Abstract
Background
The purpose of this study was to analyze biomechanical properties of a novel wedge locking plate in medial open wedge high tibial osteotomy (OWHTO) in a porcine tibial model.
Methods
A uniform 8-mm OWHTO was performed in 12 porcine tibiae. Six of them were subsequently fixed with the plate without a wedge, whereas the other 6 were additionally reinforced with a metal wedge of 8 mm. Biomechanical properties (stiffness, displacement of the osteotomy gap, and failure load) were evaluated under axial load. The different modes of failure were also investigated.
Results
The plate showed an axial stiffness of 2,457 ± 450 N/mm with a wedge and 1,969 ± 874 N/mm without a wedge. The maximum failure load was 5,380 ± 952 N with a wedge and 4,354 ± 607 N without a wedge. The plate with a wedge had a significantly greater failure load and significantly less displacement of medial gap at failure than that without a wedge (p = 0.041 and p = 0.002, respectively). The axial stiffness was not different between the two types of fixation. Most failures were caused by lateral cortex breakage and there was no implant failure.
Medial open wedge high tibial osteotomy (OWHTO) is a well-established method for the treatment of medial unicompartmental osteoarthritis of the knee, which has shown good mid- and long-term results.1) Several factors for good results have been suggested, including the correct indication, adequate correction angle, standardized rehabilitation protocols, and the quality of internal fixation.23) Among these, there has been a recent increase in the interest of fixation methods.
Several types of internal fixation devices have been used for OWHTO, including conventional plates, spacer plates, angular stable plates, etc.4) However, the spacer plate, i.e., Puddu plate, has been reported to have marginally sufficient strength to withstand the axial load on the proximal tibia.56) TomoFix locking plate (Synthes, Oberdorf, Switzerland) is a locking plate that has been widely used and provided good stability with multiple locking screws; however, application of the device can sometimes be challenging for Asian patients with smaller tibial bones due to the relatively large profile of the plate.78)
For patients with small tibiae that require considerable correction angle, a smaller locking plate with a similar strength of TomoFix would be necessary. For this purpose, we devised a novel rigid plate that has a short length (112 mm vs. 115 mm) and smaller profile (85 mm, 3-hole type vs. 95 mm, 4-hole type) than TomoFix by combining the advantages of locking plates and wedge blocks.
The novel plate is equipped with semi-locking threaded plate holes, which can provide both good stability and adjustable screw orientation. Most of all, the distinguishable feature of this plate is it can be assembled with metal wedges of various sizes for the provision of stability and feasibility of surgical procedure. The aim of this study is to evaluate the biomechanical properties of the novel plate and to investigate biomechanical advantages of a metal wedge. The hypotheses of this research were the plate has good biomechanical properties compared to other fixation methods available and a metal wedge can enhance biomechanical properties of the plate.
The novel plate (TDM, Seoul, Korea) is a T-shaped precontoured locking plate. The plate has 8 (or 9) holes, each of which has a thread to lock the screw to the plate. Proximal 4 holes are for metaphyseal fixation, distal 3 (or 4) holes are for diaphyseal fixation, and one hole in the middle is for assembling a metal wedge. The distal holes are 3 or 4 for the purpose of choosing an adequate plate size for individual patient during surgery. This middle hole is located on a small arm directed to the posterior side of the plate, which can be placed at the most posterior part of the osteotomy gap. The metal wedge is available in different sizes from 5 mm to 16 mm with an increment of 1 mm according to the osteotomy gap. Proximal holes have semi-locking fashion threads to allow for adjustment of directions of the screws according to the bone geometry during surgery (Fig. 1).
The study involved 1-year-old female domestic pigs whose tibiae had been stored at −18℃ before use. A total of 12 specimens were used in the study and they were divided into 2 groups of 6 specimens each. The first group was subjected to fixation using the plate only and the second group had an additional reinforcement with a metal wedge. The specimens were thawed to room temperature for at least 24 hours before use.
All the specimens were subjected to a medial open wedge osteotomy using an opening wedge of 8 mm. At first, an osteotomy was done on the anterior third of the tibial cortex involving the tibial tuberosity fragment in the frontal plane which was at an angle of 110° to the axial plane. Subsequently, a K-wire of 1.6 mm in size was drilled at a distance of 4 cm from the medial joint line and directed towards the tip of the fibula. The confirmation of the direction was done with the K-wire being drilled through the opposite cortex and just touching the tip of the fibula. An oscillating saw was then used with the blade placed just above the K-wire. The osteotomy was then performed along the medial tibial cortex along the direction of the K-wire from the posterior cortex to the anterior. The osteotomy was stopped at 1 cm from the lateral tibial cortex, which was marked before surgery with a waterproof marker, thus leaving a hinge for the opening of the osteotomy. The osteotomy gap was carefully opened by the 3 chisel technique aided by a valgus force being imparted to the distal fragment. The gap was measured and once confirmed to be 8 mm, then plate fixation was carried out. The 7-hole plate was used for all specimens. In the first group, fixation of the plate was performed using 4 proximal (3 transverse and 1 longitudinal) unicortical and 3 distal bicortical locking screws while the gap was not bridged with any wedge. In the second group, prior to the plate insertion, the osteotomy gap was bridged with an 8 mm metal wedge, which was introduced in the posterior 1/3 of the osteotomy space and fixed to the plate with a screw. Subsequently, the plate was fixed to the bone in the same manner as in the first group. In both groups, all screws were fixed using a sleeve guide in order to place the screws in the same direction.
