Abstract
Background
The estimation of anterior cruciate ligament (ACL) tear is required in certain cases involving legal and financial administration, such as the worker's compensation and/or insurance. The aim of this study is to propose and evaluate a quantitative evaluation instrument to estimate the chronicity of the ACL tear, based on the four magnetic resonance imaging (MRI) findings.
Methods
One hundred and fifty one cases of complete ACL tear confirmed by arthroscopy were divided into 4 groups according to the time from ACL injury to MRI acquisition: acute (< 6 weeks), subacute (6 weeks to 3 months), intermediate (3 months to 1 year), and chronic (> 1 year). The four MRI findings including ACL morphology, joint effusion, posterior cruciate ligament angle, and bone bruise were analyzed for temporal changes among the 4 groups. Binary logistic regression equations were formulated using the MRI findings to estimate the chronicity of ACL tear in a quantitative manner, and the accuracy of the formulated regression equations was evaluated.
Results
The four MRI findings showed substantial temporal correlation with the time-limits of ACL injury to be included in the estimation model. Three predictive binary logistic equations estimated the probability of the ACL injury for the three cutoff time-limits of 6 weeks, 3 months, and 1 year with accuracies of 82.1%, 89.4%, and 89.4%, respectively.
Estimating the time of the anterior cruciate ligament (ACL) tear is required in certain cases involving legal and financial administration, such as the worker's compensation or insurance affairs. The availability of objective and quantitative tools to estimate the chronicity of the ACL tear is valuable for the related parties in such cases, as the injury history taken from the patient can be distorted by the related parties' interest in secondary gains. Magnetic resonance imaging (MRI) can provide objective information pertinent to the chronicity of ACL tear, and several MRI findings have been documented to display temporal changes after the ACL injury.1-6)
The MRI findings with temporal significance include ACL morphology, joint effusion, posterior cruciate ligament (PCL) angle, and bone bruise.3,6-8) The ACL morphology appears edematous and swollen in acute tears, and hypointense and fragmented or absent in chronic tears.3,6) Joint effusion is a non-specific marker for acute intra-articular lesion.9) PCL angle or buckling represents anterior translation of the tibial plateau relative to the femoral condyles due to ACL insufficiency, which increases with time after ACL injury.1,5,10) Bone bruise is detected on MR images in more than 80% of acute ACL rupture cases,11) and gradually diminishes from 3 weeks to several months post-injury.12-15) Our previous study found that the four MRI findings correlated well with the chronicity of ACL injury.6) However, previous studies have offered only qualitative estimation, and there has not been a quantitiative evaluation model for the chronicity of ACL injury.
The aim of this study was to propose the quantitative evaluation instruments to estimate the chronicity of ACL tear. We undertook to analyze a large number of MRIs with regard to the four MRI findings with temporal significance at various times after ACL injury, and to integrate the MRI findings into a regression model to estimate the chronicity of ACL injury. We hypothesized that the formulated regression models could estimate the chronicity of ACL tear quantitatively.
In this retrospective study, we reviewed 504 cases of arthroscopy-proven complete ACL tear, for which ACL reconstruction was performed by two surgeons (TKK and CBC) between February 2004 and December 2008. Inclusion criteria were the available precise ACL injury history with an obvious single major trauma event, confirmed by repeated history-taking in the emergency room, outpatient clinic, and first day of hospitalization. Exclusion criteria were the history of previous knee surgeries, knee deformity or history of fracture around the knee, any associated posterior cruciate ligament injury, or partial or total disruption of one or both collateral ligaments. According to the