This article has been
cited by other articles in ScienceCentral.
Abstract
Background
There have been few reports on altered kinematics of the shoulder after reverse total shoulder arthroplasty (RTSA). We investigated differences in 3-dimensional (3D) scapular motions assessed using an optical tracking system between RTSA treated shoulders and asymptomatic contralateral shoulders during arm motion.
Methods
Thirteen patients who underwent RTSA were assessed for active arm elevation in 2 distinct elevation planes (sagittal plane flexion and scapular plane abduction). Their mean age was 72 years (range, 69 to 79 years) and the mean follow-up was 24.4 months (range, 13 to 48 months). The dominant side was the right side in all the 13 patients, and it was also the side treated with RTSA. Scapular kinematics was recorded with an optical tracking system. The scapular kinematics and the scapulohumeral rhythm (SHR) of the RTSA shoulders and asymptomatic contralateral shoulders were recorded and analyzed during arm elevation.
Results
There were no significant differences in internal/external rotation and anterior/posterior tilting of the scapula between shoulders during arm motion (p > 0.05). However, upward rotation of the scapula differed significantly during arm motion (p = 0.035 for sagittal plane flexion; p = 0.046 for scapular plane abduction). There were significant differences in the SHR between the two shoulders (p = 0.016 for sagittal plane flexion; p = 0.021 for scapular plane abduction).
Conclusions
The shoulder kinematics after RTSA showed significant differences from the contralateral asymptomatic shoulders. Increased upward rotation and decreased SHR after RTSA indicate that RTSA shoulders use more scapulothoracic motion and less glenohumeral motion to elevate the arm.
Go to :

Keywords: Shoulder, Reverse total shoulder arthroplasty, 3D scapular motions, Scapulohumeral rhythm, Optical tracking system
Rotator cuff tear arthropathy is characterized by massive rotator cuff tears in addition to various structural changes in the glenohumeral joint.
1) Patients with this condition may experience shoulder pain, inability to elevate the arm, and proximal migration of the humeral head, which restrict normal activities of daily living. Reverse total shoulder arthroplasty (RTSA) was introduced as a successful procedure in these patients for relief of shoulder pain and discomfort and resumption of normal daily tasks.
234567) Afterwards, the indications for RTSA have expanded to include fracture sequelae, instability, and post-cancer surgery repair along with revision arthroplasty.
89)
Scapulohumeral rhythm (SHR) described by Codman
10) is the kinematic interaction between the scapula and the humerus, and the normal SHR ratios range from 1.35:1 to 7.9:1.
1112131415161718) This interaction is important for the dynamic motion of the shoulder complex, and it is a sensitive measure of shoulder dysfunction.
19202122) Therefore, a number of studies have reported altered shoulder kinematics including SHR in the RTSA shoulders.
19202122) However, there is a paucity in the literature on 3-dimensional (3D) scapular motions consisting of upward/downward rotation, anterior/posterior tilting, and internal/external rotation in patients with RTSA.
The primary purpose of this study was to evaluate the dynamic 3D scapular motions in addition to the SHR in the RTSA and contralateral shoulders during dynamic arm motion. Our hypothesis was that there would be differences in scapular motions between the RTSA and contralateral shoulders during arm motion.
METHODS
Approval for the present study was obtained from Eulji University Hospital Institutional Review Board. Twenty-nine patients with RTSA (28 females and 1 male) participated in our study. All the patients underwent primary RTSA using Aequalis Reverse Shoulder Prosthesis (Tornier, Montbonnot-Saint-Martin, France) for rotator cuff tear arthropathy and had no revision history. Inclusion criteria were RTSA surgery for at least 1 year before evaluation of the scapular motions, painless active shoulder elevation to at least 120° in the RTSA shoulder, and the asymptomatic contralateral shoulder. The exclusion criteria were any previous history of fractures or revisions of both shoulders. The definition of asymptomatic contralateral shoulder was determined as no medical history or pain in the contralateral shoulder within the previous 12 months; no restriction in range of motion (ROM) in the contralateral shoulder; and absence of shoulder pain in clinical examination and abnormality in plain radiography in the contralateral shoulder. All evaluations were performed by an experienced shoulder surgeon who was otherwise not involved in the study.
