Abstract
Purpose
There is evidence supporting the concept of tumor progression from pulmonary adenocarcinoma in situ (formerly bronchioloalveolar carcinoma, BAC) to adenocarcinoma with varying degrees of invasion. The aim of this study was to investigate the role of transforming growth factor β1 (TGFβ1) in tumor invasiveness in lung adenocarcinoma, and to determine the potential relationships between its expression and immunophenotypes of cell adhesion molecules.
Materials and Methods
Tumor samples from adenocarcinoma in situ (n=13), minimally invasive adenocarcinoma (formerly BAC with ≤5 mm invasion, n=2), and lepidic predominant invasive adenocarcinoma (formerly mixed adenocarcinoma showing non-mucinous BAC features with >5 mm invasion, n=25) were examined for the expression of TGFβ1, E-cadherin, N-cadherin, and H-cadherin proteins using immunohistochemistry.
Results
Of a total of 40 cases, 25 (63%) were positive for TGFβ1. The frequency of immunoreactivity in patients with adenocarcinoma in situ, minimally invasive adenocarcinoma, and lepidic predominant invasive adenocarcinoma was 23% (3/13), 50% (1/2), and 84% (21/25), respectively (p=0.001). TGFβ1 correlated with T classification (p=0.006) and stage (p=0.001). Loss of E-cadherin expression was more frequently observed in invasive adenocarcinomas than in adenocarcinomas in situ (p=0.034). E-cadherin expression inversely correlated with T classification (p=0.009). TGFβ1 expression showed a statistically significant correlation with H-cadherin expression (p=0.040), but not with E-cadherin expression (p=0.752).
Pulmonary adenocarcinoma in situ (formerly bronchioloalveolar carcinoma, BAC) grows in a lepidic fashion along the alveolar septa. The diagnosis is restricted to noninvasive lesions as defined by the World Health Organization (WHO) (1). The lepidic growth pattern sometimes seen in varying degrees with invasive adenocarcinomas (2) has led to the hypothesis of tumor progression from in situ carcinoma to invasive adenocarcinoma (3,4). Sequential accumulation of genetic aberrations may resultin the development of increasingly invasive tumor phenotypes. It remains unclear which molecular events are responsible for invasive progression of pulmonary adenocarcinoma.
The transforming growth factor β (TGFβ) signaling pathway plays a dual role in epithelial malignancies. TGFβ acts as a tumor suppressor early in tumorigenesis, but when genetic or epigenetic alterations in multiple pathways compromise the tumor suppressor activity later in the process, it then functions as an oncogene to promote tumor progression (5,6). Three TGFβ isoforms (TGFβ1, TGFβ2, TGFβ3) are expressed in mammals, and TGFβ1 is the most abundant and universally expressed isoform. The expression of TGFβ1 has been reported in various human malignancies, including lung carcinoma. TGFβ1 expression is associated with angiogenesis and plays a role in tumor progression of non-small cell lung carcinomas (NSCLC) (7). The acquisition of epithelial-to-mesenchymal transition (EMT) may be induced by TGFβ, especially TGFβ1 (8,9). EMT phenotype in cancers has been associated with tumor invasion, metastasis and poor clinical outcome (9). However, the precise mechanisms that mediate these processes remain poorly defined, especially for lung adenocarcinoma.
In the present study, samples obtained from patients with pulmonary adenocarcinoma demonstrating lepidic pattern with or without invasion were re-categorized according to the new criteria by the International Association for the Study of Lung Cancer, American Thoracic Society, and European Respiratory Society (10), and were investigated using immunohistochemistry for the expression of TGFβ1 to determine its role in invasive progression. Cell adhesion molecules, including E-cadherin, N-cadherin, and H-cadherin proteins were also evaluated for possible correlation with TGFβ1.
