Abstract
The molecular approaches to distal renal tubular acidosis (dRTA) associated AE1 mutations lead us to understand the genetic and pathophysiological aspects of the acidification defects. An unanticipated high value of the urine-blood (U-B) PCO2 after NaHCO3 loading observed in a case of dRTA and southeast Asian ovalocytosis (SAO) might be from a mistarget of the AE1 to the luminal membrane of type A intercalated cells. The mutations of the AE1 gene resulted in SAO and also affected renal acidification function. Notwithstanding, after the NH4Cl loading in 20 individuals with SAO, the acidification in the distal nephron was normal. The presence of both SAO and G701D mutations of AE1 gene would explain the abnormal urinary acidification in the patients with the compound heterozogosity. In terms of the effect of the mutations on trafficking of AE1, truncated kidney isoform (kAE1) of wild-type showed a 'dominant-positive effect' in rescuing the recessive mutant kAE1 (S773P or G701D) trafficking to the plasma membrane, in contrast with the dominant mutant kAE1 (R589H) resulting in a 'dominant-negative effect' when heterodimerized with the wild-type kAE1. It is notable that the dominant mutants kAE1 (R901X or G609R) expression in MDCK cells clearly results in aberrant surface expression with some mutant protein appearing at the apical membrane. These might result in net bicarbonate secretion and increasing U-B PCO2 in the distal nephron. The molecular physiological and genetic approaches have permitted identification of the molecular defects, predominantly in transporter proteins, and should in turn prompt development of novel therapeutic strategies.
To maintain acid-base homeostasis, the kidney must reclaim all filtered bicarbonate (HCO3-) and regenerate new HCO3- by excreting net acid sufficient in quantity to match that produced by systemic metabolism. The net acid excretion is mediated largely by energy-dependent proton pumps located in the apical membrane of type A intercalated cells of the distal nephron1). Failure of the urinary acidification process in the distal nephron results in distal renal tubular acidosis (dRTA). The clinical and biological features of dRTA include hyperchloremic metabolic acidosis, hypokalemia, impaired growth, nephrocalcinosis, nephrolithiasis, hypercalciuria, hypocitraturia, and rickets or osteomalacia2, 3).
dRTA is highly prevalent in the northeast of Thailand and has been given the name endemic dRTA4). There was a spectrum of tubulointerstitial abnormalities ranging from suspected to overt dRTA in the geographic area. Possible environmental insults such as potassium (K+) deficiency, might be the important pathogenic factor of endemic dRTA in the northeast of Thailand5). Notwithstanding, familial dRTA inherited in both autosomal dominant (AD) and autosomal recessive (AR) pattern6-11), have been reported in different kindreds with primary dRTA. Some patients remain asymptomatic until adolescence or adulthood, whereas others may be severely affected in infancy, with impaired growth and early nephrocalcinosis causing eventual renal insufficiency. Our studies2, 4) showed that the endemic dRTA was also seen in children in the northeast of Thailand and had familial tendency.
In the past decade, the molecular genetic approach of positional cloning and candidate gene analysis have been combined to identify the genes responsible for these inherited conditions12, 13) and have enhanced our understanding of normal renal physiology. This review will demonstrate the molecular approach for the cases of dRTA in Thailand in order to understand the genetic causes of primary inherited dRTA. It will, in addition, evaluate the ability of known functional and biochemical properties of these mutant proteins to explain the pathophysiology of associated renal acidification defects.
We had reported a case of dRTA and SAO from the southern part of Thailand14). The patient was a 33-year-old woman who presented with 4 months of generalized muscle weakness. Only she and her father had SAO. Her principal laboratory findings were hypokalemic metabolic acidosis and a normal anion gap in her plasma (Table 1).
The results showed hypokalemia with a relatively high transtubular K+ gradient (TTKG) and K+ excretion rate, a relatively low rate of excretion of NH4+ and high urine pH (>5.5) during hyperchloremic metabolic acidosis. These are all typical for the a patient with dRTA15).
