Abstract
Background
Regarding reconstruction surgery of the anterior cruciate ligament (ACL), there is still a debate whether to perform a single bundle (SB) or double bundle (DB) reconstruction. The purpose of this study was to analyze and compare the volume and surface area of femoral and tibial tunnels during transtibial SB versus transportal DB ACL reconstruction.
Methods
A consecutive series of 26 patients who underwent trantibial SB ACL reconstruction and 27 patients with transportal DB ACL reconstruction using hamstring autograft from January 2010 to October 2010 were included in this study. Three-dimensional computed tomography (3D-CT) was taken within one week after operation. The CT bone images were segmented with use of Mimics software v14.0. The obtained digital images were then imported in the commercial package Geomagic Studio v10.0 and SketchUp Pro v8.0 for processing. The femoral and tibial tunnel lengths, diameters, volumes and surface areas were evaluated. A comparison between the two groups was performed using the independent-samples t-test. A p-value less than the significance value of 5% (p < 0.05) was considered statistically significant.
Results
Regarding femur tunnels, a significant difference was not found between the tunnel volume for SB technique (1,496.51 ± 396.72 mm3) and the total tunnel volume for DB technique (1,593.81 ± 469.42 mm3; p = 0.366). However, the total surface area for femoral tunnels was larger in DB technique (919.65 ± 201.79 mm2) compared to SB technique (810.02 ± 117.98 mm2; p = 0.004). For tibia tunnels, there was a significant difference between tunnel volume for the SB technique (2,070.43 ± 565.07 mm3) and the total tunnel volume for the DB technique (2,681.93 ± 668.09 mm3; p ≤ 0.001). The tibial tunnel surface area for the SB technique (958.84 ± 147.50 mm2) was smaller than the total tunnel surface area for the DB technique (1,493.31 ± 220.79 mm2; p ≤ 0.001).
The anterior cruciate ligament (ACL) lesion is one of the most frequent events in sport injuries amongst young people.1) ACL reconstruction aims to restore knee stability,2) and the modern orthopedic practice offers several ACL reconstruction techniques. The single bundle (SB) ACL reconstruction ensures good outcomes and it is a well-established and widespread technique,3) although 15%-25% of suboptimal results (residual pain and instability) are recorded.4)
Recent anatomical studies5) have shown that the normal ACL structure mainly consists of 2-3 bundles: the anteromedial (AM), posterolateral (PL), and intermediate bundles. The first two bundles are considered the most important from a functional point of view. The SB ACL reconstruction technique, depending on the technique utilized and the insertion site chosen, creates a SB. The double bundle (DB) technique replaces both AM and PL bundles; thus it represents a more anatomically sound method, which should reflect better joint function. Although the DB ACL reconstruction technique has shown some biomechanical advantages over SB techniques,6) this has not yet been translated into any clinical advantages or reduction of revision rates.7) Nevertheless, DB ACL reconstruction has become increasingly popular over the past decade. However, reconstruction with a DB technique is known to add additional operating time to the reconstruction and also increase the cost of the procedure through the use of added fixation of the second graft to the tibia and femur and often the use of 1 or multiple expensive allograft tendons.8)
There are a few studies investigating the tunnel aperture and orientation in ACL reconstruction surgery based on radiographic measurements,9) despite the fact that they may be difficult to identify correctly on conventional radiographic images.10) Recent studies are based on three-dimensional computed tomography (3D-CT)10) or 3D magnetic resonance imaging.11) The purpose of this study was to assess the quantitative parameters (volume, surface area, etc.) of tunnels for transtibial SB versus transportal DB ACL reconstruction with hamstring autograft using a 3D-CT and computer software programs. We hypothesized that bone loss and the surface area, which may reflect the bone loss and the capacity for tendon-bone healing, would be decreased in each bone tunnels in transportal DB ACL reconstruction technique.
