Abstract
Preservation methods on the physiological and brewing technical characters in bottom and top brewing yeast strains were investigated. The preserved yeasts were reactivated after 24 months storage and grown up to stationary phase. The samples of filter paper storage indicated a higher cell growth and viability during propagation than those of nitrogen and lyophilization storage independent on propagation temperature. In addition, the filter paper storage demonstrated a faster absorption of free amino nitrogen and a highest level of higher aliphatic alcohols production during propagation than other preservation methods, which can be attributed to intensive cell growth during propagation. Moreover, the filter paper storage showed a faster accumulation for glycogen and trehalose during propagation, whereas, in particular, lyophilization storage noted a longer adaptation time regarding synthesis of glycogen and trehalose with delayed cell growth. In beer analysis, the filter paper storage formed an increased higher aliphatic alcohols than control. In conclusion, the preservation of filter paper affected positively on yeast growth, viability and beer quality independent on propagation temperature. In addition, in this study, it was obtained that the HICF and Helm-test can be involved as rapid methods for determination of flocculation capacity.
The preservation of brewing yeast strains is a basic aspect in yeast management, and the storage on slant agar is a simple and old method to preserve the brewing yeast. Thorne and Nohr (1963) described, for example, a phenomenon, at which some strong flocculating bottom yeasts were changed to weakly flocculating yeast due to often subculture. The lyophilization storage is also often described with regard to brewing yeast as a preservation method (Fischborn, 1997; Fischer et al., 2000; Harrison et al., 1996; Sips et al., 1998) at which the yeast may be preserved stabile for long time in spite of lower viability. Hill (1981) reported that yeast cells from the stationary phase are less sensitive than that of the exponential phase regarding physical damage, when the yeasts were preserved under a liquid nitrogen condition. Fischer and Rahn (2000) investigated the storage ability and characters of brewing yeast using the filter paper method over 20 months, and found a decrease of living cells. Flocculation of yeast cells and subsequent removal of the clumps from the medium is of considerable importance in beer brewing, governed by the competition between electrostatic repulsion (nonspecific interactions) and polysaccharide-protein bonds (specific interactions) (Costa & Ferreira, 1993; Dengis et al., 1997; Fontana et al., 1992; Van Hamersveld et al., 1998). For brewing yeast, flocculation of bottom fermenting strains was related to an increase in surfaced hydrophobicity which was attributed to surface proteins (Amory et al., 1988; Dengis & Rouxhet, 1997; Kamada & Murata, 1984; Rouxhet et al., 1994; Smit et al., 1992; Straver et al., 1994). Flotation of cell yeasts was also related to surface hydrophobicity (Romano et al., 1994; Straver et al., 1994; Tybussek et al., 1994). Stewart et al. (1995) isolated and characterized the cell wall proteins that mediate flocculation directly from the yeast cell extracts. Amory et al. (1988) reported that the effect of the culture conditions on the tendency of the cells to flocculate was related to the variation of the surface electrical properties. Floc formation is a result of effective collisions between cells (Van Hamersveld et al., 1998). Also, glycogen and trehalose are the major reserve carbohydrate in brewing yeasts (Quain & Tubb, 1982). Wackerbauer et al. (1997a, 1997b) reported that glycogen and trehalose had an influence on yeast viability and vitality. The objective of this study was to characterize the effect of different preservation methods on yeast physiology, flocculation capacity, and beer flavour in bottom and top brewing fermenting yeasts.
