Abstract
Background
To determine if exogenously injected bone marrow derived platelet-rich plasma (PRP) plus bone morphogenetic protein (BMP)-2 could accelerate the healing of bone-tendon junction injuries and increase the junction holding strength during the early regeneration period.
Methods
A direct injury model of the bone-tendon junction was made using an Achilles tendon-calcaneus bone junction in a rabbit. In the PRP/BMP-2/fibrin group, 0.05 mL of bone marrow derived PRP and 100 ng/mL of BMP-2 both incorporated into 0.1 mL of fibrin glue were injected into Achilles tendon-calcaneus bone junctions. The effect of the intervention was tested by comparing the results of an intervention group to a control group. The results of biomechanical testing, and histological and gross analyses were compared between the 2 groups at the following time points after surgery: 2 weeks, 4 weeks, and 8 weeks.
Results
Histologic examinations showed that woven bone developed in tendon-bone junctions at 2 weeks after surgery in the PRP/BMP-2/fibrin group. Mechanical test results showed no significant difference between the PRP/BMP-2/fibrin and control groups at 2 and 4 weeks after surgery, but the mean maximal load in the PRP/BMP-2/fibrin group was significantly higher than in the control group (p < 0.05) at 8 weeks after surgery.
Tendon-bone junctions are composed of a complex mix of hard bony and soft tendon tissues. The restoration of collagen fibers and fibrocartilage in the transitional zone after injury is crucial in terms of regaining the mechanical properties of the tendon-bone junction. However, this transitional region has poor healing potential due to its hypovascularity.1,2)
Previous studies have examined bone-tendon junction healing within bone tunnels, and various methods have been devised to improve early fixation and to facilitate healing. These methods have included decortication and the creation of a bone trough, the use of a dowel plug, and stapling.3-5) In the past, shock waves, allogenic chondrocytes, platelet-derived growth factor, and bone marrow matrix cells with bone morphogenetic protein have been reported to facilitate bone-tendon junction healing.6-10) However, few studies have been reported an accelerated healing of bone-tendon junction injuries.6,11) Platelet-rich plasma (PRP) has attracted much attention, because it is a safe and cost effective source of growth factors that stimulate cellular regeneration, and as a result, many reports have been issued on the osteogenic effect of PRP.12-16) In addition, PRP has been reported to accelerate tenocyte proliferation and collagen production by injured tenocytes.17) PRP from bone marrow aspirate contains platelets, growth factors, and condensed nucleated bone marrow cells, all of which contribute to tissue regeneration.18)
Repair processes after tissue injury are regulated by different cell types, growth factors, and proteins. Of these growth factors, bone morphogenetic protein (BMP) regulates cell proliferation, differentiation, apoptosis, morphogenesis, and organogenesis.19-21) Furthermore, experimental studies have revealed that BMP has a beneficial effect on tendon healing.19)
We hypothesized that PRPs could accelerate tendon healing and BMPs bone healing at the tendon-bone junction. The present study was designed to evaluate and to compare the microscopic features and mechanical properties of tissue healing in surgically created tendon Achillescalcaneus junction injuries in a rabbit model in response to exogenous BMP-2 plus bone marrow PRP in a fibringlue carrier.
Three-month-old skeletally mature male New Zealand white rabbits (n = 54, 3.5 ± 0.5 kg; Jung-Ang Animal Laboratory, Seoul, Korea) were housed in cages and fed commercially available rabbit food. Right hind legs were used for the experiment and left hind legs were preserved. NIH guidelines for the care and use of laboratory animals published by the National Institute of Health (NIH publication no. 85-23, revised 1985) were observed throughout the study.
The animals were anesthetized with an intramuscular injection of 250 mg ketamine hydrochloride (Yuhan, Seoul, Korea). Standard sterile drape was applied to the pelvic area, and 2 mL of bone marrow was aspirated in the standard manner from iliac crests. Autogenous PRP was obtained by centrifuging bone marrow aspirates at 4,000 rpm for 10 minutes.
Under standard aseptic conditions, an L-shaped skin incision was made over the posterior aspect of the each right heel and the insertion site of the Achilles tendon was exposed. The tendon was transected at its insertion and a bone tunnel was made on both sides of the calcaneus using an arrow pointing device (towel clip). The tendon was reattached later at the calcaneal tuberosity using a pullout steel wire 2-0 suture (Ethicon, Johnson & Johnson International, Warsaw, IN, USA). The plantaris tendon also transected (Fig. 1).