The specimen tibiae with the constructs were mounted on the universal servo-hydraulic testing machine (MTS858 Bionix; MTS System Co., Eden Prairie, MN, USA) (Fig. 2). The major parameters for evaluation were axial stiffness of the plate, displacement within the osteotomy space, and the maximum resistance to failure load in both groups. The tibiae were mounted in a specially designed fixture that was connected to the MTS machine and aligned for the mechanical axis to pass through a point located 60%–70% lateral to the medial edge of the specimen. For assessment of displacement within the space, a mark was made on the ventral and dorsal sides of both proximal and distal tibial surfaces with a waterproof marker (Fig. 3).
The load was applied on the tibia from 0 up to 1,000 N at the rate of 5 mm/sec and maintained for 1 minutes. This cycle was repeated 20 times. The stiffness of the plate was measured as was the displacement within the osteotomy space in the 1st and 20th cycles.3) Finally, 6 specimens from each group were subjected to the maximum resistance to failure load at the rate of 5 mm/min. Failure was defined as a visible fracture of the lateral tibial cortex with or without fissuring of the lateral tibial plateau and/or a fall of the load to 30% of the maximum load.
Four markers (φ = 0.5 mm, stainless steel, pin type) were used for measurement of gap opening. Two markers were for measurement of the medial gap, which were placed on the superior and inferior borders of the wedge gap medial to the plate and the other two were for the lateral gap measurement, which were also placed on the superior and inferior borders lateral to the plate (Fig. 3). Three-dimensional (3D) movements of the gap before and after loading sequences were assessed in a stereo-photogrammetric setup with two digital cameras (IXUS 200IS; Cannon, Tokyo, Japan). This is a method capable of determining 3D coordinates of the markers in space. It uses the method of direct linear transformation and stereo-photogrammetry based on two images of the markers that were taken simultaneously by two cameras. The two digital cameras were oriented at 60° to the markers to form an isosceles triangle. The laboratory coordinate system was established through a calibration procedure with a specially-constructed calibration frame. MATLAB (MathWorks, Natick, MA, USA) software was used for measurement of 3D displacement. The 3D movement of the markers, or the gap between the markers, was assessed based on changes in the 3D coordinates of the markers before and after loading sequences (resolution, 0.01 m).
The Mann-Whitney U-test was used for comparison of the outcomes (stiffness, failure load, posteromedial and anterolateral gap) between the two groups. The sample size, 6 specimens in each group, was calculated based on the failure load, using a significance level of 5% and a test power of 80%, as described in a previous publication.9)
Stiffness of the plate without a metal wedge was 454 N/mm in the 1st cycle and 1,969 N/mm in the 20th cycle. Stiffness of the plate with a metal wedge was 490 N/mm in the 1st cycle and 2,457 N/mm in the 20th cycle. There were no significant differences between the groups in both the 1st and 20th cycles (Table 1).
The mean yield load was 2,997 N without a wedge and 3,221 N with a wedge, but the difference was not statistically significant. The mean maximal load at failure was 4,354 N without a wedge and 5,380 N with a wedge. With a spacer, there was a significant improvement of the maximal failure load (p < 0.05) (Table 1).
Under the 1,000 N axial load, the displacement measured at the posteromedial gap was 0.79 mm without a wedge and 0.62 mm with a wedge, indicating no significant difference. However, at the failure load, there was significant difference between the group with a wedge and the group without a wedge (1.41 mm vs. 0.97 mm; p < 0.05) (Fig. 4). Regarding the displacement measured at the anterolateral gap, it was 0.80 mm and 0.99 mm, respectively, under 1,000 N and 2.86 mm and 3.49 mm, respectively at failure load for the group without a wedge and the group with a wedge; the differences were not significant under 1,000 N axial load and at failure load (Table 1).
Regardless of the use of a wedge, lateral cortical fracture was observed. However, screw or plate disengagement was not observed in both groups (Table 2).
The novel plate was developed to provide stable internal fixation after OWHTO. It is designed to have a small profile and short length compared to the TomoFix plate. The present study has shown that the novel plate has good mechanical properties with great initial fixation strength. In addition, a metal wedge enhanced the mechanical properties of the plate.