A consecutive series of 26 patients who underwent transtibial SB ACL reconstruction (group I) and 27 patients with transportal DB ACL reconstruction (group II) using hamstring autograft from January 2010 to October 2010 were included in this study. Patients who had less than a 6-month interval from injury to the operation were classified as 'group I.' This group consisted of 26 patients who underwent transtibial SB ACL reconstruction using the remnant preservation technique. Remnant preservation technique was used in more acute cases because more sufficient and healthier remnant tissue would be present. Patients who had over 6-month internal from the injury to operation were classified as 'group II.' This group consisted of 27 patients who underwent transportal DB ACL reconstruction. One senior surgeon performed all procedures. The Institutional Review Board approved the study protocol; all included patients provided written-informed consent.
In both techniques, the hamstring autograft tendon (semitendinosus and gracilis tendons) was harvested. Double loops (four-stranded) graft of hamstring tendon was made for group I (transtibial SB technique). A triple-stranded semitendinosus (for AM bundle) and triple-stranded gracilis (for PL bundle) were made for group II (transportal DB technique).
The mean diameter of double loop graft used for SB reconstruction technique was 8 ± 1 mm for femoral side and 8 ± 1 mm for the tibial tunnel. The mean diameters of triple-stranded semitendinosus (for AM bundle) and triple-stranded gracilis (for PL bundle) grafts used in DB reconstruction technique for femoral side were 7 ± 1 mm and 5 ± 1 mm, respectively. For tibial side, the graft diameters for AM and PL bundle were 7 ± 1 mm and 5 ± 1 mm, respectively. For the tunnel preparation, identical sized reamer was used. A tunnel dilator was not used.
In addition to the conventional anterolateral (AL) and AM portal, an accessary AM portal was made for later traction of the sutured remnant tissue. A suture hook (ConMed Linvatec, Largo, FL, USA) with a No. 0 polydioxanone synthetic (PDS; Ethicon, Sommerville, NJ, USA) was passed through the remnant ACL tissue. The remnant tissue on the tibial side was elevated using a curette and the remnant ACL stump was retracted to the medial side for protection during ACL reconstruction.12) A tibial tunnel was made using an ACL tibial guide (ConMed Linvatec) set at a 45° angle, with the tip of the tibial guide positioned at the central portion of the ACL remnant tissue. The extra-articular landmark of the tibia tunnel was 1 cm above the insertion of the pes anserinus and 1.5 cm medial to the tibial tubercle. Thereafter, the femoral tunnel was made using the transtibial technique between the 10 and 10:30 o'clock position for the right knee and between the 1:30 and 2 o'clock position for the left knee. A reamer, 1.0 mm smaller than the graft diameter, was introduced and the femoral tunnel was made to a 32-mm depth and at 1-2 mm before the posterior cortex.13)
For femoral fixation, two sleeves for the RigidFix cross pins (DePuy Mitek, Raynham, MA, USA) were inserted from the lateral side of the lateral femoral condyle. After confirming the precise location for inserting cross pins by arthroscopy, a Maxon 2-0 suture loop was inserted through the inferior sleeve of cross pin and retrieved to the far AM portal. The ends of the PDS sutures then were inserted into the Maxon suture loop, which was retrieved out of the inferior sleeve of cross pin. After graft passage, the graft was tensioned in full extension with 20 Lbs applied by a tensionometer (DePuy Mitek). Finally, inferior cross pin (2.7 mm) fixation was performed. Additional distal tibial post-tie fixation was performed.
Portal placement is important during anatomic DB ACL reconstruction. After making the routine AL and AM portals, the prepatella fat pad was debrided to allow clear visualization of the medial meniscus anterior horn. Needle localization is used to establish the accessory AM portal. When the needle is introduced, it should be directed towards the ACL femoral insertion site to ensure adequate access for later drilling of the tunnel.
After examining the ruptured ACL, the femoral footprints of both bundles were defined and marked with a thermal device (ArthroCare Co., Sunnyvale, CA, USA). To assist in identifying the anatomical outer margin of the footprint, osseous landmarks were used.14) The length and width measurements of each insertion sites were then taken using a metallic ruler.