The animals were divided into 3 groups: in the control group (n = 18), after reattaching the tendon, no further procedure was done; in the other second group (each n = 18), a mixture of bone marrow derived PRP and fibrin glue (0.1 mL; the PRP/fibrin group); and in a 3rd group, bone marrow derived PRP, BMP-2 (Sigma-Aldrich, St. Louis, MO, USA), and fibrin glue (0.1 mL of fibrin glue mixed with 100 ng/mL of BMP-2 and 0.05 mL of bone marrow derived PRP: the PRP/BMP-2/fibrin group) was injected into the tendon-bone repaired gap. Wounds were closed in a layered fashion and limbs were immobilized using a short leg cast in the position of ankle-plantar flexion. Rabbits were allowed to move freely within their cages. Six rabbits per group were sacrificed at 2, 4, and 8 weeks after surgery for evaluation.
Animals were anesthetized with an intramuscular injection of 250 mg ketamine hydrochloride and euthanized with i.v., potassium chloride. Under standard aseptic conditions, previous surgical wounds were opened and the tendon Achilles-calcaneus complex was harvested. Harvested specimens were examined grossly and findings were recorded. Specimens were allocated randomly for histological and mechanical studies.
The specimens for histological analysis were fixed in 10% buffered formalin, decalcified with decalcifying agent (Decal Rapid, National Diagnostics, Atlanta, GA, USA), dehydrated using a graded alcohol series and embedded in paraffin. Tissue sections (6 µm) were cut perpendicular to the tendon-to-bone junction and stained with H&E and Masson's trichrome. Specimens for biomechanical studies were frozen separately at -70℃ in 50 mL bottles until required for testing.
The following parameters were assessed using a light microscope (Zeiss, Carl Zeiss, Oberkochen, Germany): cell types, nature of the matrix present in the tendon-bone interface, collagen fiber alignment, amount of fibrocartilage, and new bone formation.
Frozen specimens were thawed in normal saline at room temperature before testing, and the cross-sectional area of healing interfaces were measured. In each case, the tendon Achilles-calcaneus complex was securely fixed in a specially designed apparatus, which allowed the specimen to be oriented such that a tensile load could be applied along the axis of the tendon. The whole construct was loaded onto an Instron mechanical tester (Instron 4201, Instron, Canton, MA, USA) operated at 10 mm/minute velocity traction speed. Maximum strain, maximum load, ultimate stress, and elasticity modulus were recorded on a personal computer.
For all the specimens from each treatment group, the Achilles tendon was loosely connected to calcaneus bone and adhered to surrounding soft tissues. Few differences were observed between the groups at any time point (2, 4, or 8 weeks after surgery) (Fig. 2).
At 2 weeks after surgery, cartilage formation was observed at tendon-bone junctions in the control group. Fibrocartilage formation was observed at tendon-bone junctions in the PRP/fibrin and the PRP/BMP-2/fibrin groups, and woven bone was visible in the PRP/BMP-2/fibrin group (Fig. 3).
At 4 weeks, fibrocartilage was observed in the control group and woven bone in the PRP/fibrin group. Fibrous tissues in junctions were well aligned with bone in the PRP/BMP-2/fibrin group. At 8 weeks, fibrous tissues were loosely connected to bone in the control group, but fibrous tissues were connected to bone through newly formed cartilages in the PRP/fibrin group and to bone through new bones in the PRP/BMP-2/fibrin group (Fig. 4).
Mean maximum loads at failure of tendon bone junction complexes were 47.9 ± 24.9, 74.7 ± 18.6, 60.5 ± 15.0 N respectively in the control, the PRP/fibrin group, and the PRP/BMP-2/fibrin group for specimens obtained at 2 weeks after surgery. Mean maximal load at failure in the PRP/BMP-2/fibrin group was second highest, but this was not significant (p > 0.05). Corresponding mean maximum loads at failure at 4 weeks after surgery were 46.1 ± 4.3, 61.3 ± 28.5, 73.9 ± 19.5 N, again the mean maximal load at failure was not significantly greater for the PRP/BMP-2/fibrin group. Similarly, mean maximal loads at failure at 8 weeks were 62.4 ± 4.9, 89.8 ± 4.6, and 99.6 ± 10.3 N, respectively, but on this occasion the mean maximum load at failure was significantly greater in the PRP/BMP-2/fibrin group (p = 0.007) (Table 1).
The Achilles tendon-calcaneus bone complex in our study serves as a good model for bone-tendon junction injury. The healing of tendon-bone junction is restoring alignment of collagen fibers and fibrocartilages with appropriate mechanical strength at the transition zone after an injury. Restoration of collagens and fibrocartilages between tendon and bone is essential for regaining the mechanical properties of the bone-tendon junction.22) About 8 week is healing time of rabbit's tendon-bone injury, but the mechanical strength is up to 80% of normal tendon bone junction.23) In this study, improvement of biomechanical properties of scar tissue with time in all groups suggested that healing progressed with time. Fibrocartilage is one of the components of repaired tissue at tendon-bone junction injury. Thick fibrocartilage may reinforce the link of between tendon and bone.24) The direct insertion complex of tendon bone junction is transitionally composed of a tendon, onocalcified fibrocartilage, calcified cartilage, and bone. This four-zone arrangement yields