Abstract
Background
This study compared clinical and radiological results between robotic total knee arthroplasty (TKA) and conventional TKA with a cruciate-retaining implant at 10-year follow-up. The hypothesis was that robotic TKA would allow for more accurate leg alignment and component placement, and thus enhance clinical and radiological results and long-term survival rates.
Methods
A total of 113 primary TKAs performed using a cruciate-retaining implant in 102 patients from 2004 to 2007 were reviewed retrospectively. Of the 113 TKAs, 71 were robotic TKAs and 42 were conventional TKAs. Clinical outcomes (visual analogue scale pain score, Hospital for Special Surgery score, Western Ontario and McMaster University score, range of motion, and complications), radiological outcomes, and long-term survival rates were evaluated at a mean follow-up of 10 years.
Results
Clinical outcomes and long-term survival rates were similar between the two groups. Regarding the radiological outcomes, the robotic TKA group had significantly fewer postoperative leg alignment outliers (femoral coronal inclination, tibial coronal inclination, femoral sagittal inclination, tibial sagittal inclination, and mechanical axis) and fewer radiolucent lines than the conventional TKA group.
Total knee arthroplasty (TKA) is highly effective for pain relief and restoration of joint function in arthritic knees, providing satisfactory results in over 90% of patients.123) Soft tissue balance and mechanical alignment are crucial for the success of TKA, implant survival, and knee function among the many influential factors. Studies have shown that well-balanced knees with good alignment are an important precondition for successful TKA and prolonged implant survival.4567) Efforts to improve clinical and radiological outcomes have spurred technological advances, such as computer navigation, patient-specific cutting guides, and semicustom patient-specific implants. Robotic TKA has gained popularity among orthopedic surgeons in the operative suite because of its accuracy and precision of component placement.8) Prospective studies comparing robotic TKA to conventional TKA have revealed that robotic TKA is more accurate with less variation in the mechanical axis in spite of no difference in clinical outcomes compared to conventional TKA.910) Because the robotic approach facilitates accurate procedure without any changes in the preoperative plan, surgical exposure and reaming process are supervised by the surgeon using the computer-controlled cutting system.11)
Most previous studies comparing postoperative results between robotic TKA and conventional TKA featured short-term or intermediate-term observations.12131415) No study has compared the long-term follow-up outcomes of robotic versus conventional TKAs using the same implant. The present study examined whether robotic TKA (1) improved clinical results; (2) reduced postoperative complications; (3) reduced postoperative leg alignment outliers and radiolucent lines; and (4) provided better long-term survival rates compared to conventional TKA over an average follow-up of 10 years. The hypothesis was that robotic TKA allows for more accurate leg alignment and precise component placement, and thus enhances clinical and radiological results and long-term survival rates.
This study was designed as a retrospective, comparative trial for which Chonnam National Universtiy Hwasun Hospital Institutional Review Board (No. CNUHH-2016-137) approval was obtained. The study included 102 patients (113 knees) who underwent TAK under the diagnosis of knee osteoarthritis from January 2004 to December 2007. Inclusion criteria were mechanical axis between 20° varus and 10° valgus and primary osteoarthritis of the knee. Exclusion criteria were a previous open knee surgery, a neurological defect affecting the lower extremity, or severe instability that was not treatable by cruciate-retaining TKA.
The total 113 knees were classified into two groups: 71 knees treated using robotic TKA (robotic TKA group) and 42 knees treated using conventional TKA (conventional TKA group). Eleven patients underwent both robotic and conventional TKAs at the same time for bilateral osteoarthritis, whereas 60 patients underwent robotic TKA only and 31 patients had conventional TKA only for unilateral osteoarthritis. The robotic TKA group was composed of 5 men and 66 women with a minimum follow-up of 8 years (average, 10.5 years; range, 8.4 to 11.5 years) after TKA. In the conventional TKA group, there were 3 men and 39 women with at a minimum follow-up of 8 years (average, 10.1 years; range, 8.4 to 12.1 years) after TKA. Patients were also excluded if there was a history of previous open knee surgery for pyogenic gonitis and secondary osteoarthritis of the knee due to Charcot arthropathy. The single experienced surgeon (EKS) of our institution performed all the robotic and conventional TKAs. The NexGen, cruciate-retaining (CR) prosthesis (Zimmer, Warsaw, IN, USA) was implanted in both groups, and the ROBODOC system (Integrated Surgical Systems, Sacramento, CA, USA) was used only in the robotic TKA group.