Using the AM portal for viewing, the centers of the femoral insertion sites of the PL bundle and AM bundle were preliminarily marked with a Steadman awl which was inserted through the accessory AM portal. The guide pin was aimed for the marked point using the Bullseye femoral footprint guide (ConMed Linvatec). With the knee in deep flexion angle, the trajectory pin was aimed more anteriorly. The Sentinel Drill Bit (ConMed Linvatec) which matches the graft size of each bundle was used. The 4.5 mm cannulated EndoButton (Smith and Nephew Endoscopy, Andover, MA, USA) reamer was drilled to the lateral cortex. After measuring the inner aperture to the lateral cortex with the EndoButton depth gauge, the appropriately sized EndoButton was then chosen. A careful inspection of the bone bridge between the two tunnels was carried out to ensure that no tunnel communication occurred.
To create the tibia tunnels, the knee was flexed to 90°. An ACL tip-guide (ConMed Linvatec), set at 45°, was inserted via the accessory AM portal to create the PL tunnel. A 3.2-mm guide wire was inserted through the drill sleeve and advanced to the footprint of the PL bundle. For AM tunnel creation, the ACL tip-guide was reset at 50°.
First, the PL graft was passed, which was followed by the passage of the AM graft. For the femoral side fixation, an EndoButton CL (Smith and Nephew Endoscopy) was used. In cases where the distance between the aperture and the lateral femoral cortex (far cortex) was shorter than 30 mm, an EndoButton Direct (Smith and Nephew Endoscopy) was used to maximize the amount of graft in the tunnel. The grafts were pretensioned by 20 cycles of full knee flexion-extension motion. Final fixation of the grafts was done using interference screws at 0° of knee flexion both for the AM bundle and the PL bundle. Additional distal tibial post-tie fixation was performed.
Patients in both groups underwent the same postoperative rehabilitation protocol: all patients began active quadriceps isometric exercise and active range of motion exercise immediately after surgery. After operation, the affected knee was permitted gradual motion initially with a limited motion brace. Joint motion exercise was carried out at 15° increments per week. At 4 and 6 weeks after surgery, 90° and 135° of motion, respectively, were allowed. Patients were educated on performing proprioceptive balancing exercise at three months after surgery. At six and nine months after surgery, straight-line running and changing the direction while running, respectively, were allowed.
A CT scanner (LightSpeed VCT, GE Medical Systems, Milwaukee, WI, USA) was used for all examinations. It was taken within 1 week after operation for all patients. The collimation was 16 × 0.625 mm. The tube parameters were 120 kVp and 200 mA. The acquisition matrix was 512 × 512. The field of view was 140 mm and the slice thickness was 0.625 mm. The knee was placed in full extension. The bone was segmented and reconstructed to 3D point cloud model from the axial CT scan slices with use of Mimics software v14.0 (Materialise, Leuven, Belgium). The reconstructed 3D point cloud bone models were then imported in the commercial package Geomagic Studio v10.0 (Research Triangle Park, NC, USA) for processing into 3D surface models.
The femoral and tibial tunnels were separated by extracting the cortical portion of each bone using the Geomagic tools (Fig. 1). The length of each tunnel (mm) was measured from the center of aperture to the end of the tunnel. The best fit circular cylinder was generated by the program and its diameter was recorded as the tunnel diameter. The volume (mm3) was also generated by the program after sealing the apertures of the tunnel.
For surface area (mm2) measurements, the images were stored in 3DS format without the aperture sealing, which was then processed using the Google SketchUp Pro v8.0. In DB femoral tunnels, the tunnels were generated according to the implant used. The EndoButton Direct-used DB femoral tunnels (tunnel length < 30 mm) were processed without further extraction but for the EndoButton CL-used DB femoral tunnels, the loop portion (15 mm from the outer femoral cortex) was removed before processing.
Tunnel widening after ACL reconstruction was evaluated from radiographs of the anteroposterior and lateral views of the knee taken at the latest follow-up. The margins of the tibial tunnel were identified as a thin, clearly visible line on the immediate postoperative radiograph and as a line of cortical bone at final follow-up radiographs. On the anteroposterior and lateral radiographs, the diameter of the tibial tunnel was measured as described by Peyrache et al.15) All radiographic measurements were done on the picture archiving and communications system (PACS; GE Healthcare, Chicago, IL, USA) by use of a mouse cursor with automated distance calculation. Tunnel widening was defined as widening of greater than 2 mm at any time postoperatively on anteroposterior or lateral radiographs.16)
Preoperative demographic characteristic data were compared for the two groups using the t-test, Fisher exact test for gender.