Pathology reports were searched through a computerized database for patients who were diagnosed as having BAC or adenocarcinoma with BAC features after lung resection at our institution from January 2007 to December 2010. Forty cases with available paraffin blocks were included in this study. All the hematoxylin-eosin stained slides were reviewed and reclassified by an experienced pathologist (J. Yoo) on the basis of the amount of invasive component as described recently by the International Association for the Study of Lung Cancer, American Thoracic Society, and European Respiratory Society (10). Tumors were categorized as 1) adenocarcinoma in situ [AIS, ≤3 cm formerly BAC as defined by WHO (1)] if there was no evidence of invasion, 2) minimally invasive adenocarcinoma (MIA, formerly BAC with focal invasion) if there was a predominant lepidic pattern with an area of invasion comprising ≤5 mm in the total tumor mass ≤3 cm, 3) lepidic predominant invasive adenocarcinoma (LPIA, formerly nonmucinous BAC pattern with >5 mm invasion). The study protocol was approved by the Institutional Review Board (IRB) of St. Vincent's Hospital, The Catholic University of Korea (IRB No. VC10SIS10028). Informed consent was waived by the IRB. Corresponding clinical information was obtained from patient medical records.
Tissue sections were obtained from 40 formalin-fixed, paraffin-embedded specimens. Immunohistochemical studies were performed using a sensitive peroxidase-streptavidin method for detection of the following antigens: TGFβ1 (Santa Cruz Biotechnology, Inc., Santa Cruz, CA, USA), E-cadherin (Cell Marque, Rocklin, CA, USA), N-cadherin (Cell Marque), and H-cadherin (Cell Marque). Sections 4µm thick were placed on positively charged slides, deparaffinized in xylene and rehydrated in graded alcohols and water. Endogenous peroxidase activity was blocked with 3% H2O2 in methanol at 45℃ for 30 minutes. For antigen retrieval, the sections were immersed in a citrate buffer (2.1 g/L, pH 6.0) in an autoclave for 15 minutes. The slides were treated with a protein blocking reagent for one hour before overnight incubation at 4℃ in a humidified chamber with primary antibodies at a 1:50 dilution, as recommended by manufacturer instructions. After washing with Tris buffer, the immunoperoxidase activity was detected using the UltraVision LP detection system (Thermo Fisher Scientific, Fremont, CA, USA). 0.05% 3,3'-diaminobenzidine was used as a chromogen and hematoxylin as a nuclear counterstain.
Positive and negative controls were run in all series. Positive controls were breast carcinoma tissue for TGFβ1 and E-cadherin, pituitary gland for N-cadherin, and heart for H-cadherin. For negative controls, the primary antibody was omitted during processing. All immunostained slides were examined by a pathologist (J. Yoo) in a blind fashion. Expression for TGFβ1 was evaluated according to the percentage of immunoreactive cells to total tumor cells showing immunoreactivities in cytoplasm: +, ≥10% of tumor cells were stained; and -, no detectable expression or <10% of tumor cells were stained (11). E-cadherin and N-cadherin stained the membrane strongly and the cytoplasm weakly. H-cadherin stained cytoplasm. Expressions for E-cadherin and H-cadherin were evaluated according to the percentage of immunoreactive cells to total tumor cells showing immunoreactivities: 0, 0~10%; 1+, 10~30%; 2+, 30~70%; 3+, >70%), and were interpreted as positive when the scores were ≥2 (12). Cases with ≥2% immunoreactive tumor cells were assigned a positive score for N-cadherin (13).
Statistical analysis was carried out using the SPSS software package version 13.0 (SPSS Inc., Chicago, IL, USA). Associations between clinicopathologic factors and protein expressions were estimated using Spearman's chi-square test and Fisher's exact test. All p-values were two-sided. p<0.05 was considered statistically significant.
Table 1 summarizes the patient demographics for the 40 cases. Patients ranged in age from 30~78 years (mean age, 61.2 years). There was a great predominance of women to men, and most patients reported a negative smoking history. There were 26 women (65%) vs. 14 men (35%), and 30 neversmokers (75%) vs. 10 former or current smokers (25%). The tumor was ≤3 cm in 30 patients (75%), and >3 cm in 10 patients (25%). Upon pathology review, tumor histology was reclassified to AIS in 13 patients (33%), MIA in 2 patients (5%), and LPIA in 25 patients (63%). Most tumors presented as pathologic Tis-T2 (95%) and pathologic stage 0-II (85%).