Five strains of Saccharomyces cerevisiae were used in this study. Strain Rh is a bottom strong flocculent brewing yeast. Strain 43P is a bottom weak flocculent brewing yeast. Strain Frank is a bottom powdery brewing yeast. Strain 160 is a top fermentation brewing yeast (called alt brewing yeast). Strain 127 is also a top fermentation brewing yeast (called wheat brewing yeast). All strains were obtained from the Brewing and Research and Teaching Institute (VLB) in Berlin.
All yeasts were inoculated into of 200 ml Erlenmeyer flask containing 50 ml of wort and incubated statically at 25℃ for 48 h.
Fifty ml of preculture were inoculated in a 10 l stirred conical glass flask (Schott Duran) that contained 5 l of sterile wort, and propagated with agitation at 100 rpm. The sterile wort contained total nitrogen 1018 ppm, and free amino nitrogen 186 ppm, zinc 0.10 ppm, and the content of fermentable extract was 12%.
Various propagated yeasts were inoculted into 5 l glass tube fermentor containing 2.5 l of 12% wort for fermentation tests and then fermented at 20℃ for 6 days. After each fermentation, the yeast was harvested and used again for the next fermentation test.
Viable cells were measured with a microscope after staining with Mg-ANS (1-aniline-8-naphthalene-sulphuric acid, Sigma, MD, USA). Viability was calculated by dividing the number of viable cells by the total number of cells, with results given as percentages.
Glycogen and trehalose concentations were determined by the method described by Quain & Tubb (1982) and Winkler et al. (1991). For the determination of the glycogen, the alkali- and acid-soluble fractions were treated with amyloglucosidase (EC.3.2.1.3.; Aspergillus niger, Sigma) and the released glucose estimated using an enzymatic colorimatic kit (Boehringer Mannheim). For the determination of trehalose, the yeast pellets extracted with water were treated with trehalase (EC. 3.2.1.28.; Sigma) and the amount of glucose released was estimated.
The test was based on modified method of Jibiki et al. (1997). Yeast cells were harvested by centrifugation and washed twice with 100 mM sodium acetate buffer (pH 4.2). Subsequently, the cells were resuspended in the same buffer to give a suspension with about 5% wet weight per volume. The concentration of the yeast corresponded to approximately 2.5 × 107 cells/ml. The hydrophobic colume was prepared in the following way: the Bio-Spin Disposable Chromatography Colume obtained from Bio-Rad Laboratories was used. The gel was Phenyl Sepharose CL-4B of Pharmacia which was packed in the colume to a volume of 0.25 ml and equilibrated with 100 mM sodium acetate buffer containing 1.0 M NaCl. Subsequently 0.1 ml of the cell suspension was applied to the colume and 3 ml of the buffer containing NaCl was then added. The eluent was collected, and the absorbance was measured at 660 nm in a spectrophotometer. All the preparation steps for yeast suspension were carried out at 4℃ to suppress the metabolic activity of yeast cells. The absorbance of 0.1 ml of the cell suspension diluted with 3 ml of the same buffer containing NaCl was also measured. The HICF value was defined by the proportion of retained cells and was calculated by using the following equation: HICF value (%)=100 (A applied-A eluent)/A applied, where A applied is the absorbance of 0.1 ml of the cell suspension diluted with 3 ml of the same buffer containing NaCl, and A eluent is the absorbance of the eluent.
The flocculation ability of different yeast cells was measured using a modified Helm-test as described by Sores et al. (1997). Yeast cells were harvested by centrifugation for 4 min at 4℃, washed twice in 250 mM EDTA solution, followed by washing the cells with NaCl solution (250 mM) at pH 2.0 and with NaCl solution (250 mM) at pH 4.5 at a final concentration near 1 × 108 cells/ml. Cell suspension (24 ml) in NaCl solution (250 mM), at pH 4.5, was placed in a 25 ml cylinder. The suspension was adjusted to 4 mM Ca2+ with the addition of CaCl2 solution (1.0 ml from a stock solution of 100 mM, at pH 4.5), and then agitated to promote flocculation. Unless stated otherwise, 18 inversions of the cylinder were used to promote flocculation. At defined periods of time, samples were taken (200-1000 µl) from a fixed position of the cylinder (the level corresponding to 20 ml), and dispersed in NaCl solution (250 mM) at pH 2.0. Cell concentration was determined by measuring the absorbance of the suspension at 620 nm. A calibration curve (number of cells versus absorbance) was previously constructed for each strain.