a gradient of mechanical stiffness that avoids traumatic injury due to concentration of stress at interface.25) Histologically fibrocartilage formation was observed earlier in PRP/fibrin group and PRP/BMP-2/fibrin in our study. Tendon was connected to the calcaneus bone through the fibrocartilage and new bone in 8 weeks PRP/BMP-2/fibrin group specimens. We thought that bone marrow derived autogenous PRP and rhBMP-2 gave strong integration between Achilles tendon and calcaneus bone. In this study, we found that a mixture of bone marrow derived autogenous PRP, rhBMP-2, and fibrin glue connected tendons to bone through newly developed bone and promoted mechanical stability following repair of a ruptured tendon-bone junction. Mechanical stability increased significantly with time after injecting bone marrow derived autogenous PRP, rhBMP-2, and fibrin glue into ruptured sites.
PRP has been used as source of platelet derived growth factor and transforming growth factor,26) and it has been reported that PRP has a positive effect on cell proliferation and collagen production, and that it induces the production of matrix-degrading enzymes and endogenous growth factors by human tenocytes.17) Furthermore, some reports have shown that PRP has osteogenic effects.12,14-16) In particular, PRP derived from bone marrow contains growth factors and mesenchymal stem cells, and these cells have been reported to promote tendon injury healing.27)
BMP are bone forming factors and can accelerate bone formation in tendons.21) Cartilage-derived BMP has been reported to promote the formation of large, strong tendon calluses.26) Rodeo et al.,28) in a dog model, demonstrated that recombinant human BMP-2 accelerated tendon to bone healing in a bone tunnel and that it induced a transitory increase in pull-out strength. In a previous study, we found that BMP-2 accelerated the formation of fibrocartilage during the early phase of healing and increased the biomechanical strength of bone-tendon junctions.6) However, no report has been issued on the effect of bone marrow derived PRP mixed with BMP-2. In the present study, a mixture of bone marrow derived PRP and BMP-2 was found to promote the production of bony tissue tendon/bone junctions and to increase biomechanical strength.
Fibrin glue can be used as a carrier of exogenous cytokines and metabolized without foreign bodies,29) and thus, we used fibrin glue as a carrier in the present study.
In the field of orthopaedic surgery, there are many kinds of tendon bone junction injury such as rotator cuff tear, collateral ligament injury of knee, patellar tendon injury, Achilles tendon injury and extensor tendon injury of finger. Furthermore, the tendon reconstruction and tendon transfer is issue of the tendon bone integration. Autogenous PRP mixed with BMP-2 could give early stability to tendon-bone junction such as, rotator cuff repair, anterior cruciate ligament graft and tendon transfer around foot and ankle area in clinical situation from our study results.
In conclusion, bone marrow derived PRP plus BMP-2 was found to improve significantly the mechanical strength of tendon bone junctions and to accelerate the formation of fibrocartilage and new bone after tendon to bone junction injury.
Figures and Tables
Fig. 1
Operative procedure. (A) Arrow point device (towel clip) made a hole at calcaneus. (B) The tendon was sutured with 2-0 steel wire in the rectangular manner and the fibrin glue mixed with bone marrow derived platelet-rich plasma and bone morphogenetic protein-2 was injected into the tendon to bone junction.
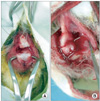
Fig. 2
Gross findings at 2 weeks. No differences were found between the 3 groups. (A) The control group. (B) The platelet-rich plasma/fibrin group. (C) The platelet-rich plasma/bone morphogenetic protein 2/fibrin group.
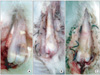
Fig. 3
Histological photographs at 2 weeks (H&E, × 50). (A) Cartilage was visible at the tendon-bone junctions in the control group. (B) Fibrocartilage was visible in the platelet-rich plasma/fibrin group. (C) Fibrocartilage and woven bone were visible in platelet-rich plasma/bone morphogenetic protein 2/fibrin group. C: calcaneus bone, J: junction, T: tendon.
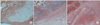
Fig. 4
Histological photographs taken at 8 weeks (H&E, × 50). (A) Fibrocartilage was visible at tendon-bone junctions in the control group. (B) Tendons were connected to bone via fibrocartilage in the platelet-rich plasma/fibrin group. (C) Tendons were connected to bone via new bone in the platelet-rich plasma/bone morphogenetic protein 2/fibrin group. C: calcaneus bone, J: junction, T: tendon.
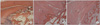
ACKNOWLEDGEMENTS
This research was supported by Basic Science Research Program through the National Research Foundation of Korea (NRF) funded by the Ministry of Education, Science and Technology (KRF-2008-313-E00356).
References
1. Thomopoulos S, Williams GR, Gimbel JA, Favata M, Soslowsky LJ. Variation of biomechanical, structural, and compositional properties along the tendon to bone insertion site. J Orthop Res. 2003. 21(3):413–419.