Medial opening wedge osteotomy always carries the risk of loss of correction due to the inherent primary instability caused by the gap creation. Strong primary fixation is essential not only for bony healing but also for early rehabilitation involving weight bearing and range of motion exercises. In the present study, the maximum load to failure of the novel plate was 5,480 N, which is much greater than the axial compressive load applied on the adult knees in the single-leg stance in normal and brisk walking.101112) Kuster et al.11) reported that the estimates of the tibiofemoral bone-on-bone contact forces were close to four times the body weight even during level walking and more than eight times the body weight during downhill walking. For example, a 60-kg person would have axial compressive load about 2,400 N during level walking and 4,800 N during downhill walking, which are below the maximum load to failure of the novel plate. In addition, based on the study by Morrison,12) the biomechanical properties of the novel plate exceed those of human legs in normal walking.
The new plate also demonstrated performance superior or comparable to that of other plates.34) Agneskirchner et al.4) compared different plates: a short spacer plate with multi-directionally insertable screws displayed greater maximum load to failure (3,226 N) than a short spacer plate without a locking screw (2,992 N) and a rigid long medial tibial plate fixator with locking screws (3,069 N). In comparison, the maximal load to failure of our plate was much greater (4,354 N with a wedge and 5,480 N without a wedge). Another biomechanical study on Puddu plate and TomoFix plate using artificial bones described the axial stiffness of the Puddu plate as 1,349 N/mm and that of the TomoFix plate as 1,701 N/mm. In comparison, the axial stiffness of our plate was much greater (1,969 N/mm without a wedge and 2,457 N/mm with a wedge).13)
The 3D measurement system was used to measure the displacement of osteotomy gap. Medial and lateral side displacements at failure load without and with a wedge were only 1.41 mm and 0.97 mm, and 2.86 mm and 3.49 mm, respectively. These results are comparable to or better than those of previous studies.34) There was a significant difference in medial displacement at failure load between the two modalities of fixation. Locking mechanism of the plate allowed screws to hold strongly to the metaphysis of the tibia thus displaying good properties to resist the axial load and to maintain the osteotomy gap. Additional fixation provided by the metal wedge enhanced stability of the construct.
A wedge spacer is known to be able to take up compressive forces within the osteotomy gap. Spahn et al.3) reported that a wedge spacer improved the biomechanical stability in their porcine tibial model. In the study, however, the plate was for non-locking thread screws, thus the effect of the wedge spacer was considerable compared to our study. In the current study, there were significant differences in maximal failure load and displacement at the posteromedial gap depending on the use of a wedge. This implies that even in the locking plate, additional wedge can have an effect of taking up compressive forces and reducing displacement of the osteotomy gap. There could be a vacant space of the wedge spacer after removal of the plate after osteotomy has healed. However, the size is so small compared to the whole tibial plate that it does not affect the stability whether it is filled with allograft bone or not. If necessary, it can be filled with allograft or other substitution material.
Porcine tibiae were used for the current study because it has been demonstrated that the average density of porcine bone is similar to that of young human bone.14) Synthetic bones are used in many biomechanical studies of HTO and they are homogenous in bone quality and minimize experimental errors caused by specimen-to-specimen variability in bone mineral density and geometry; however, they do not truly represent the real bone.15)
In terms of clinical use, the novel plate has a small profile and shorter length so that it is easy to implant and causes less mechanical irritation after implantation. Plates developed in the Western countries are sometimes difficult to utilize in Asian people because of the relatively small size of the tibia. A metal wedge is another big plus of this plate. Maintenance of the osteotomy gap during surgery has been considered a challenge, but it can be conveniently overcome by the insertion of a metal wedge and subsequent fixation to the implant with a screw. In addition, the proximal holes are designed to allow 15° of freedom for screw movement and a mobile metal wedge can be inserted in the osteotomy gap. The present study has some limitations. First, all measurements were performed at the time zero point; therefore, the obtained values do not reflect the biomechanical properties at multiple time points. Second, only axial load perpendicular to the tibial plateau was applied to the specimen and torsional rigidity was not tested. Third, all soft tissues were removed during the preparation of the specimen; soft tissues around joints have a stabilizing effect as well as provide a completely different environment. Fourth, the osteotomy gap was made to be 8 mm only and only the 8-mm metal wedge was used; more than 8-mm osteotomy gap was not able to be created due to the small size of the porcine tibia. Fifth, porcine tibiae were used in the present study; their biomechanical properties and the geometry are not completely identical to those of the human tibiae.
In conclusion, the novel locking wedge plate showed good biomechanical properties and the use of an additional wedge resulted in significant enhancement of the biomechanical properties. This plate can be considered a good fixation method in OWHTO.
References
1. Saito T, Kumagai K, Akamatsu Y, Kobayashi H, Kusayama Y. Five- to ten-year outcome following medial opening-wedge high tibial osteotomy with rigid plate fixation in combination with an artificial bone substitute. Bone Joint J. 2014; 96(3):339–344. PMID: 24589788.