The surgeon (EKS) used the measured technique for bone cutting and prosthesis size selection. Care was taken to make the same amount of bone and cartilage removed as the thickness of the prosthesis in order to restore the knee to the premorbid state. The surgical approach for both robotic and conventional TKAs involved a longitudinal midline incision and patellar eversion with a medial parapatellar arthrotomy extending approximately 3–4 cm into the quadriceps tendon.
The conventional TKA was performed following the manufacturer's technique using manual instruments. The femur was prepared using an intramedullary rod set at 6° of anatomic valgus without considering the preoperative femoral anatomy, and the entry point of the guide was located at an anteromedial point of attachment site of the posterior cruciate ligament. Femoral element rotation was set at 3° of external rotation to the posterior condylar axis. The tibia was prepared using an extramedullary cutting guide with the posterior slope set at 7° in the sagittal plane. Rotation of the tibial component was in line with the medial one-third of the tibial tubercle. Alignment was confirmed with extramedullary rods referenced to the anterior superior iliac spine from which the center of femoral head was about 20 mm medial and placed 5–10 mm medially from the mid spot of both malleoli.9)
The robotic TKA was a two-step process. The first step involved computed tomography (CT)-based preoperative planning using the ORTHODOC workstation (Integrated Surgical Systems) before the day of operation. The second step included robotic surgery using the ROBODOC Surgical Assistant.
For the first step, the CT scan data were linked to the ORTHODOC workstation. After making a surface model of the tibia and femur, the femoral and tibial mechanical axes were established in accordance with the anatomical centers of the ankle, hip, and knee, and the bones were aligned along these axes. The femoral component was selected and manipulated three-dimensionally using the ORTHODOC workstation with a goal of restoring the shape of the distal femur and the original premorbid size with an assumed cartilage thickness of 3 mm.10161718) The CT scans loaded on the ORTHODOC workstation were used to identify the mechanical axis of the patient's femur; these CT images were viewed in three orthogonal planes, and angular and distance measurements were performed while placing the implant template in three dimensions. The distal surface of the femoral component was designed to be perpendicular to the axis in the coronal plane. The rotational movement of the femoral component was aligned with the transepicondylar axis in the axial plane. The tibial component was selected and manipulated to fit the size and location for restoration of the articular surface of the tibia assuming a cartilage thickness of 2 mm on the least worn plateau.10161718) The tibial element was aligned vertical to a line from the center of the tibial plateau to the center of the dome of the talus. The tibial element in the axial plane was rotated and aligned with the medial one-third of the tibial tubercle. The posterior slope of the tibial element was set at 7°. The surgeon then oriented the bones to make the proximal tibial implant surface and the distal femoral surface parallel using virtual surgery to ensure restoration of the mechanical axis. The final plan was saved in a compact disc (CD) and loaded onto the ROBODOC system on the day of surgery.
The surgically-exposed knee was flexed to approximately 70° to 80° using a special leg holder during surgery. Two Steinmann and Hoffman fixation systems (Stryker Osteosynthesis, Geneva, Switzerland) were used for the femur and tibia each to fix the leg to the ROBODOC base. Two recovery markers and one bone motion monitor were installed into the femur and tibia. Surface registration and verification were carried out by the surgeon using the ROBODOC DigiMatch registration system. Afterward, as per the plan generated in the ORTHODOC station, ROBODOC milled the femur and tibia under direct control and supervision. This process was conducted very carefully so that the soft tissues were protected in the ROBODOC workspace. The ROBODOC device was eliminated from the operative area when cutting was completed.