2. Benjamin M, Evans EJ, Copp L. The histology of tendon attachments to bone in man. J Anat. 1986. 149:89–100.
3. St Pierre P, Olson EJ, Elliott JJ, O'Hair KC, McKinney LA, Ryan J. Tendon-healing to cortical bone compared with healing to a cancellous trough: a biomechanical and histological evaluation in goats. J Bone Joint Surg Am. 1995. 77(12):1858–1866.


4. Ward JJ, Meyer RD, Lemons JE. Tensile strength comparison of dowel plug technique to standard techniques of tendonbone attachment. Foot Ankle. 1988. 8(5):248–253.


5. Wong MW, Qin L, Lee KM, et al. Healing of bone-tendon junction in a bone trough: a goat partial patellectomy model. Clin Orthop Relat Res. 2003. (413):291–302.
6. Kim HJ. The Healing effect of bone morphogenetic protein with fibrin glue on an injury of the tendon-bone junction [dissertation]. 2006. Seoul: Korea University.
7. Kobayashi K, Healey RM, Sah RL, et al. Novel method for the quantitative assessment of cell migration: a study on the motility of rabbit anterior cruciate (ACL) and medial collateral ligament (MCL) cells. Tissue Eng. 2000. 6(1):29–38.


8. Ouyang HW, Goh JC, Thambyah A, Teoh SH, Lee EH. Knitted poly-lactide-co-glycolide scaff old loaded with bone marrow stromal cells in repair and regeneration of rabbit Achilles tendon. Tissue Eng. 2003. 9(3):431–439.


9. Wang CJ, Huang HY, Pai CH. Shock wave-enhanced neovascularization at the tendon-bone junction: an experiment in dogs. J Foot Ankle Surg. 2002. 41(1):16–22.


10. Wong MW, Qin L, Tai JK, Lee SK, Leung KS, Chan KM. Engineered allogeneic chondrocyte pellet for reconstruction of fibrocartilage zone at bone-tendon junction: a preliminary histological observation. J Biomed Mater Res B Appl Biomater. 2004. 70(2):362–367.
11. Hashimoto Y, Yoshida G, Toyoda H, Takaoka K. Generation of tendon-to-bone interface "enthesis" with use of recombinant BMP-2 in a rabbit model. J Orthop Res. 2007. 25(11):1415–1424.