Of the original 29 patients initially enrolled in the study, 12 patients were excluded upon a clinical examination due to pain or radiologic abnormalities in the contralateral shoulder. In addition, 4 patients were lost to follow-up. Ultimately, 13 patients (12 females and 1 male) were included in the study. Their average age was 72 years (range, 69 to 79 years) at the time of evaluation that was performed at an average of 24.4 months (range, 13 to 48 months) after RTSA. In the 13 patients, the RTSA shoulder was the dominant right side.
All the operations were performed by one senior surgeon with the patient placed in the beach chair position under general anesthesia following interscalene plexus block. The standard deltopectoral approach was used in all patients. The glenoid components were placed inferior to the glenoid surface with no inferior or superior inclination. In 11 shoulders, a 25-mm glenosphere was implanted, and in 2 shoulders, a 36-mm glenosphere was used. The humeral components had all been placed in 10° to 20° of retroversion. In all shoulders, the teres minor muscle was still intact and the infraspinatus muscle was completely ruptured. In all patients, the inferior two-thirds of the subscapularis muscle were still intact and reattached at the end of the procedure.
Postoperative management was the same for all patients. For 3 postoperative weeks, an abduction brace was used and only pendulum-type exercises were allowed. At 3 weeks after surgery, passive ROM exercises were initiated carefully beginning with forward flexion exercises. At 6 weeks after surgery, active assisted exercises were performed. Active ROM exercises and strength training exercises were started at 12 weeks after surgery.
The 3D scapular motions and humeral elevation relative to the trunk were recorded using the optical tracking system (Motion Analysis Co., Santa Rosa, CA, USA). This system allowed for 120-Hz data capture with 6 synchronized infrared cameras placed circumferentially around the patients, and this system needed at least 3 noncollinear reflective markers on each bone for data collection (
Fig. 1A).
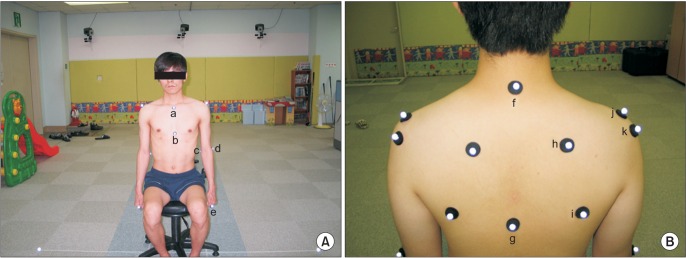 | Fig. 1Gross photographs showing reflective markers on bony landmarks and synchronized infrared cameras. (A) The photograph shows reflective markers on the anterior aspect of the chest wall and upper extremities. a: jugular notch of the sternum, b: xiphoid process of the sternum, c: medial epicondyle of the humerus, d: lateral epicondyle of the humerus, e: nail of the thumb. (B) The photograph shows reflective markers on the posterior aspect of the chest wall and upper extremities. f: spinous process of the seventh cervical vertebra, g: spinous process of the eighth thoracic vertebra, h: base of the scapular spine, i: inferior angle of the scapula, j: midpoint between the most anterosuperior aspect of the acromioclavicular joint and the angle of the acromion, k: the angle of the acromion. The scapular segment was created using "h," "j," and "k" markers.
|
Using the technique described in our previous study,
18) we made the scapular segment to estimate the dynamic motions of the scapula using (1) the midpoint between the most anterosuperior aspect of the acromioclavicular joint and the angle of acromion; (2) the angle of acromion; and (3) the base of the scapular spine (
Fig. 1B). We estimated the static position of the scapula using (1) the angle of the acromion; (2) the base of the scapular spine; and (3) the inferior angle of the scapula (
Fig. 2). The segments of the humerus and trunk were made using points as recommended by the International Society of Biomechanics (ISB).