To investigate the pathogenesis of dRTA, urine-blood (U-B) PCO2 after NaHCO3 loading is a useful diagnostic tool to provide a qualitative reflection of the secretion of H+ in type A intercalated cells of the distal nephron16, 17). After the bicarbonate loading of the patient with her urine pH up to 7.7, her U-B PCO2 was increased for more than 25 mm Hg (27 mm Hg). The U-B PCO2 in two patients with hereditary spherocytosis and incomplete dRTA coinherited with a mutation in the AE1 gene was also reported to have an increased U-B PCO2 within the normal range after the bicarbonate loading18). There was an unanticipated high value for the U-B PCO2 of the patients, perhaps the result of HCO3- secretion, which itself could also reduce the net rate of secretion of H+ in the distal nephron. The explanation might be from a mistarget of the Cl-/HCO3- exchanger 1 (AE1) from the basolateral membrane to the luminal membrane of the type A intercalated cells14).
SAO is common in parts of Southeast Asia and Melanesia with a prevalence reaching 30% in certain ethnic groups19). It has been shown that SAO results from an AE1 gene mutation, which decreases red cell membrane AE1 transport and increases membrane rigidity20, 21). The AE1 gene which encode AE1, multifunctional polytopic membrane glycoprotein containing 911 amino acids, is located on chromosome 17q21-q22 encompassing approximately 20 kb and consisting of 20 exons separated by 19 introns. The mutation of the abnormal AE1 gene in SAO showed that it contained the two linked mutations: a deletion of codons 400 to 408 in the boundary of the cytoplasmic domains and a point mutation in the first base of codon 56 (K56E), the Memphis I, polymorphism.
AE1 is also found in the basolateral membrane of the type A intercalated cells of renal collecting ducts, which is involved in H+ secretion22). Kidney AE1 (kAE1) is transcribed from an alternative promoter resulting in the production in human kidney of an isoform that is missing the N-terminal 65 amino acids found in erythroid isoform (eAE1). Many studies and reviews10, 11, 23, 24) have shown that mutations of AE1 gene affect renal acidification function. To examine the possibility, we studied the renal acidification of the subjects with SAO24). Table 2 provides the results after the short acid loading study by oral administration of 0.1 g/kg NH4Cl in the 10 patients with SAO and 7 control subjects. Urine pH and NH4+ excretion rate after the acid load were not significantly different (5.0±0.1 vs 4.9±0.1, and 39±6 vs 37±4 uEq/min respectively). We also studied the NH4+ excretion rate and urine pH after the three-day NH4Cl loading in another 10 patients with SAO and 4 control subjects. Neither the subject groups after the acid loading had a statistical significant difference in the NH4+ excretion rate (65±6 vs 65±4 uEq/min) and urine pH (5.0±0.1 vs 4.9±0.1). These suggest that the rate of excretion of NH4+ or the function of HCO3- regeneration of the kidney in the patients with SAO was normal. Thus, the SAO mutation of AE1 gene is not sufficient to cause dRTA.
During the previous acid-loading studies, we also identified the two clinically affected unrelated patients in the families with dRTA and SAO24). The two patients showed a low rate of NH4+ excretion and an inability to lower the urine pH below 5.5 in the presence of systemic acidosis. The patients also have a high TTKG given the degree of hypokalemia. No abnormal renal acidification was detected in their parents.
The mutation of AE1 gene in the two patients with dRTA and SAO and their families (YAT and KSN family) was studied. Leukocyte genomic DNA samples from the propositi and their parents were screened for mutations in exons 4 to 20 of the AE1 gene and in intron 3, the promoter region of the kidney isoform, with polymerase chain reaction (PCR) and single strand conformational polymorphism (SSCP). The results showed that I-1 (the father of YAT) and I-2 (the mother of KSN) had the mobility shift in exon 17. I-2 (the mother of YAT) and I-1 (the father of KSN) had the mobility shift in exon 11. The patients (II-1) in both families had mobility shifts of single-stranded DNA in both exons 11 and 17. The PCR-SSCP patterns of all the other exons, including intron 3, were normal, except for the mobility shift of exon 4 (the Memphis I polymorphism). Mutations of the AE1 gene were also analyzed by the PCR-SSCP method in 20 individuals with SAO. The individuals with SAO had only mobility shifts of only exons 4 and 11.