2. Floerkemeier S, Staubli AE, Schroeter S, Goldhahn S, Lobenhoffer P. Outcome after high tibial open-wedge osteotomy: a retrospective evaluation of 533 patients. Knee Surg Sports Traumatol Arthrosc. 2013; 21(1):170–180. PMID: 22744433.


3. Spahn G, Muckley T, Kahl E, Hofmann GO. Biomechanical investigation of different internal fixations in medial opening-wedge high tibial osteotomy. Clin Biomech (Bristol, Avon). 2006; 21(3):272–278.


4. Agneskirchner JD, Freiling D, Hurschler C, Lobenhoffer P. Primary stability of four different implants for opening wedge high tibial osteotomy. Knee Surg Sports Traumatol Arthrosc. 2006; 14(3):291–300. PMID: 16284740.


5. Schroter S, Gonser CE, Konstantinidis L, Helwig P, Albrecht D. High complication rate after biplanar open wedge high tibial osteotomy stabilized with a new spacer plate (Position HTO plate) without bone substitute. Arthroscopy. 2011; 27(5):644–652. PMID: 21663721.
6. Nelissen EM, van Langelaan EJ, Nelissen RG. Stability of medial opening wedge high tibial osteotomy: a failure analysis. Int Orthop. 2010; 34(2):217–223. PMID: 19189104.


7. Jung WH, Chun CW, Lee JH, Ha JH, Kim JH, Jeong JH. Comparative study of medial opening-wedge high tibial osteotomy using 2 different implants. Arthroscopy. 2013; 29(6):1063–1071. PMID: 23623294.


8. Valkering KP, van den Bekerom MP, Kappelhoff FM, Albers GH. Complications after tomofix medial opening wedge high tibial osteotomy. J Knee Surg. 2009; 22(3):218–225. PMID: 19634725.


9. Han SB, Bae JH, Lee SJ, et al. Biomechanical properties of a new anatomical locking metal block plate for opening wedge high tibial osteotomy: uniplane osteotomy. Knee Surg Relat Res. 2014; 26(3):155–161. PMID: 25229045.


10. Andriacchi TP, Andersson GB, Fermier RW, Stern D, Galante JO. A study of lower-limb mechanics during stair-climbing. J Bone Joint Surg Am. 1980; 62(5):749–757. PMID: 7391098.


11. Kuster MS, Wood GA, Stachowiak GW, Gachter A. Joint load considerations in total knee replacement. J Bone Joint Surg Br. 1997; 79(1):109–113. PMID: 9020457.


12. Morrison JB. The mechanics of the knee joint in relation to normal walking. J Biomech. 1970; 3(1):51–61. PMID: 5521530.


13. Stoffel K, Stachowiak G, Kuster M. Open wedge high tibial osteotomy: biomechanical investigation of the modified Arthrex Osteotomy Plate (Puddu Plate) and the TomoFix Plate. Clin Biomech (Bristol, Avon). 2004; 19(9):944–950.