aforementioned criteria, 353 subjects were excluded from the study; and 151 knees of 151 subjects (130 male and 21 female) were included, with the mean age of 31.5 years (range, 10 to 56 years; standard deviation [SD], 10.3). Study subjects were allocated into 4 groups according to the time lapsed between the ACL injury and MRI acquisition: acute (within 6 weeks from injury); subacute (more than 6 weeks to 3 months from injury); intermediate (more than 3 months to 1 year from injury); and chronic (more than 1 year from injury).2,10,16) There were 71 acute, 19 subacute, 23 intermediate, and 38 chronic cases. Mean duration between ACL injury and MRI acquisition was as follows: 9.6 (SD, 11.4) days in acute; 63.2 (SD, 13.0) in subacute; 163.2 (SD, 67.8) in intermediate; and 2,220.5 (SD, 1,691.8) in chronic group. Demographic variables such as age, gender, and injury side were not significantly different among the four chronicity groups (p > 0.05). The study protocol was approved by the Institutional Review Board of the authors' institution.
All MR images were acquired digitally by using a picture archiving and communication system (PACS) in Digital Imaging and Communication in Medicine (DICOM) format. Image assessments were subsequently performed using PACS software (Impax: Agfa, Antwerp, Belgium) by a 1.5 Tesla scanner (Intera, Philips, Best, the Netherlands). The utilized protocol was as follows: T1-weighted (T1WI) sagittal (TR/TE, 450/20 ms); T2-weighted fat-suppressed sagittal (TR/TE, 2500/50 ms); proton density-weighted sagittal (TR/TE, 3300/12 ms); dual-echo proton density and T2-weighted coronal (TR/TE, 3300/12 or 55 ms); proton density-weighted coronal oblique (TR/TE, 3300/12 ms) and proton density-weighted fat suppressed axial images (TR/TE, 2700/20 ms); a 16 cm field of view; a 90° of flip angle; a 3 to 4 mm thickness; and a 512 × 512 matrix.
Four MRI findings with temporal significance were assessed according to the previous analysis: ACL morphology, joint effusion, PCL angle, and bone contusion.6) ACL morphologies were evaluated in the sagittal images that best visualized the ACL in the longest dimension and lowest signal intensity, and were evaluated using the three components of the signal intensity, shape, and nonvisualization.3,4,6,16) An abnormal signal intensity was defined as an increased signal intensity within the ACL, whether of a focal or diffuse pattern, or whether continuous or interrupted. The abnormal shape was defined as an edematous mass-like shape with a large wavy contour, or a band-like fragmented shape. Nonvisualization was defined as a failure to directly visualize ACL substance in any images on T1WI, proton-density, and T2WI images. Eventually, the ACL morphology was assorted into 3 grades: grade 1 was defined as early pattern with increased signal intensity and an edematous mass-like shape; grade 2 as an intermediate pattern with increased signal intensity and a band-like fragmented shape; and grade 3 as a late pattern with low signal intensity and a band-like fragmented shape, or nonvisualization (Fig. 1).3,4,6,17) Joint effusion was defined as the presence of fluid collection between the quadriceps tendon and fat pad in the posterior suprapatellar pouch, visualized as low signal intensity by T1WI and as high signal intensity by T2WI. The amount of joint effusion was measured by maximal dimension (mm) in the anteroposterior dimension of suprapatellar fluid collection on sagittal cuts of T2WI sagittal MRIs.2,18,19) PCL angle was defined as the angle between the lines drawn through the central portion of the tibial and femoral insertions of PCL on the sagittal MRIs that best showed the PCL.5,7) Bone bruise was defined as an increased signal intensity of bone marrow versus that of normal muscle substance, as described by the International Cartilage Repair Society classification.20) The anterior side of the lateral femoral condyle or the posterior side of the lateral tibial plateau was employed to measure the bone bruise on the fat suppressed T2WI sagittal image. The signal intensity of the bone bruise was measured using a modified circular region of interest (ROI).1,14,21,22) A circle of 1 cm2 area was set on an area of bone bruise using the circular ROI tool in the PACS software, and the maximum signal intensity of the interested region was measured. Signal intensity of the control bone marrow was measured in the same manner on the normal metaphyseal bone marrow (Fig. 2). Degree of bone contusion was equated to signal contrast, which was calculated from the ratio of the signal intensity of bone contusion to that of control bone marrow. All measurements were performed by a fellowship-trained musculoskeletal radiologist (JYC) who was blinded to the patient data.