The intraclass correlation coefficient (ICC) and standard error of measurement represented the intraobserver and interobserver reliability of each measurement. The single measured ICC was used to determine intraobserver reliability of measurements obtained on two occasions by each observer. The average measured ICC was used to evaluate interobserver reliability by comparing the means of two measurements of each variable. The ICC can assume any value from 0 to 1: where a value (x) > 0.80 represents good agreement, a value between 0.60 and 0.79 represents moderate agreement, and a value (x) < 0.59 represents poor agreement.
The mean, standard deviation, standard error, minimum, maximum, and 95% confidence interval values were determined for each measurement. Kolmogorov-Smirnoff test was used to assess the assumption of normality. An independent-samples t-test was used for comparison of total volume and surface area between the SB and DB reconstruction method.
All statistical analyses were performed with the SPSS ver. 12.0 (SPSS Inc., Chicago, IL, USA) and Analysis Excel 2007 (Microsoft Co., Redmond, WA, USA). A p-value of less than 0.05 was considered significant.
There was no significant difference between the SB and DB patient demographic characteristics (p > 0.05) (Table 1). All patients in the studied group were male with a mean age of 33.7 ± 1.7 for SB reconstruction patients and 31.8 ± 2.2 for DB ACL reconstruction patients. The ICC demonstrated high intraobserver and interobserver reliability (all values > 0.9). The measurements did not differ based on which surgeon was taking the measurements or whether it was the first or second measurements taken. All values showed normal distribution. There were no correlations with the 3D-CT measurements, including tunnel volume and contact surface area with the clinical outcome (all values > 0.05). Radiologic follow-up was possible (minimum 24 months) for 23/26 patients (88.5%) in SB group and 24/27 patients (88.9%) in DB group. None of the patients demonstrated tunnel widening (p > 0.05). A clinical difference between groups was not found (p > 0.05).17)
The length and diameter of femoral tunnel in transtibial SB technique was 33.83 ± 2.84 mm and 7.44 ± 0.78 mm, respectively (Table 2). The length and diameter of tibial tunnel in transtibial SB technique was 35.69 ± 3.85 mm and 8.45 ± 1.07 mm, respectively. The length and diameter of AM femoral tunnel in transportal DB technique was 30.70 ± 3.63 mm and 7.44 ± 0.83 mm, respectively (Table 3). The length and diameter of PL femoral tunnel was 30.64 ± 4.11 mm and 5.69 ± 0.85 mm, respectively. The length and diameter of AM tibia tunnel was 30.96 ± 4.24 mm and 7.94 ± 0.92 mm, respectively (Table 4). The length and diameter of PL tibia tunnel was 38.87 ± 2.92 mm and 5.95 ± 0.94 mm, respectively.
Regarding the femoral tunnel volume, there was no significant difference between the mean femoral tunnel volume for SB technique (1,496.51 ± 396.72 mm3) and the total femoral tunnel volume for DB technique (1,593.81 ± 469.42 mm3; p = 0.366) (Tables 2 and 3). The mean femoral tunnel volume for the AM bundle and PL bundle was 1,052.61 ± 327.98 mm3 and 541.20 ± 213.16 mm3, respectively. However, there was a significant difference of tibia tunnel volume (2,070.43 ± 565.07 mm3) for the SB technique and the total tibia tunnel volume (2,681.93 ± 668.09 mm3) for the DB technique (p ≤ 0.001) (Tables 2 and 4). The tibia tunnel volume was larger in the DB technique. The mean tibia tunnel volume for AM bundle and PL bundle used for DB reconstruction technique was 1,577.07 ± 371.19 mm3 and 1,104.86 ± 36.82 mm3, respectively.
Regarding the surface area, significant difference in total femoral and tibial tunnel surface area between the SB and DB technique was found. The relative total tunnel volumes and surface area was larger in the DB reconstruction technique. The femoral tunnel surface area for the SB technique was 810.02 ± 117.98 mm2, while the total femoral tunnel (AM + PL) surface area for the DB technique was 919.65 ± 201.79 mm2 (p ≤ 0.001) (Tables 2 and 3). The tibial tunnel surface area for the SB technique was 958.84 ± 147.50 mm2, while the total tibia tunnel (AM + PL) surface area for the DB technique was 1,493.31 ± 220.79 mm2 (p ≤ 0.001) (Tables 2 and 4).