The standard analysis for wort and beer was determined according to the method reported by Pfenninger (1993). The original gravity, apparent extract and alcohol in beer were analyzed using the beer analyzer of Anton Paar with autosampler, (Modell SP-1). The extract was determined during the fermentation using Biegeschwinger (Modell Anton Paar, DPR Y), which determines the extract by measuring its density. The aromatic compounds were analysed GC using a Hewlet Packard 6890 gas chromatography fitted with a flame ionize (FID). Two ml of the solution was injected onto a carbowax column (50 m × 0.2 mm i.d.,1/4 inch o.d., Perkin-Elmer, USA) with 65℃ isothermally for 4 min, then increased by 9.5℃/min to 180℃, and held at 180℃ for 25 min. The injection temperature and detector temperature were 200℃. Nitrogen was used as the carrier gas (1 ml/min).
The wort bouillon and the filter paper chip were prepared. The petri dish was prepared and a dried medium was given on the bottom of petri dish. Thereafter the circular filter paper was placed on the petri dish. The desiccator was filled with a dried medium. A sterilization was performed for 5 h of petri dish and desiccator at 180℃. The wort bouillon was inoculated with yeast and afterwards was incubated for 48 h at 27℃ in incubator. The yeast suspension was transferred after determination of cell numbers to sterile centrifuge tube and harvested by centrifugation for 10 min at 3000 rpm. The cell concentration was determinated by direct microscopic counting. The condensed milk was broached and then taken out with a sterile injection. The milk was added into the centrifuge tube so much that sufficient cells could be suspended. The yeast-milk-suspension was taken out using a sterile disposable pipette and then 0.2 ml was given on each chip. The petri dish was transferred together with filter paper chip into the desiccator and then to the refrigerator. The complete drying took about 120 h. In order to reactive of the preserved yeast, the chip was placed in 10 ml sterile potassium chloride solution and the yeast was recovered from the filter paper.
The yeast grown at 27℃ was cultivated to the stationary phase. Then 1 ml suspension transferred into a sterile test tube and then centrifuged for 10 min at 6000 rpm. The pellet was resuspended in 1 ml of 15% low fat milk. Each 0.2 ml of yeast-milk-suspension was transferred into sterile glass ampulae. There after the ampulae were frozen at -20℃ for 1 h and then dried in the freeze drying equipment for 3 h, whereby the rest moisture of pellets can be reduced up to 3%. The ampulae were melted and then stored in a refrigerator. The reactivation of preserved yeast was performed in sterile potassium chloride solution or wort.
The yeast grown at 27℃ was cultivated to the stationary phase. Afterwards 0.3 ml glycerin was filled as protection medium in a sterile test tube and added 1 ml cell culture. In order to submerge in liquid nitrogen at -196℃ for 2 min, the test tube was allowed to stand for 20 min at room temperature. The following storage of the test tube was performed at -70℃ and the reactivation was accomplished in warm water at 30℃.
The yeasts were reactivated after 24 months storage and grown up stationary phase at 15 and 25℃ respectively. The results of yeast cell growth from preserved and non-preserved yeasts grown at 15℃ are shown in Fig.1A. The samples of control and filter paper storage showed its maximum of cell growth at 70 h during propagation, at which the cell number was about 160 and 180 million /ml, respectively. In contrast, the samples of nitrogen and lyophilization storage indicated its maximum of cell growth between 90 and 95 h with a lower cell number. A similar trend was also obtained from preserved and non-preserved yeasts grown at 25℃ (Fig. 1B). Moreover, the sample of filter paper storage presented a higher viability than other preservation methods (data not shown). From these results, it was pointed out that the preservation of filter paper affect positively on yeast growth and viability independent on propagation temperature.