After bone cutting, soft tissue balancing was performed in both groups. A commercial tensor device (Stryker Howmedica Osteonics Corp., Allendale, NJ, USA) was inserted with the knee in extension as described in previous studies.15192021) The medial and lateral gaps were measured, and required release was done to produce within ± 2 mm of medial-lateral gap difference. Releases were performed in a stepwise way, releasing only to the extent required to achieve balance. Extension gaps recorded with the tensor included the distance from the femur to the tibia in the middle of the gap. The knee was flexed to 90° and the flexion gap was measured and compared to the extension gap. The intent was to create a flexion gap 2 mm greater than the extension gap.22) The medial and lateral gaps were recorded (mm) at full extension and 90° of flexion after any required soft tissue release was completed.
After ligament balancing, the remainder of the procedure was conducted in all patients. The range of motion (ROM) and stability were confirmed with the trial elements inserted. The tibial insert thickness was selected and the actual implants were cemented in place.
As per the Knee Society Roentgenographic Evaluation System,23) all radiographic assessments were respectively performed by two observers (HYY and HAL). Picture Archiving and Communication Systems (PACS) digital radiographic software (Infinitt Healthcare, Seoul, Korea) was used for all the measurements. Because images could be enlarged and the software tools could provide the measurement values to the second decimal point precision, this software system was useful for precise measurements.
To determine coronal plane mechanical axis measurements using the angle between the mechanical femoral axis (line connecting the center of femoral head and center of intercondylar notch) and the mechanical axis of the tibia (line connecting the center of tibia plateau with the center of the ankle), radiographic evaluations were performed soon after surgery and at the latest follow-up using standing full-leg radiographs. Femoral component alignment was measured in the coronal plane (α, optimum, 90°) and tibial component alignment in the coronal plane (β, optimum, 90°) on an anteroposterior radiograph of the knee. The alignment was defined as the angle between the respective prosthetic position and the mechanical axis. Femoral component alignment was also measured in the sagittal plane (γ, optimum, 0°) and tibial component alignment (δ, optimum, 83°) in the sagittal plane on a lateral radiograph of the knee at the last follow-up visit. Radiological outcomes were considered acceptable when all radiographic angles were within 3° of the optimum angle, with the remaining angles regarded as outliers. A radiolucent line was considered to be progressive if it increased in size or gradually progressed from one zone to an adjacent zone. Successive radiographs were reviewed for evidence of component subsidence or change in position of greater than 2 mm and/or angular change greater than 3° compared to the surrounding bone.
Clinical assessments were performed 3, 6, and 12 months postoperatively and then yearly thereafter. Data analyses were done collectively preoperatively and at the latest follow-up by two observers (HYY and HAL) who were not involved in the surgery. Clinical outcome was assessed with regard to the Western Ontario and McMaster University (WOMAC) score, ROM, visual analogue scale (VAS) pain score, Hospital for Special Surgery (HSS) score, and any complications.
Statistical analyses were conducted using IBM SPSS ver. 20.0 (IBM Co., Armonk, NY, USA). The Kolmogorov-Smirnov (K-S) test was used to determine whether the data showed normal distribution. For postoperative VAS pain scores, HSS and WOMAC scores, and ROM, paired t-test was conducted. The incidence of outliers for each parameter was compared using chi-square test or Fisher exact test. Statistical significance was indicated by p < 0.05. The survival rate was estimated by Kaplan-Meier survival analysis using revision for any reason as the endpoint in both groups. The two groups were compared using log-rank analysis.
There was no notable difference in preoperative patient demographics including age and varus deformity between the conventional and robotic TKA groups. Preoperative HSS score, WOMAC score, VAS score, and ROM were also similar between the groups (Table 1).
No significant differences between the two groups were evident for postoperative mechanical axis alignment (robotic, 1.8°; conventional, 2.4°; p = 0.31); average femoral inclination in the coronal plane (robotic, 89.4°; conventional, 88.4°; p = 0.14) or sagittal plane (robotic, 2.0°; conventional, 3.5°; p = 0.51); and tibial inclination in the coronal plane (robotic, 90.0°; conventional, 90.2°; p = 0.06) and sagittal plane (robotic, 83.5°; conventional, 84.5°; p = 0.21) (Table 2). Concerning outliers (>|3|° from neutral), the robotic TKA group had 6 outliers for the mechanical axis, 4 for the femoral coronal axis, 1 for the tibial coronal axis, 10 for the femoral sagittal axis, and 6 for tibial sagittal inclination. The conventional TKA group had 13 outliers for the mechanical axis, 12 for the femoral coronal inclination, four for the femoral sagittal inclination, 23 for the femoral sagittal inclination, and 17 for tibial coronal inclination (Table 3). There were no radiolucent lines in the robotic TKA group. Six radiolucent lines were found in the conventional TKA group: 4 on the femoral side and 2 on the tibial side. Thickness in 5 patients was ≤ 2 mm and had no influence on implant stability. None of the radiolucent lines progressed over time. The incidence of radiolucent lines was significantly different between the groups (p < 0.001).