23) The segment of the trunk consisted of the seventh cervical vertebra, the eighth thoracic vertebra, the jugular notch, and the xiphoid process of the sternum. The humeral segment was made by the lateral epicondyle, the medial epicondyle, and the glenohumeral rotation center (
Fig. 1). The rotation center of the glenohumeral joint was calculated through regression analysis using the scapular bony landmarks.
24) With the subject's trunk and both upper extremities being exposed, we attached 0.9 cm sized reflective markers (Motion Analysis Co.) on the above mentioned points.
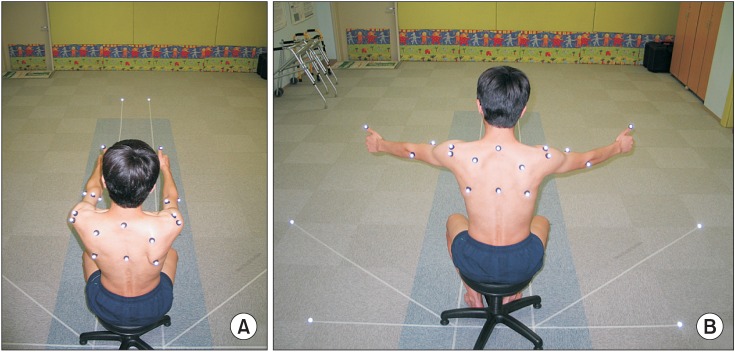 | Fig. 2To guide the motion on the intended plane, lines were drawn with attached reflective markers on the floor. (A) The photograph shows the subject performing sagittal plane flexion. (B) The photograph shows the subject performing scapular plane abduction.
|
Patients sat on a chair and elevated both upper extremities symmetrically to reduce compensatory movement of the trunk. We drew the guidelines on the laboratory floor along each plane of arm elevation. The patients elevated their arms while directing their thumbs according to guidelines to reduce the variation of the humeral rotation and perform proper elevation patterns (
Fig. 2). Prior to the evaluation, they were asked to practice several times to ensure proper arm movement pattern and timing. After several practices, elevation was performed five times for each patient.
The collected data represents the 3D scapular motions during arm elevation in 2 distinct elevation planes (sagittal plane flexion and scapular plane abduction). We defined sagittal plane flexion as an arm movement at 90° to the coronal plane (
Fig. 2A), and scapular plane abduction as an arm movement at 40° anterior to the coronal plane (
Fig. 2B). Using guidelines from the available literature, we instructed the patients to elevate both arms for approximately 4 seconds.
25) The collected data were analyzed using EVaRT 5.0 (Motion Analysis Co.) and SkB Software (Houston, TX, USA) that transformed the data obtained from cameras to an anatomically based local coordinate system.
In this study, the final data represented motions of the scapula and the humerus relative to the trunk. When the arm-trunk angles were recorded, the angles of 3D motions of the scapula were calculated simultaneously at each 10° increment during elevation of the arm in each of the planes of motion. After the segment of scapula was made, a local coordinate system of the scapula was created as recommended by the ISB.
23) The 3D scapular motion relative to the trunk was described using the YXZ Euler sequence.
23) The X-axis represented anterior/posterior tilt, Y-axis represented internal/external rotation, and Z-axis represented upward/downward rotation. The local coordinate systems developed for the scapula were oriented with the X-axis positive posteriorly, the Y-axis positive internally, and the Z-axis positive upwardly (
Fig. 3).