The most significant finding of this study was: although the femoral tunnel volume was similar between the two techniques, the surface area was larger in the DB technique. For the tibia, both total tunnel volume and the surface area were larger in the DB technique. To the author's knowledge, this is the first study directly comparing the 3D tunnel volume and surface area between primary transtibial SB and transportal DB ACL reconstruction in vivo.
During the ACL reconstruction procedure, in addition to the movement toward more anatomic graft placement, DB using two tunnels in both femur and tibia had been suggested to better restore the AM and PL ACL bundle function.18) By creating two tunnels during the DB reconstruction procedure, additional bone damage into the metaphyseal trabecular cancellous bone of the distal femur and proximal tibia can be created.19) Important to be noted is that the peri-graft bone provides the surfaces for grafted tendon anchorage and the quality of tendon-to-bone healing is predictive of the outcome.20) Every effort had been taken for knee surgeons to recognize the importance of restoring osseous integrity during ACL reconstruction surgeries.21) The bony loss during SB reconstruction technique was 1,496.51 ± 396.72 mm3 for femoral tunnel and 2,070.43 ± 565.07 mm3 for tibia tunnel. However, the bony loss for DB reconstruction technique was larger: the total femoral bony loss (AM + PL tunnels) was 1,593.81 ± 469.42 mm3 and 2,681.93 ± 668.09 mm3 for tibia tunnels.
The optimum distance recommended for the amount of soft-tissue graft in a bone tunnel for healing had not been determined.22) In a recent goat study performed by Zantop et al.,23) they compared the structural and biomechanical properties of 15-mm and 25-mm split Achilles graft lengths within the bone tunnel. There was no difference in biomechanical stability between the two groups after 12 weeks of follow-up. Yet, most surgeons anecdotally prefer to have 20 mm or more of graft to give the best chance of strong tendon healing to bone within the tunnel because lack of graft incorporation is a common cause of surgical failure.24) In this study, all tunnels were more than 20 mm in length. For the SB technique, the length of femoral and tibia tunnel was 33.83 ± 2.84 mm and 35.69 ± 3.85 mm, respectively. For the DB technique, the length of femoral tunnels were 30.70 ± 3.63 mm for AM tunnel and 30.64 ± 4.11 mm for PL tunnel, respectively. For tibia tunnels, the length was 30.96 ± 4.24 mm for AM tunnel and 38.87 ± 2.92 mm for PL tunnel. In cases when the distance between the aperture and the lateral femoral cortex (far cortex) was shorter than 30 mm, an EndoButton Direct was used to maximize the amount of graft in the tunnel in this study. However, in order to promote appropriate healing, not only the length of the tunnel itself, but also the contact surface area between the tunnel and the graft should be considered. Contact surface area was generated directly from the software programs using Geomagic Studio v10.0 for processing into 3D surface models and the Sketchup Pro v8.0 calculating the surface area from the reconstructed 3D surface models (Fig. 1). As noted, contact surface area for both femur and tibia was larger during the DB reconstruction technique.
Because of the remarkable advantages of 3D measurement tools, they have been widely introduced in the medical field recently. Three-dimensional reconstructed CT scans of knee joint provide good visualization of the tunnel aperture and the surrounding bony morphology in both distal femur and proximal tibia models. Tunnel position evaluation using 3D-CT had been highly reliable,25,26) and 3D surface imaging and calculation programs had also been used in other medical fields.27) High reliability of these imaging studies and programs were also demonstrated in this study with high ICC for all measurements.
The present study has some limitations. First, only male patients were included in this study. Gender differences may be present and further study including both sexes would enhance the significance of the study. Second, the ratio between the tunnel diameter and graft size had not been analyzed. Increasing the tunnel diameter with the constant graft size, thus increasing the ratio between the tunnel diameter and the graft cross-sectional area, may provide more space for the graft to move at the tunnel. This graft movement in tunnel may decrease graft healing.28) Third, in order to reduce potential human errors measuring tunnel diameter, volume and surface area, the best fit diagram had been generated by the software program itself. But this method, again, has possible inaccuracies. To minimize the errors, all images were cropped and analyzed by two observers at different intervals. Fourth, clinical results are lacking. Lastly, the aim of each technique used in this study may be different. The SB reconstruction technique is based on slightly improved oblique femoral footprint position than the isometric method, while the DB reconstruction technique relies on anatomic foot print restoration.
