3 µm (Corning Incorp., Corning, NY). The lower surface of the insert filter was coated with 10 µL of 1 mg/mL gelatin solution and dried for 1 h. THP-1 cell suspensions of 106 cells/mL in serum-free RPMI 1640 were loaded in the upper compartment of the transwell chamber. The cells were treated with 25 µM resveratrol and exposed to 10 ng/mL TNF-α for 48 h. Transmigration was stopped by removing the transwell insert. The number of cells that had migrated to the lower compartment across the lower gelatin surface of the filter was counted microscopically. In addition, photography was obtained using a Motic AE30/31 inverted microscope (Motic Co., Xiamen, China).
The results are presented as mean ± SEM for each treatment group in each experiment. Statistical analyses were conducted using Statistical Analysis Systems statistical software package (SAS Institute Inc., Cary, NC). Significance was determined by one-way ANOVA followed by Duncan multiple range test for multiple comparisons. P values <0.05 were considered statistically significant.
The in vitro data for the THP-1 cell adhesion to HUVEC was shown in Fig. 1. A small number of monocytes were adhered to quiescent HUVEC free of 10 ng/mL TNF-α. There was heavy staining in TNF-α-alone-exposed HUVEC, indicative of a substantial increase in the THP-1 cell adherence to the activated HUVEC. However, adding resveratrol at non-toxic doses of 10-50 µM to TNF-α-exposed cells markedly attenuated the monocyte adherence in a dose-dependent manner (Fig. 1). It should be noted that ≥10 µM resveratrol was effective in inhibiting leukocyte adhesion to cytokine-activated endothelium.
Western blot analysis was performed to address that resveratrol blocked expression of VCAM-1 induced by TNF-α. Expression of VCAM-1 protein was greatly elevated in TNF-α-stimulated HUVEC over the quiescent cells (Fig. 2). When resveratrol was supplemented in the range between 10 and 50 µM, the enhanced expression of VCAM-1 dose-dependently declined. This data showed that ≥25 µM resveratrol diminished the TNF-α-stimulated expression of VCAM-1. These results imply that other adhesion molecules such as ICAM-1 and PECAM-1 may be involved in blocking the leukocyte trafficking.
When HUVEC were treated with 10 ng/mL TNF-α under serum-free conditions, the gelatin zymography revealed that the gelatinolytic activity of MMP-2 active form secreted in culture media within 24 h was substantially increased (Fig. 3A). In contrast, the treatment of TNF-α-exposed HUVEC with resveratrol mitigated the increased gelatinolytic activity of MMP-2 active form (69 and 68 kDa) only at its concentrations of ≥25 µM (Fig. 3A). It should be noted that the pro-form of MMP-2 (72 kDa) secretion was not influenced in presence of TNF-α and/or resveratrol. In addition, the enzyme activity of pro-form MMP-9 (92 kDa) secreted from TNF-α-added THP-1 cells was elevated, whereas 25 µM resveratrol markedly suppressed the MMP-9 activity (Fig. 3B). The active form of MMP-9 (82 kDa) was not apparent in TNF-α-exposed HUVEC.
Fig. 4 showed inhibitory effects of resveratrol on THP-1 transmigration into the vascular matrix tissue. The THP-1 cell migration across the gelatin layer mimicking basement membranes was markedly augmented within 48 h after an addition of TNF-α. On the contrary, the elevated monocyte transmigration was abolished by 25 µM resveratrol, indicating that resveratrol blunted cytokine-stimulated monocyte extravasation effectively through suppressing secretion of gelatinolytic MMP and subsequent degradation of vascular matrix.
This study attempted to test the hypothesis that resveratrol, a polyphenol found in red wine and peanuts, inhibits mononuclear leukocyte recruitment on the TNF-α-induced vascular endothelium. Four major findings were observed from this study. 1) Resveratrol at the non-toxic dose of ≥10 µM, nearly abolished THP-1 adhesion onto TNF-α-exposed cells. 2) Adding resveratrol in the dose range between 10 and 50 µM dose-dependently attenuated expression of VCAM-1 up-regulated by TNF-α. 3) When resveratrol at the doses of ≥25 µM was supplemented to TNF-α-exposed endothelial cells, the elevated gelatinolytic activity of MMP-2 was mitigated. In addition, the enhanced activity of MMP-9 was reduced in 25 µM resveratrol-treated THP-1 cells. 4) Resveratrol at the dose of 25 µM suppressed the transmigration of THP-1 monocytes exposed to TNF-α. These overall observations demonstrate that resveratrol has the potential capability to prevent the early events in atherosclerosis. The ability to block the TNF-α-induced monocyte trafficking and extravasation argues for the major target of action of resveratrol.
Numerous studies have focused on beneficial effects of polyphenols on cardiovascular functions including improvement of endothelial function, inhibition of angiogenesis and cell migration and proliferation in blood vessels (Choi et al., 2005; Katiyar, 2006; Middleton et al., 2000; Nijveldt et al., 2001). There is compelling evidence that the distinct structures of polyphenols appear to be partially responsible for their protective functions (Fukuhara et al., 2006; Hu et al., 2006; Teixeira et al., 2005). The mechanisms triggered by polyphenols with specific structures contribute to the vasoprotective, anti-angiogenic, anti-atherogenic, vasorelaxant and anti-hypertensive effects found in animals and in patients (Stoclet et al., 2004). In addition, the current evidence suggests that there is a plausible target for inhibiting atherosclerosis by grapes and/or its polyphenolic constituents through modulating novel pathways involved in the leukocyte extravasation.