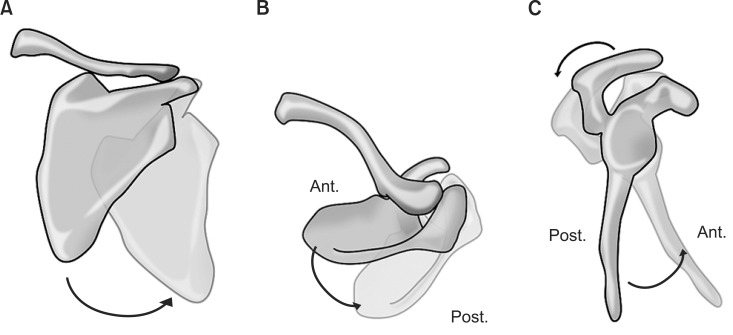 | Fig. 3The illustrations show the definition of right scapular motion with respect to the trunk. (A) Upward rotation. (B) Internal rotation. (C) Posterior tilting. Ant.: anterior, Post.: posterior.
|
Furthermore, 5 patients (all women; age, 69 to 75 years) were involved in a repeatability test using our system. We randomly measured the elevation of both upper extremities 5 times on the first day and repeated the same measurements 1 week later. We measured 3D scapular motions for 5 elevation evaluations of the patients, and calculated intra-class correlation coefficients (ICCs [1,5]) and the standard error of measurement for the 3 rotations of scapula mentioned above using the obtained values.
After the repeatability test, the angle of the humerus and the 3D scapular motion were recorded for each patient during arm elevation in each plane. The SHR was then calculated with the arm-trunk angle (H) and the angle of scapular upward rotation (S) using the following formula: SHR = (H – S) / S.
The Wilcoxon rank-sum test was performed to analyze differences between both shoulders. All statistical analyses were performed using SPSS ver. 18.0 (SPSS Inc., Chicago, IL, USA). A p-value of less than 0.05 was considered significant for differences between both shoulders.
Go to :

RESULTS
Overall, the system was found to be accurate within 0.03 mm at rest and 0.27 mm at motion for length, with angular orientations of 0.09° at rest and 0.43° at motion.
The ICC for trial-to-trial values was excellent on the first day, second day, and the total of both days: the ICC for upward rotation of the scapula ranged from 0.94 to 0.97; for internal rotation, from 0.96 to 0.99; and for posterior tilting, from 0.91 to 0.96. The standard error of measurement values in the 5 evaluations averaged 1.6° and 1.7° for sagittal plane flexion and scapular plane abduction, respectively.
The mean angular values for each of the humerus and scapula at rest are provided in
Table 1. We assumed 0° as the starting position for angle of the humerus and scapula. At the time of evaluation, the mean elevation of arm was 125.1° (range, 120.0° to 135.0°) for RTSA shoulders and 143.0° (range, 136° to 160°) for contralateral shoulders in sagittal plane flexion and scapular plane abduction. These patients reported a mean American Shoulder and Elbow Surgeons Standardized Shoulder Assessment Form (ASES) outcome score of 89.6 (range, 69.9 to 95) in the RTSA shoulders.
Table 1
Humeral and Scapular Positions between RTSA and Contralateral Shoulder at the Starting Position
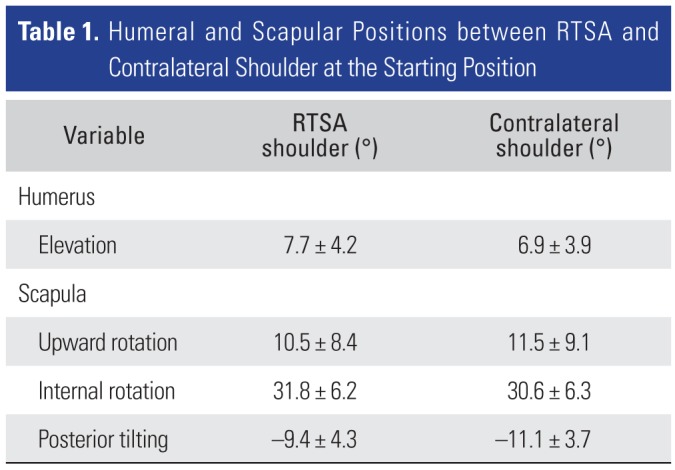
Variable |
RTSA shoulder (°) |
Contralateral shoulder (°) |
Humerus |
|
|
Elevation |
7.7 ± 4.2 |
6.9 ± 3.9 |
Scapula |
|
|
Upward rotation |
10.5 ± 8.4 |
11.5 ± 9.1 |
Internal rotation |
31.8 ± 6.2 |
30.6 ± 6.3 |
Posterior tilting |
−9.4 ± 4.3 |
−11.1 ± 3.7 |

In sagittal plane flexion, the mean upward rotational angle, internal rotational angle, and posterior tilting angle of the scapula were 53.1° ± 5.7°, 47.2° ± 4.5°, and 20.5° ± 4.8°, respectively, for the RTSA shoulders, and these angles for the contralateral shoulders were 47.2° ± 7.1°, 45.2° ± 7.0°, and 23.7° ± 4.7°, respectively. Statistically significant difference was found in the upward rotation (
p = 0.035) of the scapula between both arms (
Table 2 and
Fig. 4A). However, there was no significant difference in the internal rotation (
p = 0.614) and posterior tilting (
p = 0.321) of the scapula between both shoulders (
Table 2,
Fig. 4B and C).