To study the influence of preservation methods on absorption of free amino nitrogen by preserved yeast, it was investigated the pattern of absorption of free amino nitrogen content during propagation (Fig. 2A and 2B). Hereby it was observed that the speed of absorption of free amino nitrogen by preserved yeasts depended on preservation methods. As a shown in Fig. 2A, a faster reduction of free amino nitrogen was observed at samples of control and filter paper storage than at the samples of nitrogen and lyophilization storage. It seemed that the higher cell growth during propagation resulted in a faster absorption of free amino nitrogen by yeast (Dengis & Rouxhet, 1997; Rouxhet, et al., 1994; Straver, et al., 1994). However, final concentration was, among the samples, almost the same. In contrast, the difference of absorption of free amino nitrogen by yeasts grown at 25℃ was not found among the samples (Fig. 2B).
The formation of higher alcohols during propagation using preserved yeasts grown at 15℃ (Fig. 3A) and 25℃ (Fig. 3B) showed different results. At low growth temperature (Fig. 3A), the storage differed with regard to quantity and speed of formation among the samples. The filter paper storage showed the highest concentration of higher alcohol, which can be attributed to strong cell growth during propagation (Kamada & Murata, 1984; Rouxhet et al., 1994; Smit et al., 1992; Straver et al., 1994). At high growth temperature (Fig. 3B), in contrast, no significant differences were found among the samples in terms of higher alcohols. Remarkably, the sample of lyophilization storage accumulated shortly the higher alcohols in spite of low cell growth.
The development of glycogen and trehalose concentration was described during propagation at 15℃ (Fig. 4A and 4B). In particular, the speed of synthesis corresponded to speed of cell growth. The samples of control and filter paper storage accumulated faster regarding both metabolite than those of nitrogen and lyophilization storage. Hereby it was also observed that the accumulation of trehalose was considerably delayed at all samples. With beginning of flocculation onset, in most instances, it appeared the degradation of these reserve carbohydrates due to beginning nutrient deficiency and utilization of cell own reserve carbohydrates (Wackerbauer, 1982). Only the lyophilization storage indicated a delayed accumulation for glycogen and trehalose, and assembled in the end of propagation (Romano et al., 1994; Straver et al., 1994; Tybussek et al., 1994). In conclusion, it was obviously determined that the nitrogen and lyophilization storage noted a longer adaptation time with delayed cell growth than control and filter paper storage.
In order to determine the effect of various preservation methods on fermentation ability and beer quality, the most important results were summarized (Fig. 5). Regarding attenuation degree of finished beer, no differences were found among the samples in the 1st cycle; however, the control beer fermented more intensive on average in the 2nd cycle than other samples. The sample of lyophilization storage also gave here the worst performance (Fig. 5A). Interesting was that there were noticeable differences at the higher aliphatic alcohols, especially, in the 1st cycle (Fig. 5B), at which all samples preserved, in particular, the filter paper storage formed an enormous increase of higher aliphatic alcohols. The results of reduction in free amino nitrogen content from wort to beer were presented in Fig. 5C. Thereby it was observed in the 1st cycle that the yeasts had a higher demand of free amino nitrogen at higher vitality, concurrently, must synthesize more amino acids, which could consequently lead to an increase of higher aliphatic alcohols. The absorption of free amino nitrogen from nitrogen and lyophilization storage was lower at same fermentation ability than that of control, but the concentration of higher aliphatic alcohols formed was higher (Costa & Ferreira, 1993; Dengis et al., 1997; Fontana et al., 1992; Van Hamersveld et al., 1998). Furthermore, the filter paper storage still noted an increased demand of free amino nitrogen despite falling fermentation power in the 2nd cycle.