The DNA samples of both YAT and KSN families revealed the mobility shift in exons 11 and the 17 of the AE1 gene. Therefore, amplified DNA of these two exons from the propositus of the KSN family was sequenced. The results showed that exon 11 had a deletion of 27 bp corresponding to codons 400 to 408 whereas exon 17 contained a nucleotide substitution of G to A in codon 701 (CGG→CAG), resulting in an amino acid change from glycine to aspartic acid (G701D, one point mutation). The sequencing results also showed the presence of homozygous band 3 Memphis I.
Studies of the influx of [35S] sulfate into red cells of the two patients and their parents indicated a consistently lower anion transport activity than the normal red cells, except for the individuals with exon 17 mutation (G701D), whom had normal anion transport activity. Although the presence of both SAO and G701D mutations had no additional effect on [35S] sulfate transport into red cells compared to the presence of SAO mutation alone, the AE1 exchanger activity in the type A intercalated cells of the renal collecting duct in the patients with the combined mutations may be abnormal. Two siblings with dRTA and hemolytic anemia have recently been found to carry a homozygous G701D missense mutation of the AE1 gene with apparently normal erythroid anion transport in the affected children and their parents8). From the expression study of G701D mutation in Xenopus oocytes, it has also been shown that the mutant protein was not transported to the surface of the cell membrane8). However if the G701D mutation was co-expressed with glycophorin A, the erythroid band 3 chaperonin, both AE1 surface expression and AE1-mediated Cl- transport were rescued. Therefore, the presence of both SAO and G701D mutation of AE1 gene would have a greater effect to the type A intercalated cells than the presence of either mutation alone, and this would explain the abnormal urinary acidification in the patients with the compound heterozogosity.
To examine the effect of mutations on the trafficking of AE1 in the patient with dRTA, Kittanokom et al.25) transiently transfected erythroid AE1 (eAE1) and truncated kidney isoform (kAE1) of the wild-type and a novel recessive missense dRTA mutation of compound heterozygous AE1 G701D/S773P6) into a human kidney cell line, human embryonic kidney (HEK)-293. The results showed that the mutant kAE1 protein of S773P could not be detected at the plasma membrane of transfected HEK 293 cells. The study showed predominant immunolocalization of the mutant protein in the endoplasmic reticulum (ER) of the HEK 293. The mutant S773P was not properly folded, as it showed decreased binding to an inhibitor affinity resin and increased sensitivity to proteases. The kAE1 G701D also exhibited defective trafficking to the plasma membrane. The kAE1 S773P was able to form homodimers and heterodimers with wild-type kAE1 or with kAE1 G701D. Heterodimers of the wild-type kAE1 with kAE1 S773P or G701D could be delivered to the plasma membrane. Whereas, the study of Quilty JA, et al.26) showed that coexpression of mutant kAE1 of the dominant R589H mutation with wild-type kAE1 impaired trafficking of the wild-type protein. Thus, the wild-type kAE1 seems to show a 'dominant-positive effect' in rescuing the recessive mutant kAE1 trafficking to the plasma membrane, in contrast with the dominant mutant kAE1 resulting in a 'dominant-negative effect' when heterodimerized with the wild-type kAE1. With little or no kAE1 at the basolateral membrane of type A intercalated cell, HCO3- could not be effectively removed from the cell, reducing the production of HCO3- and H+ by carbonic anhydrase. The reduced production of H+ would result in less acidification of the urine.
From the study in Madin-Darby canine kidney (MDCK) epithelial cells27), the results showed that the recessive mutants, kAE1 S773P and G701D had distinct trafficking defects. The recessive mutant kAE1 S773P, while misfolded and largely retained in the ER in non-polarized MDCK cells, was targeted to the basolateral membrane after polarization while kAE1 G701D was retained in the Golgi in both non-polarized and polarized cells. The co-expression study in MDCK cells also confirmed that the dominant mutant kAE1 R589H retained wild-type kAE1 protein, while the recessive kAE1 mutants did not. The co-expression of kAE1 S773P and G701D showed some co-localization of S773P with G701D in the Golgi, but kAE1 S773P could still traffic to the basolateral membrane. No kAE1 G701D was detected at the cell surface, suggesting that kAE1 S773P did not assist the retained kAE1 G701D to traffic to the cell surface, as did the wild-type kAE1, despite their ability to oligomerize in the MDCK cells. Therefore, compound heterozygous G701D/S773P mutations, only the mis-folded S773P/S773P homodimers which may not properly function, can reach the basolateral membrane of the kidney type A intercalated cells, resulting in the development of dRTA.