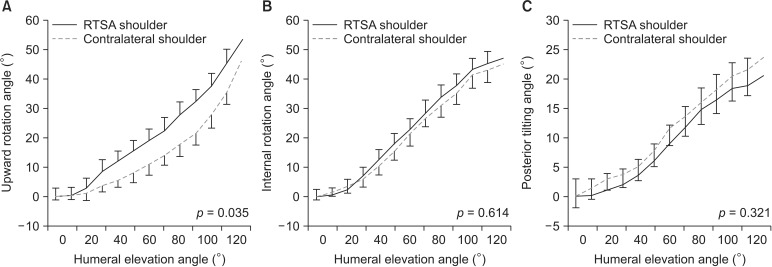 | Fig. 4Graphs showing the measured angle of the scapular motion of the reverse total shoulder arthroplasty and contralateral shoulders during sagittal plane flexion. (A) Upward rotation of the scapular motion was significantly different between both shoulders. However, there was no significant difference in internal rotation (B) and posterior tilting (C) of the scapular motion between both shoulders. RTSA: reverse total shoulder arthroplasty.
|
Table 2
Mean Anlge of Scapular Motion between RTSA and Contralateral Shoulder
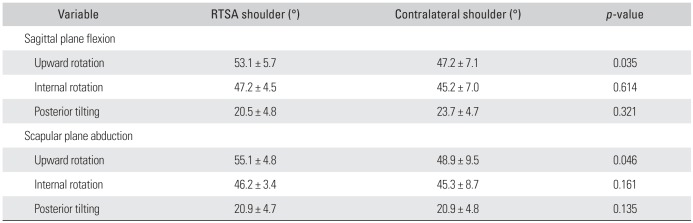
Variable |
RTSA shoulder (°) |
Contralateral shoulder (°) |
p-value |
Sagittal plane flexion |
|
|
|
Upward rotation |
53.1 ± 5.7 |
47.2 ± 7.1 |
0.035 |
Internal rotation |
47.2 ± 4.5 |
45.2 ± 7.0 |
0.614 |
Posterior tilting |
20.5 ± 4.8 |
23.7 ± 4.7 |
0.321 |
Scapular plane abduction |
|
|
|
Upward rotation |
55.1 ± 4.8 |
48.9 ± 9.5 |
0.046 |
Internal rotation |
46.2 ± 3.4 |
45.3 ± 8.7 |
0.161 |
Posterior tilting |
20.9 ± 4.7 |
20.9 ± 4.8 |
0.135 |

In scapular plane abduction, the mean upward rotational angle, internal rotational angle, and posterior tilting angle of scapula were 55.1° ± 4.8°, 46.2° ± 3.4°, and 20.9° ± 4.7°, respectively, for the RTSA shoulders, and these angles for the contralateral shoulders were 48.9° ± 9.5°, 45.3° ± 8.7°, and 20.9° ± 4.8°, respectively. Statistically significant difference was found in the upward rotation (
p = 0.046) of the scapula between both arms (
Table 2 and
Fig. 5A). However, there was no significant difference in the internal rotation (
p = 0.161) and posterior tilting (
p = 0.135) of the scapula between both shoulders (
Table 2,
Fig. 5B and C).