The average preoperative HSS score was 65.7 ± 7.7 in the robotic TKA group and 65.4 ± 6.8 in the conventional TKA group. The scores improved to 88.7 ± 10.1 and 87.7 ± 11.0 at the last follow up, respectively (p < 0.001 for preoperative versus the last follow-up in both groups). No significant intergroup differences were observed in the final follow-up HSS score (p = 0.79). The mean preoperative WOMAC score of 80.8 ± 15.4 in the robotic TKA group and 75.3 ± 16.5 in the conventional TKA group improved to 7.6 ± 9.4 and 11.5 ± 14.5 at the last follow-up, respectively (p < 0.001 for preoperative versus the last followup in both groups). No significant intergroup differences were detected in the final follow-up WOMAC score (p = 0.12). The preoperative VAS pain score of 5.5 ± 3.5 in the robotic TKA group and 5.2 ± 3.9 in the conventional TKA group improved to 1.1 ± 1.0 and 1.2 ± 1.1 at the last follow-up, respectively (p < 0.001 for preoperative versus the last follow-up for both groups) (Table 4). No significant intergroup differences were detected in the final follow-up VAS pain score (p = 0.51). The preoperative ROM of 121.7° ± 16.0° in the robotic TKA group and 122.0° ± 14.3° in the conventional TKA group was enhanced to 132.6° ± 10.5° and 131.0° ± 20.4° at the last follow up, respectively (p < 0.001 for preoperative versus last follow-up in both groups). No significant intergroup differences were observed in the final follow-up ROM (p = 0.92). There were two cases of complication (both infections) in the robotic TKA group and three cases of complication (two cases of infection and one case of instability) in the conventional group (p = 0.27).
There were five revision surgeries in both groups. Two periprosthetic joint infections occurred in the robotic TKA group. Revision surgery was performed after 1 year and 4 months in one case, and after 6 years in the other case. There were 3 revision surgeries in the conventional TKA group. Two knees were revised for infection: one at 4 years and 7 months after surgery and the other at 6 years after surgery. The other patient presented with gradually increasing knee pain when walking. Serial radiographs did not show any change in component positioning and alignment, but instability was apparent from physical examination. Revision TKA was performed at 9 years postoperatively, which revealed wear of the polyethylene liner.
Using the Kaplan-Meier survival analysis, the overall survival rate of femoral and tibial components with revision as the endpoint was estimated. Five patients underwent revision TKA at a mean of 5.3 years after surgery; record review revealed that the implants functioned well in these patients before reoperation. Revision surgery comprised the robotic technique (n = 2) and the conventional technique (n = 3). Cumulative survival in the robotic group and the conventional group was 98.5% and 97.6% at 5 years, respectively, and 97.1% and 92.3%, respectively, at 10 years. No significant difference was clearly identified between two groups using the log-rank analysis (p = 0.31) (Fig. 1).
Patients desire a reliable and durable TKA for improvement in knee function and pain relief. Postoperative alignment of TKA components affect surgical outcomes and implant longevity.72425) Robotic TKA allows for anatomic restoration with consistent and accurate postoperative leg alignment, optimized soft tissue balancing, and restoration of normal joint kinematics.1012131426) However, until now, long-term outcomes of robotic and conventional TKAs using the same implant have not been compared in a sufficient follow-up period to evaluate complications and implant survivorship.
This study was designed specifically for a comparative review of long-term survival and clinical and radiological outcomes of robotic TKA and conventional TKA using the same cruciate-retaining implant. Robotic TKA was effective in achieving the desired mechanical axis with a statistically significant reduction in the number of outliers and radiolucent lines compared to conventional TKA. In addition, the robotic technique produced better clinical and radiological outcomes than the conventional approach, although the long-term implant survival rate was similar after an average follow-up of 10 years.