Co-expression of kAE1 and kAE1 SAO with the dRTA mutants (G701D, V850, R602H, and A858D) was also studied in polarized epithelial MDCK cells28). The results showed that decreasing in cell-surface expression of the dRTA mutants as a result of the interaction with kAE1 SAO would account for the impaired expression of functional kAE1 at the basolateral membrane of α-intercalated cells, resulting in dRTA in compound heterozygous patients.
It is notable that the dominant mutants kAE1 R901X and G609R expression in MDCK cells grown to polarity clearly results in aberrant surface expression with some mutant protein appearing at the apical membrane29, 30). These mutations might result in net bicarbonate secretion into the urine and also reduce the net rate of secretion of H+ in the distal nephron. One way to address this would be to measure U-B PCO2 that would not be reduced to the extent usually observed in dRTA.
Approaching these conditions with molecular physiology and genetics has permitted identification and understanding of the molecular defects of the patients with dRTA and AE1 mutations. The modes of inheritance are primarily dictated by altering the position of amino acid in the encoded kAE1 gene and by the type of amino acid replacement. The mutant might affect kAE1 folding and molecular structure without significantly changing anion exchange function in the heterozygous state with wild-type kAE1. The mutant can form hetero-oligomers, a newly described 'dominant-positive effect', producing the AR dRTA phenotype. This does not prevent trafficking of the wild-type protein to the basolateral membrane of the type A intercalated cell to retain adequate bicarbonate transport into the blood. In the case of AR dRTA, the mutant homodimers can traffic to the Golgi (G701D) or the basolateral membrane (S773P), but since the latter protein is mis-folded, no transport activity is achieved. In contrast to the AD dRTA, the mutant kAE1 in the heterodimer induces a trafficking defect of wild-type kAE1, the so called 'dominant-negative effect', leading to the AD dRTA phenotype. The retention of the wild-type protein by the heterodimer formation does not allow sufficient kAE1 to be localized to the basolateral membrane. In some cases (R901X and G609R), the dominant mutants can exit the ER and are partially missorted to the apical membrane, which would also impair the basolateral bicarbonate reabsorption and apical acid secretion of the type A intercalated cells. The way to address this would be to measure U-B PCO2 that would not be reduced to the extent usually observed in dRTA.
This review demonstrated how to make it possible to evaluate and elucidate genotype-phenotype relationships and should in turn prompt development of novel therapeutic strategies.
Acknowledgements
Thanks to the APCN 2010 for its support of the Symposium; to my colleagues Sanga Nilwarangkur, Sumalee Nimmannit, Prida Malasit, Vipada Chaovakul, Wattanachai Susaengrat, Pathai Yenchitsomanus, Charoen Kaitwatcharachai, Prayong Vachuanichsanong, Peti Thuwajit, Suchai Sritippayawan, Vichai Laosombat, Prapon Wilairat, and Mitchell L. Halperin for their contributions to this work supported by grants from the Research Development and Medical Education Fund of the Faculty of Medicine, Siriraj Hospital, Mahidol University.
References
1. Karet FE, Finberg KE, Nelson RD, et al. Mutations in the gene encoding B1 subunit of H+-ATPase cause renal tubular acidosis with sensorineural deafness. Nat Genet. 1999; 21:84–90. PMID: 9916796.
2. Nilwarangkur S, Nimmannit S, Chaovakul V, et al. Endemic primary distal renal tubular acidosis in Thailand. Q J Med. 1990; 74:289–301. PMID: 2385736.
3. Halperin ML, Goldstein MB, Richardson RM, Stinebaugh BJ. Distal renal tubular acidosis syndromes: a pathophysiological approach. Am J Nephrol. 1985; 5:1–8. PMID: 3970076.