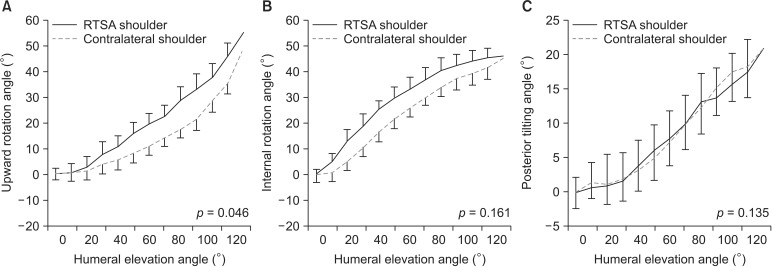 | Fig. 5Graphs showing the measured angle of the scapular motion of reverse total shoulder arthroplasty and contralateral shoulders during scapular plane abduction. (A) There was significant difference in the upward rotation of the scapular motion between both shoulders. However, internal rotation (B) and posterior tilting (C) of the scapular motion were not significantly different between both shoulders. RTSA: reverse total shoulder arthroplasty.
|
The mean SHRs during elevation are shown in
Table 3 and
Fig. 6. The SHR between both shoulders during arm elevation was significantly different in sagittal plane flexion (
p = 0.016) and scapular plane abduction (
p = 0.021) (
Table 3).
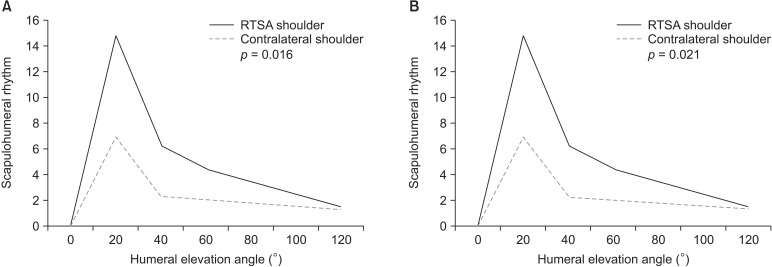 | Fig. 6Graphs showing the scapulohumeral rhythm (SHR) of the reverse total shoulder arthroplasty and contralateral shoulders during sagittal plane flexion (A) and scapular plane abduction (B). There was significant difference in the SHR during sagittal plane flexion and scapular plane abduction between both shoulders. RTSA: reverse total shoulder arthroplasty.
|
Table 3
The Average SHR between RTSA and Contralateral Shoulder during Arm Motion

Variable |
RTSA shoulder |
Contralateral shoulder |
p-value |
Sagittal plane flexion |
1.25:1 |
1.53:1 |
0.016 |
Scapular plane abduction |
1.17:1 |
1.45:1 |
0.021 |

Go to :

DISCUSSION
In previous studies, although the majority of motions from both healthy and RTSA shoulders were derived from the glenohumeral joint, the relative contribution of scapular motions to shoulder elevation was significantly increased in the RTSA shoulders.
2122) Such studies, however, reported only on the upward rotation of 3D scapular motions and SHR, whereas other motions including internal/external rotation and posterior/anterior tilting have not been addressed.
19202122) Therefore, we performed this study to analyze these additional scapular motions besides the upward rotation.
Few studies on RTSA shoulders with massive rotator cuff tears have reported on scapular kinematics including scapular upward rotation and SHR that may be insufficient to accurately describe the shoulder kinematics during elevation on account of more complicated motions of the scapula including upward/downward rotation, internal/external rotation, and anterior/posterior tilting.