The mechanical axis improved from 9.0° varus to 1.9° varus in the robotic TKA group, and from 10.0° varus to 2.9° varus in the conventional TKA group. Concerning the postoperative leg alignment outliers, although extramedullary alignment guides and intramedullary rods were used for component alignment, conventional TKA produced considerably more outliers than robotic TKA. This result echoes prior studies.1012131426) The robotic system enables surgeons to carry out a more accurate and precise procedure using a more patient-specific plan based on CT scans. More radiolucent lines were evident following conventional surgery, but none influenced implant stability.
The clinical data showed typical outcomes of TKA. The postoperative scores of the robotic group (HSS, 88.7; WOMAC, 7.6; ROM 132.6°) and the conventional group (HSS, 87.2; WOMAC, 11.5; ROM 131.0°) were similar to those previously reported following robotic TKA (HSS, 95.2; WOMAC, 11; ROM 129°) and conventional TKA (HSS, 90.6; WOMAC, 32.2; ROM 129°).910) This implies that both techniques produced comparably good clinical results as measured by the aforementioned parameters; however, it was not determined if clinical outcomes were of any significance.
Infections developed in the early or medium-term postoperative period in both the robotic and conventional TKA groups. Although the mean operative time may be longer with the robotic system9) and some studies reported that increased operative time is related to increased risk of infection,27) infection is not thought to be directly related to the difference in technique and operative time (≤ 30 minutes). Revision TKA in the robotic TKA group was exclusively due to infection, while one revision in the conventional TKA group was because of polyethylene wear. A study that analyzed outcomes after TKA in 164 patients with an average overall alignment of 3° reported that limb malalignment influenced survivorship and outcomes after TKA.2) Another study reviewed 3,992 patients to determine the effect of limb alignment on failure of TKA: attaining neutral limb alignment was important in implant survival.28) As mentioned above, many studies reported that a mechanical axis malalignment of greater than 3° after TKA could bring worse clinico-radiological outcomes.242728) Therefore, the accuracy of implantation influences patient outcomes in terms of knee function and implant survival. In the present study, 1 patient in the robotic TKA group experienced medial retinacular rupture during surgery and showed increasing discomfort in the treated knee and patellar subluxation during follow-up. This patient received medial retinacular repair 2 years after surgery. Despite improved accuracy, the robotic system is designed to execute a specific plan and lacks the ability to alter the plan for an unexpected injury. This limitation warrants further scrutiny.
This study has several limitations. This is a retrospective study with the usual issues of potential inaccuracy of medical records and information bias. However, we did not include clinical scoring systems, such as the Knee Society clinical rating29) or Oxford Knee score, in the analysis.30) Second, the extent of femoral rotation was different between the two groups: the femoral component was lined up with the transepicondylar axis in the robotic TKA group whereas 3° of external rotation to the posterior condylar axis was used in the conventional TKA group considering the difficulty of performing transepicondylar axis surgery in conventional TKA. The axis can be identified on most CT scans, but it is difficult for surgeons to identify manually during surgery. Thus, using a fixed 3° rotation alignment in the conventional TKA group might have resulted in an inaccurate component position. Also, the relatively small number of patients could have weakened the power of the analyses. Third, in order to improve the accuracy of radiographic measurement, CT should have been used especially for evaluation of femoral component alignment in the sagittal plane. The difficulty of radiographic measurement of γ angle may have caused the differences and acted as a confounding factor.
Robotic and conventional TKAs provided good clinical outcomes and postoperative leg alignments. Robotic TKA was more effective in achieving the desired mechanical axis with a reduced number of outliers and fewer radiolucent lines than conventional TKA. Robotic TKA produced better long-term implant survival although the difference was not statistically significant.
ACKNOWLEDGEMENTS
This work was supported by a research grant from the Research Institute of Medical Sciences, Chonnam National University (No. 2010-CURIMS-DR004).
References
1. Laskin RS. The Genesis total knee prosthesis: a 10-year followup study. Clin Orthop Relat Res. 2001; (388):95–102.
2. Rodriguez JA, Bhende H, Ranawat CS. Total condylar knee replacement: a 20-year followup study. Clin Orthop Relat Res. 2001; (388):10–17.
3. Scott WN, Rubinstein M, Scuderi G. Results after knee replacement with a posterior cruciate-substituting prosthesis. J Bone Joint Surg Am. 1988; 70(8):1163–1173. PMID: 3417701.