Intra- and interobserver reliabilities of the MR assessments were investigated in randomly selected 15 knees. A musculoskeletal radiologist (JYC) and an orthopaedic specialist (JPY) performed measurements of the four MR findings twice with an interval of two weeks. The reliability was assessed using the intraclass correlation coefficient (ICC), which quantifies the proportion of the variance of the rating due to the between measurement variability. The ICC can assume any value from 0 and 1; a higher value means better agreement. In the analyses of the 15 knees, the ICC for intra- and interobserver reliabilities was greater than 0.8 for all 4 MR findings, similar to the previous studies.9,10,23,24) These results indicated that the MR assessments had satisfactory intra- and interobserver reliability.
Statistical analyses were conducted using SPSS ver. 12.0 (SPSS Inc., Chicago, IL, USA), and p-value < 0.05 was considered significant. In advance, the 4 MRI findings including ACL morphology, joint effusion, PCL angle, and bone bruise were compared among the 4 groups, divided by the interval between the injury and MRI acquisition. ACL morphology and the prevalence of bone bruise were compared by Mantel-Haenszel chi-square test. Joint effusion, PCL angle, and the signal contrast of the bone bruise were analyzed by correlation analyses. Finally, binary logistic regression analyses were performed to construct equations estimating the time of injury. The independent variables were the four MRI findings, namely, ACL morphology by grade 1-3, joint effusion (mm), PCL angle (degrees), and signal contrast of bone contusion. The dependent variable was the probability that injury had occurred after 6 weeks, 3 months, and 1 year. Based on the above assumptions, three logistic equations were formulated for the 3 cutoff time points, and classification accuracies were estimated for the formulated equations.
The four MRI findings showed substantial temporal changes to be included in the predictive equation model. High grade ACL morphology was more prevalent in older tears (p < 0.001). The amount of joint effusion was significantly correlated with the chronicity of injury (Spearman coefficient, -0.544; p < 0.001). The PCL angle (Spearman coefficient, -0.567; p < 0.001) and signal contrast of bone bruise (Spearman coefficient, -0.606; p < 0.001) also decreased with the chronicity of the injury. Because the changes of the 4 MRI findings showed substantial correlation with the times after ACL injury, they were included in the following estimation model.
Three logistic regression equations were formulated to calculate the probability that the ACL injury had occurred after three cutoff time-points (6 weeks, 3 months, and 1 year), and found to have statistically meaningful accuracies in estimating the chronicity of the ACL injury (Fig. 3). The three equations generated alpha values, which were then input to the common fractional exponential equation to yield the probability that the chronicity of ACL injury was greater than each time limit. If the probability that the ACL injury had occurred before each time-point was necessary, it could be calculated by subtracting the calculated probability from 1. The R2 coefficients of Nagelkerke for the three logistic regression models were 0.603, 0.745, and 0.678, suggesting that the predict models would explain the variation of the outcome to the extent of 60.3%, 74.5%, and 67.8%, respectively. The Hosmer-Lemeshow goodness-of-fit statistic for each of the three models revealed no significant departure from a good model fit (p = 0.678, 0.638, 0.944). The classification accuracies of the logistic regression models were 82.1%, 89.4%, and 89.4%, respectively.