19202122) On the other hand, in our study, we estimated 3D scapular motions consisting of upward/downward rotation, anterior/posterior tilting, and internal/external rotation in RTSA shoulders with rotator cuff tear arthropathy during arm elevation along sagittal plane flexion and scapular plane abduction. According to our data, during sagittal plane flexion, the scapular upward rotation was 53.1° and 47.2° in the RTSA shoulders and the contralateral asymptomatic shoulders, respectively (
p = 0.035). Similarly, during scapular plane abduction, the values for the RTSA shoulders and asymptomatic shoulders were 55.1° and 48.9°, respectively (
p = 0.046). The significant increase in the upward rotation of the scapula in the RTSA shoulders was consistent with that in previous studies.
212226) However, internal rotation and posterior tilting of the scapula were not significantly different between the RTSA and contralateral shoulders in this study. Although further research is needed to determine the cause of these differences, we identified that RSTA affected only upward rotation of the 3D scapular motion. We did not assess the scapular motion in the coronal plane abduction since a baseline comparison between the shoulders deemed impractical because dominant and nondominant shoulders would behave differently during this type of motion according to a previous study conducted using the same optical tracking system.
18)
The SHR has been a simple and reasonable representation of dynamic motions of the shoulder complex. It has been widely described as an important parameter of coordinated motion in healthy and diseased shoulders. The SHR in young normal shoulders has been reported to range from 1.35:1 to 7.9:1 whereas the SHR in RTSA shoulders has been reported to range from 1.3:1 to 2.4:1.
212226) In our study of 13 patients, the SHR of the RTSA shoulders was 1.25:1 and that of the contralateral shoulders was 1.53:1 during sagittal plane flexion (
p = 0.016). Similarly, the SHR was 1.17:1 for RTSA shoulders and 1.45:1 for the contralateral shoulders during scapular plane abduction (
p = 0.021). This indicates that the SHR is consistently lower in shoulders with RTSA than in healthy shoulders, which is also consistent with the findings of previous studies.
212226)
Increased scapular motion and reduced glenohumeral motion after RTSA may result from the reduced ability of the deltoid to provide support due to cuff deficiency and altered mechanics
22) although this should be confirmed by additional studies. In spite of the similar conclusions, the values of upward rotation and SHR in our study were quite different from those in previous studies. This may be attributable to the differences in instrumentation, planes of analysis, definition of axis orientation, determination of angular value around the starting position, measuring range, trunk position, types of subjects, and the use of static versus dynamic motion.
12)
There are some inherent limitations of this study. First, it is not certain whether such changes in the kinematics of the RTSA shoulders are due to the reversal of the anatomy after RTSA or adaptation that already existed before surgery as a response to rotator cuff deficiency. It is difficult to elucidate the cause of kinematic changes because it was difficult to perform preoperative kinematic examinations in most of these patients who were unable to elevate the arm before surgery. Second, the contralateral shoulders were evaluated based on history taking, physical examination, and simple X-ray without magnetic resonance imaging, ultrasonography, or arthroscopy. We attempted to minimize evaluation errors by performing exhaustive evaluations by an experienced shoulder surgeon who was otherwise not involved in this study. Third, the sample size was small. Nevertheless, in this study, we compared the RTSA shoulders to the contralateral asymptomatic shoulders of same subjects. This is a great strength of our study considering that previous studies compared the RTSA shoulders to those of other subjects or of younger subjects.
2122)
In conclusion, when we compared the 3D scapular motions and SHR between the RTSA and contralateral asymptomatic shoulders during arm motion, changes of shoulder kinematics in the RTSA shoulder were observed in the SHR values and the upward rotation of the scapular motion, not in the internal rotation and posterior tilt.
As increased scapular motions, especially the upward rotation, significantly affects shoulder motions after RTSA, a reduction of scapular motion should be carefully examined during preoperative evaluation. In addition, periscapular muscle pain, subscapular bursitis, acromioclavicular joint pain, and scapular spine stress fractures caused by altered shoulder kinematics have all been observed in shoulders with RTSA.
27) Thus, shoulder rehabilitation after RTSA should be focused on strengthening the periscapular muscle.
Go to :