Although the femoral tunnel volume was similar between two techniques, the surface area was larger in the DB technique. For the tibia, both total tunnel volume and the surface area were larger in the DB technique.
Figures and Tables
Fig. 1
Tunnel separation. (A) Transparent image obtained through three-dimensional digital reconstruction. (B) Removing parts other than the tunnel. (C) Sealing the tunnel for each volume measurement (black arrows). (D) Image processed for surface area measurement: (A-C) was processed in Geomagic Studio v10.0 and (D) was processed in SketchUp Pro v8.0.
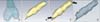
Table 1
Patient Demographic Characteristics of the Single Bundle and Double Bundle Reconstruction Groups
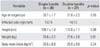
Table 2
Results of Quantitative Measurements of Femoral and Tibial Tunnels in Single Bundle ACL Reconstruction
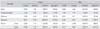
ACKNOWLEDGEMENTS
The authors thank Ms. Min-Jung Lee for her assistance with the statistical assessment of the data in this study and Ms. Ah-Reum Kim for patient data collection.
References
1. Gianotti SM, Marshall SW, Hume PA, Bunt L. Incidence of anterior cruciate ligament injury and other knee ligament injuries: a national population-based study. J Sci Med Sport. 2009; 12(6):622–627.
2. Galway HR, MacIntosh DL. The lateral pivot shift: a symptom and sign of anterior cruciate ligament insufficiency. Clin Orthop Relat Res. 1980; (147):45–50.
3. Li S, Su W, Zhao J, et al. A meta-analysis of hamstring autografts versus bone-patellar tendon-bone autografts for reconstruction of the anterior cruciate ligament. Knee. 2011; 18(5):287–293.
4. Freedman KB, D'Amato MJ, Nedeff DD, Kaz A, Bach BR Jr. Arthroscopic anterior cruciate ligament reconstruction: a metaanalysis comparing patellar tendon and hamstring tendon autografts. Am J Sports Med. 2003; 31(1):2–11.
5. Colombet P, Robinson J, Christel P, et al. Morphology of anterior cruciate ligament attachments for anatomic reconstruction: a cadaveric dissection and radiographic study. Arthroscopy. 2006; 22(9):984–992.
6. Yamamoto Y, Hsu WH, Woo SL, Van Scyoc AH, Takakura Y, Debski RE. Knee stability and graft function after anterior cruciate ligament reconstruction: a comparison of a lateral and an anatomical femoral tunnel placement. Am J Sports Med. 2004; 32(8):1825–1832.
7. Tsuda E, Ishibashi Y, Fukuda A, Tsukada H, Toh S. Comparable results between lateralized single- and double-bundle ACL reconstructions. Clin Orthop Relat Res. 2009; 467(4):1042–1055.
8. Brophy RH, Wright RW, Matava MJ. Cost analysis of converting from single-bundle to double-bundle anterior cruciate ligament reconstruction. Am J Sports Med. 2009; 37(4):683–687.
9. Musahl V, Burkart A, Debski RE, Van Scyoc A, Fu FH, Woo SL. Anterior cruciate ligament tunnel placement: comparison of insertion site anatomy with the guidelines of a computer-assisted surgical system. Arthroscopy. 2003; 19(2):154–160.
10. Basdekis G, Christel P, Anne F. Validation of the position of the femoral tunnels in anatomic double-bundle ACL reconstruction with 3-D CT scan. Knee Surg Sports Traumatol Arthrosc. 2009; 17(9):1089–1094.
11. Sadoghi P, Kropfl A, Jansson V, Muller PE, Pietschmann MF, Fischmeister MF. Impact of tibial and femoral tunnel position on clinical results after anterior cruciate ligament reconstruction. Arthroscopy. 2011; 27(3):355–364.