The chronicity of ACL injury needs to be estimated based upon the imaging studies in certain cases, such as the medicolegal occasions or insurance claims. In these cases, the MRI findings with temporal significance may provide valuable data for the objective and quantitative estimation of the chronicity of ACL injury. We formulated three logistic probability equations from the significant temporal changes of 4 MRI findings with chronological significance, and it could be estimated the chronicity of ACL injury with statistically meaningful accuracies of greater than 80%. The temporal patterns of the 4 MRI findings with chronological significance are well known by the previous studies involving ACL morphology,4,24) joint effusion,9) PCL angle,1,10) and bone bruise,13-15) which was also documented synthetically in a previous study.6)
However, clinical application of the information is limited because of the lack of quantitative estimation model. Our finding of substantial correlation between the MRI findings and the chronicity of ACL injury was adopted to build a quantitative evaluation model to estimate the chronicity of ACL tear.
The formulated regression equations provide statistically meaningful accuracy for the quantitative estimation of the chronicity of ACL tear, which indicates that the formulated equations can be employed for practical use when objective and quantitative estimation of the chronicity of ACL tear is required. Also, we believed that our statistical model might explain the overall pathophysiological changes reflected on MR images in ACL deficient knees. The explanatory capability of the three equations was greater than 60%, and the classification accuracies by the logistic regression models for the cutoff time-points of 6 weeks, 3 months, and 1 year were 82.1%, 89.4%, and 89.4%, respectively. We expect that the equations can be useful in scenarios where the chronicity of ACL tear needs to be estimated from imaging modalities only, as required in some medicolegal and insurance cases. A case in example, a 33-year-old man sustained an ACL injury 3 days before the MRI was taken. ACL morphology was grade 1 (A1 = 0, A2 = 0), joint effusion (B) was 10.6 mm, PCL angle (C) was 138 degrees, and signal contrast of bone contusion (D) was 2.80 (Fig. 4). The alpha value was calculated to be -1.9054, and the probability that the ACL was injured more than 6 weeks prior was 12.9% by the first equation. The probability that the injury chronicity was less than 6 weeks was 87.1% (1 - 0.129). In the same manner, the probabilities of injury chronicity less than 3 months and less than 1 year were calculated to be 98.5% (1 - 0.015) and 99.7% (1 - 0.003) from the second and third equation, respectively. Accordingly, the probability of injury chronicity less than 6 weeks is relatively larger than the other cutoff time period, and the injury chronicity can be concluded to be less than 6 weeks. Taking for example another case, which had the MRI taken 4 months after ACL injury, ACL morphology was grade 2 (A1 = 1, A2 = 0), joint effusion (B) was 1.5 mm, PCL angle (C) was 104 degrees, and signal contrast of bone contusion (D) was 2.21. The probability of injury chronicity more than 6 weeks, more than 3 months, and more than 1 year were 86.0%, 61.1%, and 4.2%, respectively; and the probability of injury chronicity less than 6 weeks, less than 3 months, and less than 1 year were 14.0%, 38.9%, and 95.2%, respectively. The data can be interpreted that the ACL injury had occurred slightly more than 3 months before the MRI was taken. In addition, a probability of 50% gives that the injury occurred at around the time of cutoff.