All samples were investigated for the expression of TGFβ1, E-cadherin, N-cadherin, and H-cadherin proteins. The negative and positive controls gave the expected results. Positive immunostaining for TGFβ1 was observed in 25 tumors (63%) (Table 2, Fig. 1A). TGFβ1 expression was positive in 3 (23%) of Tis, in 21 (84%) of T1~2 tumors, and in 1 (50%) of T3~4 tumors. This difference was statistically significant (p=0.006). TGFβ1 was more frequently expressed in patients with N1-3 status than in patients with N0 status (78% vs. 58%), but the difference was not statistical significant (p=0.232). TGFβ1 was detected in 3 (23%) of the patients with stage 0 disease, whereas it was overexpressed in 17 (81%) of patients with stage I-II disease and in 5 (83%) of patients with stage III-IV disease (p=0.001). E-cadherin was expressed in 20 tumors (50%), N-cadherin in 2 tumors (5%), and H-cadherin in 6 tumors (15%) (Table 2, Fig. 1B~D). E-cadherin was preserved in 9 (69%) of Tis, in 10 (40%) of T1~2 tumors, and in 1 (50%) of T3~4 tumors. This difference was a statistically significant negative correlation (p=0.009). N status and stage were not associated with E-cadherin expression. There were no associations between the N-cadherin expression and any of parameters. Of 6 samples positive for H-cadherin, one was an in situ lesion (Tis, p=0.090; and stage 0, p=0.062) and the other 5 were invasive lesions.
A greater prevalence of TGFβ1 expression was identified with increasingly invasive tumor phenotypes: 3 in AIS (23%), 1 in MIA (50%), and 21 in LPIA (84%) (Table 3). This difference was statistically significant (p=0.001). Loss of E-cadherin expression was significantly more frequent in LPIA than in AIS: 64% (16/25) vs. 31% (4/13) (p=0.034). N-cadherin expression was detected only in 2 cases with LPIA (2/25, 8%; p=0.279). H-cadherin was expressed in 8% (1/13) of AIS, 50% (1/2) of MIA, and 16% (4/25) of LPIA (p=0.646). When the AIS and MIA were grouped together, the results were the same. The central area of the LPIA, which showed invasive components, and the peripheral area, which showed lepidic pattern, were examined separately for the immunoreactivities for each protein. Sixteen of 21 TGFβ1-positive LPIAs demonstrated immunostaining only in the central area. On the other hand, E-cadherin staining was more frequently identified in the tumor cells within the peripheral region than in the tumor cells within the central region. No association was found between TGFβ1 and E-cadherin expressions (p=0.752) (Table 4). TGFβ1, however, strongly correlated with H-cadherin expression (p=0.040).
TGFβs are members of a large superfamily of pleiotropic cytokines, and regulate complex processes including cell proliferation, differentiation, adhesion, cell-cell and cell-matrix interactions, motility, and cell death (14). TGFβs bind to a heteromeric complex of serine/threonine kinases, the type I and type II receptors (TβRI and TβRII). Following ligand binding to TβRII, TβRI is recruited to the complex, which allows for the constitutively active TβRII kinase to transphosphorylate and activate TβRI and thus regulates gene transcription (15). Overexpression of TGFβ is frequently associated with metastasis and poor prognosis. Furthermore, TGFβ antagonism has been shown to prevent metastasis in preclinical models (16-19). There are, however, no collective data regarding the significance of TGFβ1 expression in pulmonary adenocarcinomas with lepidic pattern. In the present study, we hypothesized that TGFβ1 is associated with invasive progression in the putative progression model from in situ to invasive adenocarcinoma. We found that the prevalence of TGFβ1 expression increased in tumors with progressively more invasive histologic type (from 23% in AIS to 50% in MIA, and to 84% in LPIA). In LPIAs, TGFβ1 was more frequently identified in the tumor cells within the central region than in the tumor cells within the peripheral region. Our findings were supported by the results of other investigations on gastric carcinoma, colorectal carcinoma and NSCLC (7,11,20). An investigation of NSCLC by Hasegawa et al. (7) showed that TGFβ1 protein level measured by enzyme-linked immunoadsorbent assay was higher in patients with lymph node metastasis and in patients with advanced stage. Their study also demonstrated the effects of TGFβ1 on angiogenesis, exhibiting a positive association of microvessel density with TGFβ1. Invasive properties during intravasation are reminiscent of EMT that occurs during embyogenesis. Hallmarks of carcinoma cells undergoing EMT are loss of polarity and cell-cell contacts (19,21).