4. Griffin FM, Insall JN, Scuderi GR. Accuracy of soft tissue balancing in total knee arthroplasty. J Arthroplasty. 2000; 15(8):970–973. PMID: 11112189.


5. Laskin RS, Rieger MA. The surgical technique for performing a total knee replacement arthroplasty. Orthop Clin North Am. 1989; 20(1):31–48. PMID: 2919077.
6. Ritter MA, Faris PM, Keating EM, Meding JB. Postoperative alignment of total knee replacement: its effect on survival. Clin Orthop Relat Res. 1994; (299):153–156. PMID: 8119010.


7. Takahashi T, Wada Y, Yamamoto H. Soft-tissue balancing with pressure distribution during total knee arthroplasty. J Bone Joint Surg Br. 1997; 79(2):235–239. PMID: 9119849.


8. Jacofsky DJ, Allen M. Robotics in arthroplasty: a comprehensive review. J Arthroplasty. 2016; 31(10):2353–2363. PMID: 27325369.


9. Song EK, Seon JK, Park SJ, Jung WB, Park HW, Lee GW. Simultaneous bilateral total knee arthroplasty with robotic and conventional techniques: a prospective, randomized study. Knee Surg Sports Traumatol Arthrosc. 2011; 19(7):1069–1076. PMID: 21311869.


10. Song EK, Seon JK, Yim JH, Netravali NA, Bargar WL. Robotic-assisted TKA reduces postoperative alignment outliers and improves gap balance compared to conventional TKA. Clin Orthop Relat Res. 2013; 471(1):118–126. PMID: 22669549.


11. Matsen FA 3rd, Garbini JL, Sidles JA, Pratt B, Baumgarten D, Kaiura R. Robotic assistance in orthopaedic surgery: a proof of principle using distal femoral arthroplasty. Clin Orthop Relat Res. 1993; (296):178–186.
12. Decking J, Theis C, Achenbach T, Roth E, Nafe B, Eckardt A. Robotic total knee arthroplasty: the accuracy of CT-based component placement. Acta Orthop Scand. 2004; 75(5):573–579. PMID: 15513489.
13. Park SE, Lee CT. Comparison of robotic-assisted and conventional manual implantation of a primary total knee arthroplasty. J Arthroplasty. 2007; 22(7):1054–1059. PMID: 17920481.


14. Song EK, Jung WB, Yoon TR, Park KS, Seo HY, Seon JK. Comparison of outcomes after bilateral simultaneous total knee arthroplasty using gender-specific and unisex knees. J Arthroplasty. 2012; 27(2):226–231. PMID: 21704482.


15. Asano H, Hoshino A, Wilton TJ. Soft-tissue tension total knee arthroplasty. J Arthroplasty. 2004; 19(5):558–561. PMID: 15284975.


16. Adam C, Eckstein F, Milz S, Putz R. The distribution of cartilage thickness within the joints of the lower limb of elderly individuals. J Anat. 1998; 193(Pt 2):203–214. PMID: 9827636.
17. Ateshian GA, Soslowsky LJ, Mow VC. Quantitation of articular surface topography and cartilage thickness in knee joints using stereophotogrammetry. J Biomech. 1991; 24(8):761–776. PMID: 1918099.


18. Shepherd DE, Seedhom BB. Thickness of human articular cartilage in joints of the lower limb. Ann Rheum Dis. 1999; 58(1):27–34. PMID: 10343537.


19. Sasanuma H, Sekiya H, Takatoku K, Takada H, Sugimoto N. Evaluation of soft-tissue balance during total knee arthroplasty. J Orthop Surg (Hong Kong). 2010; 18(1):26–30. PMID: 20427829.


20. Sekiya H, Takatoku K, Takada H, Sasanuma H, Sugimoto N. Postoperative lateral ligamentous laxity diminishes with time after TKA in the varus knee. Clin Orthop Relat Res. 2009; 467(6):1582–1586. PMID: 18941848.


21. Winemaker MJ. Perfect balance in total knee arthroplasty: the elusive compromise. J Arthroplasty. 2002; 17(1):2–10.


22. Sugama R, Kadoya Y, Kobayashi A, Takaoka K. Preparation of the flexion gap affects the extension gap in total knee arthroplasty. J Arthroplasty. 2005; 20(5):602–607. PMID: 16309995.


23. Ewald FC. The Knee Society total knee arthroplasty roentgenographic evaluation and scoring system. Clin Orthop Relat Res. 1989; (248):9–12.


24. Jeffery RS, Morris RW, Denham RA. Coronal alignment after total knee replacement. J Bone Joint Surg Br. 1991; 73(5):709–714. PMID: 1894655.