To determine the exact change of flocculation behavior during fermentation at different preservation methods, the flocculation capacity was determined using HICF-test (Fig. 6). It was shown the HICF-value of pitching yeast Rh and 43P over 6 cycles, in which the lyophilization storage was determined only in the 4th cycle. Clearly, a difference was obtained among the yeast strains. Thus, the brewing yeast Rh flocculated intensively during fermentation, which led to a higher cell surface hydrophobicity. Both samples of lyophilization storage at yeast Rh and 43P respectively showed an increased flocculation capacity during fermentation (Dengis et al., 1995; Dengis et al., 1997; Fontana et al., 1992; Van Hamersveld et al., 1998). This could clearly be proven by increased HICF-value. The increase of flocculation intensity corresponded to steady increase of HICF-values from 1st to 4th cycle in yeast strain 43P, but no relationship was found between preserved and non-preserved yeast strains in terms of flocculation rate and HICF-value (Fig. 6A and 6B). The powder yeast strain Frank noted the lowest HICF-values over 6 cycles, in contrast, the yeast strain 160 showed HICF-value of over 90% (Fig. 6C). The top fermenting yeast strain 127 also indicated the higher hydrophobicity (data not shown). It is likely that an increased protein content of cell wall resulted in a higher hydrophobicity in top fermenting yeast strains which was the same as the results of Dengis and Rouxhet (1997).
In this study, it was described that several typical results of the Helm-test to examine flocculation capacity (Fig. 7). The yeast which was taken out from the exponential phase of the propagator did not flocculate for control, filter paper, and lyophilization storage using the Helm-test after the 1st cycle, although the yeasts noted a high flocculation behavior during fermentation. After the 2nd cycle, the results of the Helm-test confirmed the results of fermentation. Thus, a high flocculation capacity of the yeast cells was recorded, at which the samples of control and filter paper storage showed an intensive flocculation capacity than those of nitrogen and lyophilization storage. No flocculation capacity was seen in powder yeast and top fermenting yeast using the Helm-test. Both methods, the HICF and the Helm-test, can be useful as a rapid method for prediction of flocculation capacity in some yeast strains.
Figures and Tables
Fig. 1
Yeast cell growth during propagation using preserved yeasts grown at 15℃ (A) and 25℃ (B) respectively in a bottom brewing yeast Rh
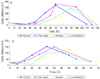
Fig. 2
Change of free amino nitrogen content during propagation using preserved yeasts grown at 15℃ (A) and 25℃ (B) respectively in a bottom brewing yeast Rh
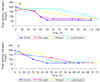
Fig. 3
Change of higher alcohols content during propagation using preserved yeasts grown at 15℃ (A) and 25℃ (B) respectively in a bottom brewing yeast Rh
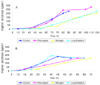
Fig. 4
Change of glycogen (A) and trehalose (B) content during propagation using preserved yeasts in a bottom brewing yeast Rh
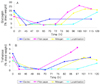
Fig. 5
Change of attenuation degree (A), higher alcohols (B), and differences of free amino nitrogen between wort and beer (C) over two cycles on average in a bottom brewing yeast Rh
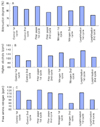
References
1. Amory DE, Rouxhet PG, Dufour JP. Flocculence of brewery yeasts and their surface properties: chemical composition, electrostatic charge and hydrophobicity. J Inst Brew. 1988. 94:79–84.