Cell-to-cell adhesion is important in maintaining tissue architecture. Cadherins are cell-surface glycoproteins that mediate cell-to-cell adhesion, and include E-cadherin, N-cadherin, and H-cadherin. E-cadherin has been subjected to extensive investigation, and reduced or absent E-cadherin has been identified to be associated with dedifferentiation, invasion and/or metastasis in various cancers (21,22). The expression of E-cadherin was significantly decreased with an increased depth of tumor invasion of colorectal cancer (23). In NSCLC, E-cadherin promoter hypermethylation and loss of E-cadherin expression were associated with the development of malignant phenotype (22), suggesting that E-cadherin may be an important component of tumor invasiveness. The present study also revealed a negative association of E-cadherin expression with invasive histology.
N-cadherin is a major cell adhesion molecule in normal physiology as well as in tumorigenesis. Up-regulation of N-cadherin is associated with induced cellular motility (24), suggesting that it may mediate adhesion of malignant cells to stromal or endothelial cells and facilitate invasion of tumor cells. We observed N-cadherin expression in only 2 patients, both with LPIA, showing no association with E-cadherin expression. To the best of our knowledge, the significance of N-cadherin expression in pulmonary adenocarcinoma has not been investigated. There is a need to conduct further work on a larger scale for N-cadherin linked to the classical cadherins.
In a study of breast cancer cell lines, EMT was induced after treatment with TGFβ, as evidenced by a strong morphologic epithelial to fibroblastic tumor differentiation and inhibition of E-cadherin (21). We observed in the current study that more invasive morphologies are positively associated with TGFβ1 and negatively with E-cadherin. Although no direct association was identified between TGFβ1 and E-cadherin expressions, TGFβ1 expression is significantly associated with H-cadherin expression. In contrast to classical cadherins, accumulating data implicate that H-cadherin is unlikely to function in the maintenance of cell-to-cell adhesion, but rather, is involved in intercellular signaling (25). Furthermore, the role of H-cadherin in EMT was recently confirmed in vivo and in vitro models (26). Our results of an association between TGFβ1 and H-cadherin expressions suggest that H-cadherin in tumor cells may represent an additional mechanism contributing to the incorporation of EMT-mediators. The acquisition of an EMT phenotype may be an important step in invasive progression from in situ to invasive tumor of pulmonary adenocarcinoma, and may be accomplished by integration of several complex EMT mediators. Further studies to ascertain the role of TGFβ1-induced EMT are warranted, including epithelial and mesenchymal markers.
In conclusion, high expression of the EMT phenotype (high TGFβ1 and low E-cadherin) was more frequently observed in tumors with invasive morphology, suggesting that TGFβ1 overexpression and down-regulation of E-cadherin may provide a favorable environment for tumor cell invasion. Although TGFβ1, E-cadherin, and H-cadherin have been implicated in EMT, the underlying molecular mechanisms need to be elucidated.
Figures and Tables
Fig. 1
Immunohistochemistry for transforming growth factor β1 showing cytoplasmic positivity (A), E-cadherin showing predominantly membranous reactivity (B), N-cadherin showing membranous and cytoplasmic staining (C), and H-cadherin showing cytoplasmic reactivity (D) (×100).
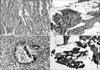
References
1. Colvy TV, Noguchi M, Henschke C, et al. Travis WD, Brambilla E, Müller-Hermelink HK, Harris CC, editors. Adenocarcinoma. World Health Organization classification of tumours: pathology and genetics of tumours of the lung, pleura, thymus and heart. 2004. Lyon: IARC Press;35–44.
2. Laskin JJ, Sandler AB, Johnson DH. Redefining bronchioloalveolar carcinoma. Semin Oncol. 2005. 32:329–335.