25. Saragaglia D, Picard F, Chaussard C, Montbarbon E, Leitner F, Cinquin P. Computer-assisted knee arthroplasty: comparison with a conventional procedure: results of 50 cases in a prospective randomized study. Rev Chir Orthop Reparatrice Appar Mot. 2001; 87(1):18–28. PMID: 11240534.
26. Fadda M, Marcacci M, Toksvig-Larsen S, Wang T, Meneghello R. Improving accuracy of bone resections using robotics tool holder and a high speed milling cutting tool. J Med Eng Technol. 1998; 22(6):280–284. PMID: 9884932.


27. Peersman G, Laskin R, Davis J, Peterson MG, Richart T. Prolonged operative time correlates with increased infection rate after total knee arthroplasty. HSS J. 2006; 2(1):70–72. PMID: 18751850.


28. Bathis H, Perlick L, Tingart M, Perlick C, Luring C, Grifka J. Intraoperative cutting errors in total knee arthroplasty. Arch Orthop Trauma Surg. 2005; 125(1):16–20. PMID: 15538589.


29. Insall JN, Dorr LD, Scott RD, Scott WN. Rationale of the Knee Society clinical rating system. Clin Orthop Relat Res. 1989; (248):13–14. PMID: 2805470.


30. Xu L, J Dibley M, D'Este C. Reliability and validity of a food-frequency questionnaire for Chinese postmenopausal women. Public Health Nutr. 2004; 7(1):91–98. PMID: 14972077.


Fig. 1
Survival analysis of robotic (red line) and conventional (blue line) total knee arthroplasty.
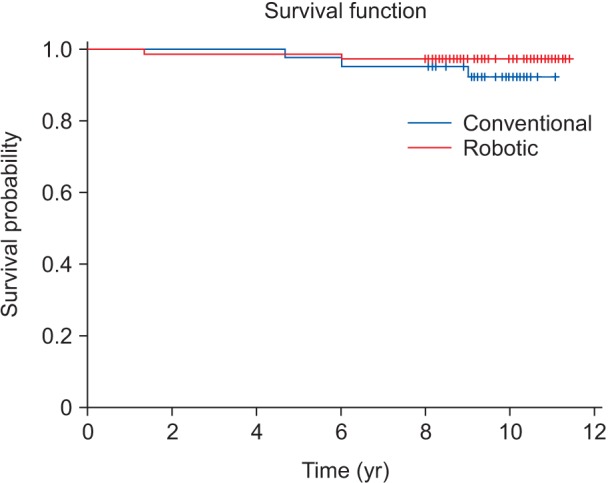
Table 1
Comparison of Preoperative Demographic Data
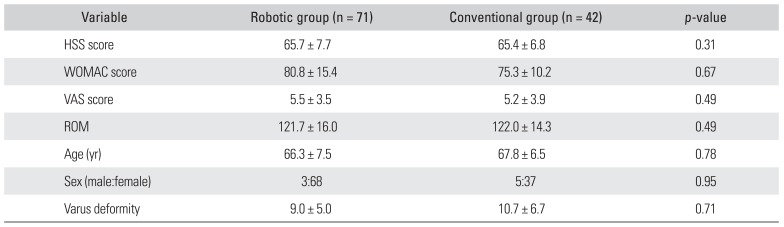
Table 2
Comparison of Average Values of Radiographic Results between Robotic and Conventional Total Knee Arthroplasty Groups
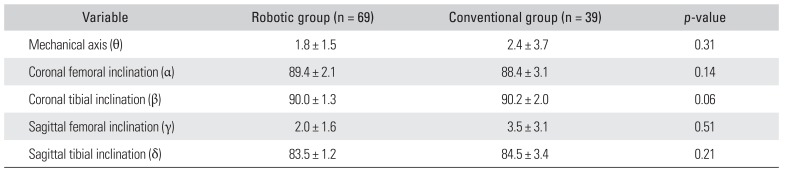
Table 3
Comparison of Radiographic Outliers between Robotic and Conventional Total Knee Arthroplasty Groups
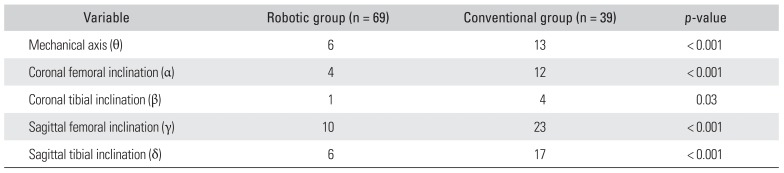