4. Nimmannit S, Malasit P, Susaengrat W, et al. Prevalence of endemic distal renal tubular acidosis and renal stone in the northeast of Thailand. Nephron. 1996; 72:604–610. PMID: 8730429.


5. Vasuvattakul S, Nimmannit S, Chaovakul V, et al. The spectrum of endemic renal tubular acidosis in the northeast of Thailand. Nephron. 1996; 74:541–547. PMID: 8938678.


6. Sritippayawan S, Sumboonnanonda A, Vasuvattakul S, et al. Novel compound heterozygous SLC4A1 mutations in Thai patients with autosomal recessive distal renal tubular acidosis. Am J Kidney Dis. 2004; 44:64–70. PMID: 15211439.


7. Yenchitsomanus PT, Vasuvattakul S, Kirdpon S, et al. Autosomal recessive distal renal tubular acidosis caused by G701D mutation of anion exchanger 1 gene. Am J Kidney Dis. 2002; 40:21–29. PMID: 12087557.


8. Tanphaichitr VS, Sumboonnanonda A, Ideguchi H, et al. Novel AE1 mutations in recessive distal renal tubular acidosis. Loss-of-function is rescued by glycophorin A. J Clin Invest. 1998; 102:2173–2179. PMID: 9854053.


9. Karet FE, Gainza FJ, Gyory AZ, et al. Mutations in the chloridebicarbonate exchanger gene AE1 cause autosomal dominant but not autosomal recessive distal renal tubular acidosis. Proc Natl Acad Sci U S A. 1998; 95:6337–6342. PMID: 9600966.


10. Jarolim P, Shayakul C, Prabakaran D, et al. Autosomal dominant distal renal tubular acidosis is associated in three families with heterozygosity for the R589H mutation in the AE1 (band 3) Cl-/HCO3- exchanger. J Biol Chem. 1998; 273:6380–6388. PMID: 9497368.
11. Bruce LJ, Cope DL, Jones GK, et al. Familial distal renal tubular acidosis is associated with mutations in the red cell anion exchanger (Band 3, AE1) gene. J Clin Invest. 1997; 100:1693–1707. PMID: 9312167.


12. Pereira PC, Miranda DM, Oliveira EA, Silva AC. Molecular pathophysiology of renal tubular acidosis. Curr Genomics. 2009; 10:51–59. PMID: 19721811.


13. Fry AC, Karet FE. Inherited renal acidoses. Physiology (Bethesda). 2007; 22:202–211. PMID: 17557941.


14. Kaitwatcharachai C, Vasuvattakul S, Yenchitsomanus P, et al. Distal renal tubular acidosis and high urine carbon dioxide tension in a patient with southeast Asian ovalocytosis. Am J Kidney Dis. 1999; 33:1147–1152. PMID: 10352205.


15. Kamel KS, Briceno LF, Sanchez MI, et al. A new classification for renal defects in net acid excretion. Am J Kidney Dis. 1997; 29:136–146. PMID: 9002543.


16. DuBose TD Jr, Caflisch CR. Validation of the difference in urine and blood carbon dioxide tension during bicarbonate loading as an index of distal nephron acidification in experimental models of distal renal tubular acidosis. J Clin Invest. 1985; 75:1116–1123. PMID: 3921566.


17. Halperin ML, Goldstein MB, Haig A, Johnson MD, Stinebaugh BJ. Studies on the pathogenesis of type I (distal) renal tubular acidosis as revealed by the urinary PCO2 tensions. J Clin Invest. 1974; 53:669–677. PMID: 4812435.