3. Aoyagi Y, Yokose T, Minami Y, et al. Accumulation of losses of heterozygosity and multistep carcinogenesis in pulmonary adenocarcinoma. Cancer Res. 2001. 61:7950–7954.
4. Sarkaria IS, Pham D, Ghossein RA, et al. SCCRO expression correlates with invasive progression in bronchioloalveolar carcinoma. Ann Thorac Surg. 2004. 78:1734–1741.


5. Yang Y, Dukhanina O, Tang B, et al. Lifetime exposure to a soluble TGF-β antagonist protects mice against metastasis without adverse side effects. J Clin Invest. 2002. 109:1607–1615.


6. Elliott RL, Blobe GC. Role of transforming growth factor beta in human cancer. J Clin Oncol. 2005. 23:2078–2093.


7. Hasegawa Y, Takanashi S, Kanehira Y, Tsushima T, Imai T, Okumura K. Transforming growth factor-β1 level correlates with angiogenesis, tumor progression, and prognosis in patients with nonsmall cell lung carcinoma. Cancer. 2001. 91:964–971.


8. Gupta PB, Onder TT, Jiang G, et al. Identification of selective inhibitors of cancer stem cells by high-throughput screening. Cell. 2009. 138:645–659.


9. Maitah MY, Ali S, Ahmad A, Gadgeel S, Sarkar FH. Upregulation of sonic hedgehog contributes to TGF-β1-induced epithelial to mesenchymal transition in NSCLC cells. PLoS One. 2011. 6:e16068.


10. Travis WD, Brambilla E, Noguchi M, et al. International association for the study of lung cancer/americna thoracic society/European respiratory society international multidisciplinary classification of lung adenocarcinoma. J Thorac Oncol. 2011. 6:244–285.
11. Saito H, Tsujutani S, Oka S, et al. The expression of transforming growth factor-β1 is significantly correlated with expression of vascular endothelial growth factor and poor prognosis of patients with advanced gastric carcinoma. Cancer. 1999. 86:1455–1462.


12. Kashiwagi S, Yashiro M, Takashima T, et al. Significance of E-cadherin expression in triple-negative breast cancer. Br J Cancer. 2010. 103:249–255.


13. Grinberg-Rashi H, Ofek E, Perelman M, et al. The expression of three genes in primary non-small cell lung cancer is associated with metastatic spread to the brain. Clin Cancer Res. 2009. 15:1755–1761.


14. Muraoka-Cook RS, Kurukawa H, Koh Y, et al. Conditional overexpression of active transforming growth factor β1 in vivo accelerates metastases of transgenic mammary tumors. Cancer Res. 2004. 64:9002–9011.


15. Santibañez JF, Quintanilla M, Bernabeu C. TGF-β/TGF-β receptor system and its role in physiological and pathological conditions. Clin Sci (Lond). 2011. 121:233–251.


16. Toonkel RL, Borczuk AC, Powell CA. TGF-β signaling pathway in lung adenocarcinoma invasion. J Thorac Oncol. 2010. 5:153–157.


17. Bandyopadhyay A, Agyin JK, Wang L, et al. Inhibition of pulmonary and skeletal metastasis by a transforming growth factor-beta type I receptor kinase inhibitor. Cancer Res. 2006. 66:6714–6721.


18. Nam JS, Terabe M, Mamura M, et al. An anti-transforming growth factor beta antibody suppresses metastasis via cooperative effects on multiple cell compartments. Cancer Res. 2008. 68:3835–3843.


19. Zhong Z, Carroll KD, Policarpio D, et al. Anti-transforming growth factor β receptor II antibody has therapeutic efficacy against primary tumor growth and metastasis through multieffects on cancer, stroma, and immune cells. Clin Cancer Res. 2010. 16:1191–1205.


20. Guzinska-Ustymowicz K, Kemona A. Transforming growth factor beta can be a parameter of aggressiveness of pT1 colorectal cancer. World J Gastroenterol. 2005. 11:1193–1195.


21. Deckers M, van Dinther M, Buijs J, et al. The tumor suppressor Smad4 is required for transforming growth factor β-induced epithelial to mesenchymal transition and bone metastasis of breast cancer cells. Cancer Res. 2006. 66:2202–2209.


22. Bremnes RM, Veve R, Hirsch FR, Franklin WA. The E-cadherin cell-cell adhesion complex and lung cancer invasion, metastasis, and prognosis. Lung Cancer. 2002. 36:115–124.


23. Hong RH, Choi DY, Lim SC, Suh CH, Kee KH, Lee MJ. The differential expressions of the epithelial-mesenchymal transition regulator, Slug and the cell adhesion molecule, E-cadherin in colorectal adenocarcinoma. Korean J Pathol. 2008. 42:351–357.
24. Maret D, Gruzglin E, Sadr MS, et al. Surface expression of precursor N-cadherin promotes tumor cell invasion. Neoplasia. 2010. 12:1066–1080.