Several limitations should be noted when interpreting our findings. First, the number of the subjects in the current study was not evenly distributed. The subacute (n = 19) and intermediate (n = 23) groups had relatively small number of subjects than acute group (n = 71), which might decrease the relevancy of the predictive model and statistical power. However, most ACL-injured patients visit emergency department or out-patient clinics and have the MRI taken at the early stages after the injury, and the predilection of subjects toward the acute group can be explained thus. Second, demographic or individual factors, such as age, gender, or injury mechanism were not considered. However, the pertinent MRI findings with chronological significance proven by the previous studies were included; and adoption of redundant variables into the equation model would not be relevant. Third, the knee position in taking MRI was not strictly standardized, and the variables might be measured differently if the knee position were changed, which was unavoidable in this retrospective study.
Finally, despite the objective and quantitative nature of the formulated equations, what the equations provide is only the probability that the chronicity of ACL tear belongs to a certain cutoff time interval. As such, the information derived from the equations cannot replace other clinical information such as physical findings and arthroscopic findings. The information from the formulated equations can be best interpreted in accordance with other relevant clinical information, if such is available.
This study demonstrates that the four MRI findings with chronological significance, namely, ACL morphology, joint effusion, PCL angle, and bone bruise can be successfully adopted to formulate logistic regression equations to estimate the chronicity of ACL tear. We propose that the three equations be an objective and quantitative tool when the chronicity of ACL tear needs to be estimated based only upon MRI findings.
Figures and Tables
Fig. 1
Classification of anterior cruciate ligament (ACL) morphology on T2-weighted fat-suppressed magnetic resonance images. (A) Grade 1: early pattern of increased signal intensity and an edematous mass-like shape. (B) Grade 2: intermediate pattern of increased signal intensity with a fragmented shape. (C) Grade 3: low signal intensity and band-like fragmented shape. (D) Nonvisualization of the ACL.
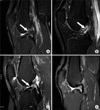
Fig. 2
Bone bruise measured by signal contrast, which was defined as the ratio of mean signal intensity of a bone contusion (black circle) versus that of normal metaphyseal area (white circle).
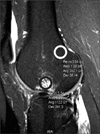
Fig. 3
Equations by logistic regression models to estimate the chronicity of anterior cruciate ligament (ACL) tear. The ACL morphology (A1, A2), joint effusion (B), posterior cruciate ligament (PCL) angle (C), and bone contusion (D) were measured on magnetic resonance imaging, and the measured values were inserted into the 3 equations. Each equation represents the probability that the ACL tear occurred after the corresponding cutoff time of 6 weeks, 3 months, and 1 year, respectively. Thus, the probability that the injury chronicity was less than the cut-off time can be calculated by subtracting the probability that the ACL injury occurred at a point higher than the cut-off time from 1.
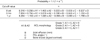
Fig. 4
A 33-year-old man underwent anterior cruciate ligament (ACL) injury 3 days before the magnetic resonance imaging (MRI) was taken. ACL morphology was grade 1 (A1 = 0, A2 = 0), joint effusion (B) was 10.6 mm, posterior cruciate ligament angle (C) was 138 degrees, and the bone contusion signal contrast (D) was 2.80. The logistic equations yield that the probability that the ACL injury occurred more than 6 weeks, 3 months, and 1 year is 12.9%, 1.5%, and 0.3%, respectively; and the probability that it occurred at less than each cutoff time is 87.1%, 98.5%, and 99.7%, respectively. This can be interpreted that the ACL tear must have occurred less than 6 weeks.
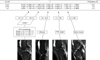
References
1. Dimond PM, Fadale PD, Hulstyn MJ, Tung GA, Greisberg J. A comparison of MRI findings in patients with acute and chronic ACL tears. Am J Knee Surg. 1998. 11(3):153–159.
2. Johnson MW. Acute knee effusions: a systematic approach to diagnosis. Am Fam Physician. 2000. 61(8):2391–2400.
3. Gentili A, Seeger LL, Yao L, Do HM. Anterior cruciate ligament tear: indirect signs at MR imaging. Radiology. 1994. 193(3):835–840.