18. Rysava R, Tesar V, Jirsa M Jr, Brabec V, Jarolim P. Incomplete distal renal tubular acidosis coinherited with a mutation in the band 3 (AE1) gene. Nephrol Dial Transplant. 1997; 12:1869–1873. PMID: 9306337.


19. Lie-Injo LE FA, Bolton JM, Gilman RH. Hereditary elliptocytosis in Malayan aborigines. Acta Haematol. 1972; 47:210–216. PMID: 4625303.
20. Jarolim P, Palek J, Amato D, et al. Deletion in erythrocyte band 3 gene in malaria-resistant Southeast Asian ovalocytosis. Proc Natl Acad Sci U S A. 1991; 88:11022–11026. PMID: 1722314.


21. Liu SC, Zhai S, Palek J, et al. Molecular defect of the band 3 protein in southeast Asian ovalocytosis. N Engl J Med. 1990; 323:1530–1538. PMID: 2146504.


22. Wagner CA, Kovacikova J, Stehberger PA, Winter C, Benabbas C, Mohebbi N. Renal acid-base transport: old and new players. Nephron Physiol. 2006; 103:p1–p6. PMID: 16352913.


23. Alper SL. Molecular physiology of SLC4 anion exchangers. Exp Physiol. 2006; 91:153–161. PMID: 16239253.


24. Vasuvattakul S, Yenchitsomanus PT, Vachuanichsanong P, et al. Autosomal recessive distal renal tubular acidosis associated with Southeast Asian ovalocytosis. Kidney Int. 1999; 56:1674–1682. PMID: 10571775.


25. Kittanakom S, Cordat E, Akkarapatumwong V, Yenchitsomanus PT, Reithmeier RA. Trafficking defects of a novel autosomal recessive distal renal tubular acidosis mutant (S773P) of the human kidney anion exchanger (kAE1). J Biol Chem. 2004; 279:40960–40971. PMID: 15252044.


26. Quilty JA, Li J, Reithmeier RA. Impaired trafficking of distal renal tubular acidosis mutants of the human kidney anion exchanger kAE1. Am J Physiol Renal Physiol. 2002; 282:F810–F820. PMID: 11934690.


27. Cordat E, Kittanakom S, Yenchitsomanus PT, et al. Dominant and recessive distal renal tubular acidosis mutations of kidney anion exchanger 1 induce distinct trafficking defects in MDCK cells. Traffic. 2006; 7:117–128. PMID: 16420521.


28. Kittanakom S, Cordat E, Reithmeier RA. Dominant-negative effect of Southeast Asian ovalocytosis anion exchanger 1 in compound heterozygous distal renal tubular acidosis. Biochem J. 2008; 410:271–281. PMID: 17941824.


29. Rungroj N, Devonald MA, Cuthbert AW, et al. A novel missense mutation in AE1 causing autosomal dominant distal renal tubular acidosis retains normal transport function but is mistargeted in polarized epithelial cells. J Biol Chem. 2004; 279:13833–13838. PMID: 14734552.


30. Devonald MA, Smith AN, Poon JP, Ihrke G, Karet FE. Non-polarized targeting of AE1 causes autosomal dominant distal renal tubular acidosis. Nat Genet. 2003; 33:125–127. PMID: 12539048.


Table 1
Laboratory Findings of the Case of dRTA and SAO
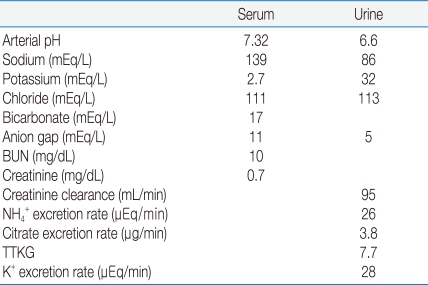
BUN, blood urea nitrogen; TTKG, transtubular potassium gradient.
*modified from the study of Kaitwatcharachai C et al. Ref.14