2. Costa MJ, Ferreira PMS. Cerevisiae flocculation: identification of specific cell wall proteins. European Brewery Convention Congress. 1993. Oslo: 283–290.
3. Dengis PB, Nelissen LR, Rouxhet PG. Mechanisms of yeast flocculation: comparison of top and bottom fermenting strains. Appl Environ Microbiol. 1995. 61:718–728.


4. Dengis PB, Rouxhet PG. Surface properties of top and bottom fermenting yeast. Yeast. 1997. 13:931–943.


5. Dengis PB, Rouxhet PG. Flocculation mechanisms of top and bottom fermenting brewing yeast. J Inst Brew. 1997. 103:257–261.


6. Fischborn T. Untersuchungen zum Trocknungsverhalten untergaeriger Hefen. 1997. Technische Universitaet Muenchen;Ph.D. Disser.
7. Fischer K, Rahn J. Einfluss der Stammkonservierung auf die genetische Satabilitaet von Backhefen. Branntweinwirtschfaft. 2000. 140:17–21.
8. Fontana A, Bidenne C, Ghommidh C, Guiraud P, Vezinhet F. Study of the flocculation of Saccharomyces diastaticus NCYC 625. J Inst Brew. 1992. 98:401–407.
9. Harrison M. Laboratory management of yeast. Ferment. 1996. 9:35–36.
10. Hill LR. Norris JR, Richmond MH, editors. Preservation of microorganisms. Essays in Appl Microbiol. 1981. New York, London, Sydney, Toronto: John Wiley, Sons Ltd..
11. Jibiki MA, Ishibiki T, Yamashita H, Eto M. A rapid and simple assay to measure flocculation in brewer's yeast. The Masters Brewers Association of the Americans Technical Quarterly. 1997. 34:278–281.
12. Kamada K, Murata M. On the mechanism of brewer's yeast flocculation. Agric Biol Chem. 1984. 48:2423–2433.


13. Pfenninger H. Brautechnische analysenmethoden Band II. Selbstverlag der Mitteleuropaeische Brautechnische Analysenkommission. 1993. 3rd Edition. Freising: Auflage.
14. Quain DE, Tubb RS. The importance of glycogen in brewing yeasts. The Masters Brewers Association of the Americans Technical Quarterly. 1982. 19:29–33.
15. Romano P, Suzzi G, Vannini L. Relationship between foaming and flocculence in Saccharomyces cerevisiae wine yeasts. Colloids Surf B Biointerfaces. 1994. 2:511–515.


16. Rouxhet PG, Mozes N, Dengis PB, Dufrene YF, Gerin PA, Genet MJ. Application of X-ray photoelection spectroscopy to microorganisms. Colloids Surf B Biointerfaces. 1994. 2:347–369.
17. Sips R. Quantifizierung und Identifizierung von Starterkulturen nach morphologischen und genetischen Kriterien. 1998. Technische Universitaet Berlin;Ph.D. Disser.
18. Smit G, Straver MH, Lugtenberg BJJ, Kijne JW. Flocculence of Saccharomyces cerevisiae cells is induced by nutrient limitation, with cell surface hydrophobicity as a major determinant. Appl Environ Microbiol. 1992. 58:3709–3714.


20. Straver MH, Smit G, Kjine JW. Induced cell surface hydrophobicity influences of brewer's yeast during fermentation in wort. Yeast. 1994. 9:527–532.


21. Straver MH, Smit G, Kjine JW. Isolation and partial characterization of a mannose specific agglutinin from brewer's yeast involved in flocculation. Yeast. 1994. 10:1183–1193.


22. Stewart RJ, Russel I, Stewart GG. Characterization of affinity-purified cell wall-binding proteins of Saccharomyces cerevisiae: possible role in flocculation. American Society of Brewing Chemists. 1995. 53:111–116.


23. Thorne RSW, Nohr B. Some observation on the stability of a brewing yeast strain. The Brewers Digest. 1963. 38:36–39.
24. Tybussek R, Linz F, Schuegerl K, Mozes N, Leonard AJ, Rouxhet PG. Comparison of the continuous flotation performances of Saccharomyces cerevisiae LBG H620 and DSM 2155 strains. Appl Microbiol Biotechnol. 1994. 41:13–22.


25. Van Hamersveld EH, Van der Lans RGJM, Caulet PJC, Luyben KChAM. Modeling brewer's yeast flocculation. Biotechnol Bioeng. 1998. 57:331–341.


26. Wackerbauer K, Tayama T, Fitzner M, Kunerth S. Zeitgemaesses management der Anstellhefe. Brauwelt. 1997a. 3:80–87.
27. Wackerbauer K, Tayama T, Kunerth S. Neurere Erkenntnisse des Einflusses der Hefelagerung auf die Gaeaktivitaet und Vitalitaet von Hefen in nachfolgenden Gaerungen. Monatsschrift fuer Brauwissenschaft. 1997b. 50:132–137.