12. Ahn JH, Lee YS, Ha HC. Anterior cruciate ligament reconstruction with preservation of remnant bundle using hamstring autograft: technical note. Arch Orthop Trauma Surg. 2009; 129(8):1011–1015.
13. Ahn JH, Park JS, Lee YS, Cho YJ. Femoral bioabsorbable cross-pin fixation in anterior cruciate ligament reconstruction. Arthroscopy. 2007; 23(10):1093–1099.
14. Ferretti M, Ekdahl M, Shen W, Fu FH. Osseous landmarks of the femoral attachment of the anterior cruciate ligament: an anatomic study. Arthroscopy. 2007; 23(11):1218–1225.
15. Peyrache MD, Djian P, Christel P, Witvoet J. Tibial tunnel enlargement after anterior cruciate ligament reconstruction by autogenous bone-patellar tendon-bone graft. Knee Surg Sports Traumatol Arthrosc. 1996; 4(1):2–8.
16. Choi NH, Lee JH, Son KM, Victoroff BN. Tibial tunnel widening after anterior cruciate ligament reconstructions with hamstring tendons using Rigidfix femoral fixation and Intrafix tibial fixation. Knee Surg Sports Traumatol Arthrosc. 2010; 18(1):92–97.
17. Yang JH, Chang M, Kwak DS, Jang KM, Wang JH. In vivo three-dimensional imaging analysis of femoral and tibial tunnel locations in single and double bundle anterior cruciate ligament reconstructions. Clin Orthop Surg. 2014; 6(1):32–42.
18. Chhabra A, Starman JS, Ferretti M, Vidal AF, Zantop T, Fu FH. Anatomic, radiographic, biomechanical, and kinematic evaluation of the anterior cruciate ligament and its two functional bundles. J Bone Joint Surg Am. 2006; 88:Suppl 4. 2–10.
19. Nyland J, Fisher B, Brand E, Krupp R, Caborn DN. Osseous deficits after anterior cruciate ligament injury and reconstruction: a systematic literature review with suggestions to improve osseous homeostasis. Arthroscopy. 2010; 26(9):1248–1257.
20. Steiner ME, Murray MM, Rodeo SA. Strategies to improve anterior cruciate ligament healing and graft placement. Am J Sports Med. 2008; 36(1):176–189.
21. Dye SF, Chew MH. Restoration of osseous homeostasis after anterior cruciate ligament reconstruction. Am J Sports Med. 1993; 21(5):748–750.
22. Kanazawa T, Soejima T, Murakami H, Inoue T, Katouda M, Nagata K. An immunohistological study of the integration at the bone-tendon interface after reconstruction of the anterior cruciate ligament in rabbits. J Bone Joint Surg Br. 2006; 88(5):682–687.
23. Zantop T, Ferretti M, Bell KM, Brucker PU, Gilbertson L, Fu FH. Effect of tunnel-graft length on the biomechanics of anterior cruciate ligament-reconstructed knees: intra-articular study in a goat model. Am J Sports Med. 2008; 36(11):2158–2166.
24. Carson EW, Anisko EM, Restrepo C, Panariello RA, O'Brien SJ, Warren RF. Revision anterior cruciate ligament reconstruction: etiology of failures and clinical results. J Knee Surg. 2004; 17(3):127–132.
25. Miller CD, Gerdeman AC, Hart JM, et al. A comparison of 2 drilling techniques on the femoral tunnel for anterior cruciate ligament reconstruction. Arthroscopy. 2011; 27(3):372–379.
26. Lertwanich P, Martins CA, Asai S, Ingham SJ, Smolinski P, Fu FH. Anterior cruciate ligament tunnel position measurement reliability on 3-dimensional reconstructed computed tomography. Arthroscopy. 2011; 27(3):391–398.
27. Dong Y, Zhao Y, Bai S, Wu G, Wang B. Three-dimensional anthropometric analysis of the Chinese nose. J Plast Reconstr Aesthet Surg. 2010; 63(11):1832–1839.
28. Rodeo SA, Kawamura S, Kim HJ, Dynybil C, Ying L. Tendon healing in a bone tunnel differs at the tunnel entrance versus the tunnel exit: an effect of graft-tunnel motion? Am J Sports Med. 2006; 34(11):1790–1800.