12. Arpornmaeklong P, Kochel M, Depprich R, Kubler NR, Wurzler KK. Influence of platelet-rich plasma (PRP) on osteogenic differentiation of rat bone marrow stromal cells: an in vitro study. Int J Oral Maxillofac Surg. 2004. 33(1):60–70.


13. Marx RE, Carlson ER, Eichstaedt RM, Schimmele SR, Strauss JE, Georgeff KR. Platelet-rich plasma: growth factor enhancement for bone grafts. Oral Surg Oral Med Oral Pathol Oral Radiol Endod. 1998. 85(6):638–646.
14. Mazor Z, Peleg M, Garg AK, Luboshitz J. Platelet-rich plasma for bone graft enhancement in sinus floor augmentation with simultaneous implant placement: patient series study. Implant Dent. 2004. 13(1):65–72.


15. Ogino Y, Ayukawa Y, Tsukiyama Y, Koyano K. The effect of platelet-rich plasma on the cellular response of rat bone marrow cells in vitro. Oral Surg Oral Med Oral Pathol Oral Radiol Endod. 2005. 100(3):302–307.


16. Tomoyasu A, Higashio K, Kanomata K, et al. Platelet-rich plasma stimulates osteoblastic differentiation in the presence of BMPs. Biochem Biophys Res Commun. 2007. 361(1):62–67.


17. de Mos M, van der Windt AE, Jahr H, et al. Can plateletrich plasma enhance tendon repair? A cell culture study. Am J Sports Med. 2008. 36(6):1171–1178.
18. Nishimoto S, Oyama T, Matsuda K. Simultaneous concentration of platelets and marrow cells: a simple and useful technique to obtain source cells and growth factors for regenerative medicine. Wound Repair Regen. 2007. 15(1):156–162.


19. Forslund C, Aspenberg P. Improved healing of transected rabbit Achilles tendon after a single injection of cartilage-derived morphogenetic protein-2. Am J Sports Med. 2003. 31(4):555–559.


20. Jones CM, Dale L, Hogan BL, Wright CV, Smith JC. Bone morphogenetic protein-4 (BMP-4) acts during gastrula stages to cause ventralization of Xenopus embryos. Development. 1996. 122(5):1545–1554.


21. Wozney JM, Rosen V. Bone morphogenetic protein and bone morphogenetic protein gene family in bone formation and repair. Clin Orthop Relat Res. 1998. (346):26–37.


22. Rodeo SA, Arnoczky SP, Torzilli PA, Hidaka C, Warren RF. Tendon-healing in a bone tunnel: a biomechanical and histological study in the dog. J Bone Joint Surg Am. 1993. 75(12):1795–1803.


23. Lu H, Qin L, Lee K, Wong W, Chan K, Leung K. Healing compared between bone to tendon and cartilage to tendon in a partial inferior patellectomy model in rabbits. Clin J Sport Med. 2008. 18(1):62–69.


24. Cooper RR, Misol S. Tendon and ligament insertion: a light and electron microscopic study. J Bone Joint Surg Am. 1970. 52(1):1–20.
25. Woo SL, Orlando CA, Gomez MA, Frank CB, Akeson WH. Tensile properties of the medial collateral ligament as a function of age. J Orthop Res. 1986. 4(2):133–141.


26. Aspenberg P, Forslund C. Bone morphogenetic proteins and tendon repair. Scand J Med Sci Sports. 2000. 10(6):372–375.


27. Chong AK, Ang AD, Goh JC, et al. Bone marrow-derived mesenchymal stem cells influence early tendon-healing in a rabbit achilles tendon model. J Bone Joint Surg Am. 2007. 89(1):74–81.


28. Rodeo SA, Suzuki K, Deng XH, Wozney J, Warren RF. Use of recombinant human bone morphogenetic protein-2 to enhance tendon healing in a bone tunnel. Am J Sports Med. 1999. 27(4):476–488.


29. Jackson MR, MacPhee MJ, Drohan WN, Alving BM. Fibrin sealant: current and potential clinical applications. Blood Coagul Fibrinolysis. 1996. 7(8):737–746.