Moderate consumption of red wine has been associated with beneficial effects on cardiovascular diseases, which is the phenomenon named as the French paradox (Belleville, 2002; Bertelli et al., 1995; Hao & He, 2004). Resveratrol has been regarded as the potential protective polyphenol present in red wine. In the present study resveratrol blunted monocyte adhesion onto the activated endothelial cells and its transmigration across a gelatin matrix barrier, indicating that resveratrol is capable to block the early process of atherosclerosis. It was reported that resveratrol might improve obesity-induced atherosclerosis by modulating the TNF-α-induced secretion changes of adipokine and adiponectin (Ahn et al., 2007). Additionally, resveratrol suppressed monocyte chemotactic protein-1 receptor CCR2 binding and expression of THP-1 cells responsible for atherosclerosis (Cullen et al., 2007). However, this study did not elucidate possible mechanisms by which resveratrol conferred the beneficial effects in endothelial responses to cytokines during inflammation. It was suggested that resveratrol be an antioxidant protecting endothelial cells from oxidized LDL-induced oxidative stress via direct ROS scavenging and inhibition of NADPH oxidase activity (Chow et al., 2007). In addition, this polyphenol inhibited IL-6-induced ICAM-1 gene expression, in part, by interfering with Rac-mediated pathways via the attenuation of STAT3 phosphorylation (Wung et al., 2005).
MMP-2 and MMP-9 preferentially degrade denatured gelatin and native collagen type IV, which are the main components of basal membranes. Accordingly, transmigration of macrophages requires disruption of basement membrane, and thus MMP-2 and MMP-9 have been implied to play a critical role in such processes (Galis et al., 1994; Nagaoka & Hirota, 2000; Saarialho-Kere et al., 1993). The present study showed that resveratrol interfered with monocyte transmigration concomitantly with dampening MMP secretion of endothelial cells and monocyes. A dose-related decrease of MMP-2 production after resveratrol treatment of human cultured glioblastoma cells revealed that resveratrol may influence the ECM remodeling occurring with tumor invasion (Gagliano et al., 2005). It was also shown that resveratrol inhibited phorbol myristate acetate-induced MMP-9 expression by inhibiting signal transduction of c-Jun N-terminal kinase and protein kinase Cδ (Woo et al., 2004). Considerable interest is increasing with regard to the use of dietary botanical supplements for chemopreventive and neuro- and cardiovascular-protective effects. Indeed, dietary botanicals such as green tea polyphenols and grape seed proanthocyanidins have been considered as MMP inhibitors and as cancer chemo-therapeutic agents (Katiyar, 2006).
In summary, the current study has demonstrated that resveratrol is capable of preventing early processes of atherosclerosis involving inducible VCAM-1 expression and MMP secretion. Resveratrol blocked monocyte adhesion onto the TNF-α-activated endothelium most likely via the down-regulation of VCAM-1 expression. In addition, this compound inhibited matrix-degrading MMP secretion from cytokine-activated endothelial cells and monocytes. This observation might have clinical implications for therapeutic strategies preventing and attenuating inflammatory diseases. Although definite mechanisms underlying the protection of resveratrol against early atherogenic process are not fully defined in this study, the VCAM-1 expression and MMP secretion may be a pivotal target of anti-atherogenic actions of resveratrol.
Figures and Tables
Fig. 1
Inhibitory dose response of THP-1 monocytes adhesion to resveratrol in TNF-α-activated HUVEC. HUVEC were pre-treated at 10-50 µM resveratrol overnight and then activated with 10 ng/mL TNF-α for 6 h. Endothelial cells were co-cultured with calcein AM-labeled THP-1 monocytes for 2 h. Microphotographs (3 independent experiments) were obtained using a fluorescence microscopy. Magnification ×200. The bar graphs (bottom panel) represent quantitative results obtained by using a Fluoroscan ELISA plate reader at λ=485 nm excitation and λ=538 nm emission. *p<0.05, relative to TNF-α-untreated control incubation. +p<0.05, relative to TNF-α-alone incubation.
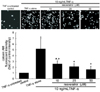
Fig. 2
Resveratrol inhibition of VCAM-1 induction in TNF-α-stimulated HUVEC. After culturing HUVEC with 10-50 µM resveratrol and 10 ng/mL TNF-α, cell extracts were subjected to 8% SDS-PAGE, followed by Western blot analysis with a VCAM-1 primary antibody. β-Actin protein was used as an internal control. The bar graphs (bottom panel) represent densitometric results (3 independent experiments). *p<0.05, relative to TNF-α-untreated control incubation. +p<0.05, relative to TNF-α-alone incubation. ++p<0.05, relative to incubation with 25 µM resveratrol.
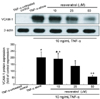
Fig. 3
Gelatinolytic activity of MMP-2 (A) and MMP-9 (B) in TNF-α-exposed HUVEC and THP-1 cells. HUVEC (for MMP-2) and THP-1 cells (for MMP-9) were with 25 µM resveratrol overnight prior to an addition of 10 ng/mL TNF-α and continuously incubated for 24 h. The collected culture media were subjected to electrophoresis on 10% SDS-PAGE co-polymerized by 0.1% gelatin as the substrate. Gelatinolytic activities were detected as unstained bands against the background of Coomassie blue-stained gelatin and gel photographs (3 separate experiments) were obtained.
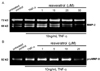
Fig. 4
Resveratrol inhibition of TNF-α-induced THP-1 monocyte transmigration. THP-1 cells were incubated by serum free-RPMI-1640 on the transwell chamber coated with 0.1% gelatin on its lower surface. Subsequently, cells were incubated for 48 h in the presence of 25 µM resveratrol and 10 ng/mL TNF-α. THP-1 cells migrated to the lower compartment of transwell chamber were microscopically photographed. The THP-1 cell migration ability was defined by the number of THP-1 cells migrated. *p<0.05, relative to TNF-α-untreated control incubation.
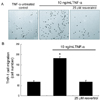
Notes
This study was supported by grant (R01-2006-000-10896-0) from the Korea Science & Engineering Foundation, a grant from the Korea Science & Engineering through the Silver Biotechnology Research Center at Hallym University, grant (10027174-2007-02) from the Ministry of Commerce, Industry and Energy, grant (s0707212-G0943230-10100011) from the Small and Medium Business Administration, and grants (KRF-2003-041-C20338 and Brain Korea 21) from Korea Research Foundation, Korea.
References
1. Ahn J, Lee H, Kim S, Ha T. Resveratrol inhibits TNF-α-induced changes of adipokines in 3T3-L1 adipocytes. Biochem Biophys Res Commun. 2007. 364:972–977.