14. Tetsumura S, Fujita A, Nakajima M, Abe M. Biomechanical comparison of different fixation methods on the tibial side in anterior cruciate ligament reconstruction: a biomechanical study in porcine tibial bone. J Orthop Sci. 2006; 11(3):278–282. PMID: 16721530.


15. Yoo JH, Seong SC, Lee S, et al. Rigid stepped plate for internal fixation for high tibial osteotomy. Orthopedics. 2009; 32(10):DOI: 10.3928/01477447-20090818-08.


Fig. 2
Testing model mounted on MTS (MTS 858 Bionix; MTS System Co.). A prosthesis for total knee replacement was used for the axial load. The specimen was embedded in polyurethane casting resin and mounted in a fixture.
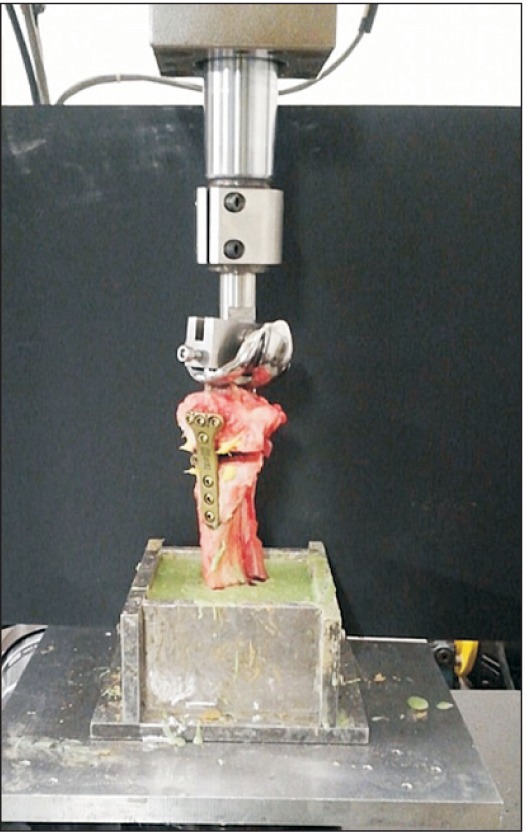
Fig. 3
Schematic drawing of four markers for the displacement measurement (ventral and dorsal sides of both proximal and distal tibial surfaces). 1 and 2 are for posteromedial gap and 3 and 4 are for anterolateral gap.
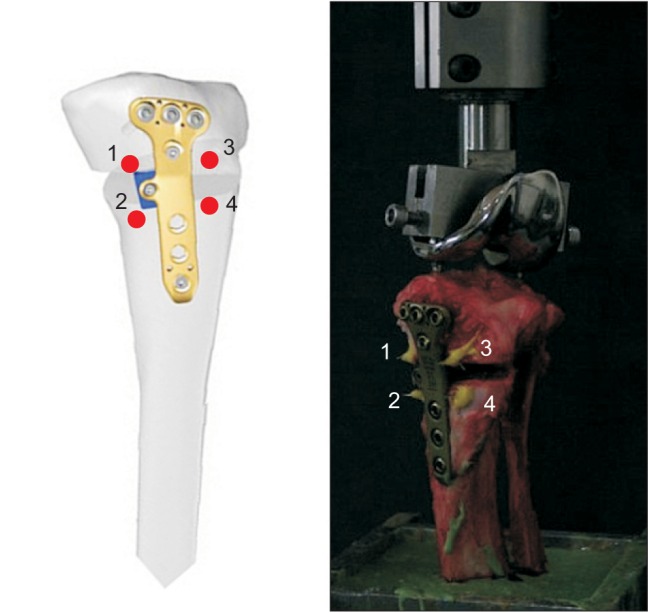
Fig. 4
A graph for the medial gap displacement. At failure load, significant difference was shown between two groups.

Table 1
Biomechanical Properties of the Plate
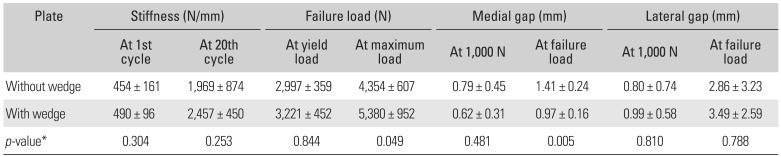