4. Vahey TN, Broome DR, Kayes KJ, Shelbourne KD. Acute and chronic tears of the anterior cruciate ligament: differential features at MR imaging. Radiology. 1991. 181(1):251–253.


5. Vahey TN, Hunt JE, Shelbourne KD. Anterior translocation of the tibia at MR imaging: a secondary sign of anterior cruciate ligament tear. Radiology. 1993. 187(3):817–819.


6. Yoon JP, Chang CB, Yoo JH, et al. Correlation of magnetic resonance imaging findings with the chronicity of an anterior cruciate ligament tear. J Bone Joint Surg Am. 2010. 92(2):353–360.


7. McCauley TR, Moses M, Kier R, Lynch JK, Barton JW, Jokl P. MR diagnosis of tears of anterior cruciate ligament of the knee: importance of ancillary findings. AJR Am J Roentgenol. 1994. 162(1):115–119.


8. Vellet AD, Marks PH, Fowler PJ, Munro TG. Occult posttraumatic osteochondral lesions of the knee: prevalence, classification, and short-term sequelae evaluated with MR imaging. Radiology. 1991. 178(1):271–276.


9. Boks SS, Vroegindeweij D, Koes BW, Hunink MM, Bierma-Zeinstra SM. Magnetic resonance imaging abnormalities in symptomatic and contralateral knees: prevalence and associations with traumatic history in general practice. Am J Sports Med. 2006. 34(12):1984–1991.


10. Lee K, Siegel MJ, Lau DM, Hildebolt CF, Matava MJ. Anterior cruciate ligament tears: MR imaging-based diagnosis in a pediatric population. Radiology. 1999. 213(3):697–704.


11. Rosen MA, Jackson DW, Berger PE. Occult osseous lesions documented by magnetic resonance imaging associated with anterior cruciate ligament ruptures. Arthroscopy. 1991. 7(1):45–51.


12. Bretlau T, Tuxoe J, Larsen L, Jorgensen U, Thomsen HS, Lausten GS. Bone bruise in the acutely injured knee. Knee Surg Sports Traumatol Arthrosc. 2002. 10(2):96–101.


13. Costa-Paz M, Muscolo DL, Ayerza M, Makino A, Aponte-Tinao L. Magnetic resonance imaging follow-up study of bone bruises associated with anterior cruciate ligament ruptures. Arthroscopy. 2001. 17(5):445–449.


14. Davies NH, Niall D, King LJ, Lavelle J, Healy JC. Magnetic resonance imaging of bone bruising in the acutely injured knee: short-term outcome. Clin Radiol. 2004. 59(5):439–445.


15. Roemer FW, Bohndorf K. Long-term osseous sequelae after acute trauma of the knee joint evaluated by MRI. Skeletal Radiol. 2002. 31(11):615–623.


16. Mink JH, Deutsch AL. Magnetic resonance imaging of the knee. Clin Orthop Relat Res. 1989. (244):29–47.


17. Chen WT, Shih TT, Tu HY, Chen RC, Shau WY. Partial and complete tear of the anterior cruciate ligament. Acta Radiol. 2002. 43(5):511–516.


18. Kolman BH, Daffner RH, Sciulli RL, Soehnlen MW. Correlation of joint fluid and internal derangement on knee MRI. Skeletal Radiol. 2004. 33(2):91–95.


19. Schweitzer ME, Falk A, Berthoty D, Mitchell M, Resnick D. Knee effusion: normal distribution of fluid. AJR Am J Roentgenol. 1992. 159(2):361–363.


20. Brittberg M, Winalski CS. Evaluation of cartilage injuries and repair. J Bone Joint Surg Am. 2003. 85:Suppl 2. 58–69.


21. Schmid MR, Hodler J, Vienne P, Binkert CA, Zanetti M. Bone marrow abnormalities of foot and ankle: STIR versus T1-weighted contrast-enhanced fat-suppressed spin-echo MR imaging. Radiology. 2002. 224(2):463–469.


22. Vogl TJ, Schmitt J, Lubrich J, et al. Reconstructed anterior cruciate ligaments using patellar tendon ligament grafts: diagnostic value of contrast-enhanced MRI in a 2-year follow-up regimen. Eur Radiol. 2001. 11(8):1450–1456.


23. Robertson PL, Schweitzer ME, Bartolozzi AR, Ugoni A. Anterior cruciate ligament tears: evaluation of multiple signs with MR imaging. Radiology. 1994. 193(3):829–834.


24. Mellado JM, Calmet J, Olona M, Gine J, Sauri A. Magnetic resonance imaging of anterior cruciate ligament tears: reevaluation of quantitative parameters and imaging findings including a simplified method for measuring the anterior cruciate ligament angle. Knee Surg Sports Traumatol Arthrosc. 2004. 12(3):217–224.