2. Aviram M, Fuhrman B. Wine flavonoids protect against LDLoxidation and atherosclerosis. Ann NY Acad Sci. 2002. 957:146–161.
3. Baur JA, Sinclair DA. Therapeutic potential of resveratrol: the in vivo evidence. Nat Rev Drug Discov. 2006. 5:493–506.


4. Belleville J. The French paradox: possible involvement of ethanol in the protective effect against cardiovascular diseases. Nutrition. 2002. 18:173–177.


5. Berk BC, Abe JI, Min W, Surapisitchat H, Yan C. Endothelial atheroprotective and anti-inflammatory mechanisms. Ann N Y Acad Sci. 2001. 947:93–109.


6. Bertelli AA, Giovannini L, Giannessi D, Migliori M, Bernini W, Fregoni M, Bertelli A. Antiplatelet activity of synthetic and natural resveratrol in red wine. Int J Tissue React. 1995. 17:1–3.
7. Bradamante S, Barenghi L, Villa A. Cardiovascular protective effects of resveratrol. Cardiovasc Drug Rev. 2004. 22:169–188.


8. Choi YJ, Jeong YJ, Lee YJ, Kwon HM, Kang YH. (-) Epigallocatechin gallate and quercetin enhance survival signaling in response to oxidant-induced human endothelial apoptosis. J Nutr. 2005. 135:707–713.


9. Chow SE, Hshu YC, Wang JS, Chen JK. Resveratrol attenuates oxLDL-stimulated NADPH oxidase activity and protects endothelial cells from oxidative functional damages. J Appl Physiol. 2007. 102:1520–1527.


10. Cullen JP, Morrow D, Jin Y, von Offenberg Sweeney N, Sitzmann JV, Cahill PA, Redmond EM. Resveratrol inhibits expression and binding activity of the monocyte chemotactic protein-1 receptor, CCR2, on THP-1 monocytes. Atherosclerosis. 2007. 195:e125–e133.


11. de Lange DW, Verhoef S, Gorter G, Kraaijenhagen RJ, van de Wiel A, Akkerman JW.. Polyphenolic grape extract inhibits platelet activation through PECAM-1: an explanation for the French paradox. Alcohol Clin Exp Res. 2007. 31:1308–1314.


12. Fukuhara K, Nagakawa M, Nakanishi I, Ohkubo K, Imai K, Urano S, Fukuzumi S, Ozawa T, Ikota N, Mochizuki M, Miyata N, Okuda H. Structural basis for DNA-cleaving activity of resveratrol in the presence of Cu2+. Bioorg Med Chem. 2006. 14:1437–1443.


13. Gagliano N, Moscheni C, Torri C, Magnani I, Bertelli AA, Gioia M. Effect of resveratrol on matrix metalloproteinase-2 (MMP-2) and Secreted Protein Acidic and Rich in Cysteine (SPARC) on human cultured glioblastoma cells. Biomed Pharmacother. 2005. 59:359–364.


14. Galis ZS, Sukhova GK, Lark MW, Libby P. Increased expression of matrix metalloproteinases and matrix degrading activity in vulnerable regions of human atherosclerotic plaques. J Clin Invest. 1994. 94:2493–2503.


15. Hao HD, He LR. Mechanisms of cardiovascular protection by resveratrol. J Med Food. 2004. 7:290–298.


16. Hu J, Huang Y, Xiong M, Luo S, Chen Y, Li Y. The effects of natural flavonoids on lipoxygenase-mediated oxidation of compounds with a benzene ring structure-a new possible mechanism of flavonoid anti-chemical carcinogenesis and other toxicities. Int J Toxicol. 2006. 25:295–301.


17. Katiyar SK. Matrix metalloproteinases in cancer metastasis: molecular targets for prostate cancer prevention by green tea polyphenols and grape seed proanthocyanidins. Endocr Metab Immune Disord Drug Targets. 2006. 6:17–24.


18. Krasinski K, Spyridopoulos I, Kearney M, Losordo DW. In vivo blockade of tumor necrosis factor-alpha accelerates functional endothelial recovery after balloon angioplasty. Circulation. 2000. 104:1754–1756.


19. Middleton E Jr, Kandaswami C, Theoharides TC. The effects of plant flavonoids on mammalian cells: implications forinflammation heart disease and cancer. Pharmacol Rev. 2000. 52:673–751.
20. Nagaoka I, Hirota S. Increased expression of matrix metalloproteinase-9 in neutrophils in glycogen-induced peritoneal inflammation of guinea pigs. Inflamm Res. 2000. 49:55–62.


21. Nakashima Y, Raines EW, Plump AS, Breslow JL, Ross R. Upregulation of VCAM-1 and ICAM-1 at atherosclerosis-prone sites on the endothelium in the Apo-E-deficient mouse. Arterioscler Thromb Vasc Biol. 1998. 18:842–851.


22. Nijveldt RJ, van Nood E, van Hoorn DE, Boelens PG, van Norren K, van Leeuwen PA. Flavonoids: a review of probable mechanisms of action and potential applications. Am J Clin Nutr. 2001. 74:418–425.


23. Pober JS, Cotran RS. The role of endothelial cells in inflammation. Transplantation. 1990. 50:537–544.


24. Ross R. The pathogenesis of atherosclerosis: a perspective for the 1990s. Nature. 1993. 362:801–809.


25. Saarialho-Kere UK, Welgus HG, Parks WC. Distinct mechanisms regulate interstitial collagenase and 92-kDa gelatinase expression in human monocytic-like cells exposed to bacterial endotoxin. J Biol Chem. 1993. 268:17354–17361.


27. Shankar S, Singh G, Srivastava RK. Chemoprevention by resveratrol: molecular mechanisms and therapeutic potential. Front Biosci. 2007. 12:4839–4854.


28. Stoclet JC, Chataigneau T, Ndiaye M, Oak MH, El Bedoui J, Chataigneau M, Schini-Kerth VB. Vascular protection by dietary polyphenols. Eur J Pharmacol. 2004. 500:299–313.


29. Teixeira S, Siquet C, Alves C, Boal I, Marques MP, Borges F, Lima JL, Reis S. Structure-property studies on the antioxidant activity of flavonoids present in diet. Free Radic Biol Med. 2005. 39:1099–1108.


30. Woo JH, Lim JH, Kim YH, Suh SI, Min DS, Chang JS, Lee YH, Park JW, Kwon TK. Resveratrol inhibits phorbol myristate acetate-induced matrix metalloproteinase-9 expression by inhibiting JNK and PKC delta signal transduction. Oncogene. 2004. 23:1845–1853.

