Abstract
Purpose
This study was aimed to evaluate the effect of implant drilling speed on the composition of particle size of collected bone debris.
Methods
Brånemark System® drills were used to collect bone debris from 10 drilling holes (1 unit) at 1,500 rpm (Group A) and 800 rpm (Group B) in bovine mandible. After separating particles by size into > 500 µm, between 250 µm and 500 µm, and < 250 µm fractions, particle wet volume, dry volume, and weight were measured and the proportion of 3 fractions of bone debris to total wet volume, dry volume and weight was calculated as wet volume % , dry volume % and weight %.
Endoosseous oral implantation has proven to be a successful approach for dental rehabilitation. This procedure often requires alveolar bone reconstruction in the maxillary and mandibular regions. Of the various procedures used for alveolar bone augmentation, guided bone regeneration (GBR) using autogenous bone, is well documented1-4).
Various graft materials has been used during the GBR procedure. Recently, various growth factors and cytokines have been studied to use for accelerating regeneration5-7). However, Autogenous bone is still considered as the gold standard during the GBR procedure due to its high biocompatibility and healing potential8,9). Autogenous bone in particulate form could be collected using hand chisels, burs, or bone collectors. Several studies showed bone debris obtained from implant drilling sites could be effective for covering exposed implant threads10-13).
The quality of bone debris could be influenced by the drilling conditions, such as, the drilling speed, the drilling time, the drill configuration (pressure, diameter, blade positioning, and angulation) and the coolant delivery system. Particle size of autogenous bone could influence on the induction of vital osteoblasts12). Zaner and Yukna14) concluded that a particle size between 300 µm and 500 µm is appropriate for periodontal surgery. Although bone debris obtained from implant drilling was used in many studies, little is known about the relationship between drilling speed and bone debris particle size during implant surgery. The aim of the study is to evaluate the effect of implant drilling speed on the composition of particle size of collected bone debris in an in vitro bovine model.
The implant drilling for collection of bone debris was performed on two lateral portions of dead bovine mandibles (Fig. 1a). Periosteum was elevated with periosteal elevators in order to expose mandibular body. This method is a modification of a previously described experimental model15).
The experiment groups were divided into Group A and B according to drilling speed. In Group A, bone debris was collected from 10 drilling holes (1 unit) at 1,500 rpm. In Group B, bone debris was collected in the same manner except drilling speed at 800 rpm.
Brånemark System® (Nobel Biocare AB. Göteborg. Sweden) drills were used to make drilling holes and collect bone debris. The drill system was connected to a KaVo INTRAsurg 300 plus® system (KaVo, Lake Zurich, IL) and set at 800 rpm or 1500 rpm. Drilling was performed according to the manufacturer's recommendations. Each drilling site was prepared up to a depth of 10 mm using a Guide drill, a Twist drill Ø2.0 mm, a Pilot drill, and a Twist drill Ø3.0 mm under copious irrigation, which is the standard drilling procedure when preparing a Regular Platform (RP) implant in type II bone.
Bone debris was collected to small bowl after the drilling procedures using saline irrigation. On one bony surface, total 50 osteotomies (holes) were randomly drilled. All bone debris obtained from the 10 holes was defined as 1 unit. To achieve statistical power, debris was collected from 10 units per group. The particles were divided into 3 fractions using sieves (Chunggye Co. Seoul, South Korea) (Fig. 1b); particles>500 µm sizes (LP), particles between 250 µm and 500 µm (MP), and particles < 250 µm (SP). Fraction volume and weight were measured. For wet volume measurements, bone debris was packed into each 2 cc syringes. Dry volume was measured after drying bone debris for 72 hours at room temperature. Weight was measured using a MX microbalance electronic-scale® (Mettler-Toledo Co. Sweden-America). Also the proportion of 3 fractions of bone debris to total wet volume, dry volume and weight was expressed as wet volume %, dry volume % and weight %.
The shapes and sizes of dried particles were determined using an Olympus® optical microscope (Olympus Co. Tokyo. Japan) fitted with a photoautomatic camera (Wild Leitz Mp551, Germany).
Mann-Whitney test were used to compare Group A and B of wet volumes, dry volumes and dry weight in total, LP, MP and SP fractions. Wilcoxon's signed rank test was used to compare wet volumes and dry volumes in each groups of Group A and B. Statistical significance was accepted for P values of < 0.05.
There was no difference between two groups in the shape of bone debris. In LP fractions, clustered particles were observed, and wood shaving-like particles were observed. In MP and SP fractions, wood shaving-like particle was not found and the difference was not observed each other except particle size (Fig. 2).
Wet volume, dry volume, and weight of drilling debris obtained from Group A (1,500 rpm) and Group B (800 rpm) are shown in Table 1. Total wet volumes (P = 0.1505), total dry volumes (P = 0.2324), and total weights (P = 0.6226) showed no statistically significant differences between Group A and Group B . Wet volume (P = 0.0312) of MP fractions and wet volume (P = 0.0213) and weight (P = 0.0015) of SP fractions were significantly greater in Group A. However, no significant differences were observed between wet volumes, dry volumes, or particle weights of the LP fractions of the two groups.
Ratios of summed measurements are shown in Fig. 3. Wet volume, dry volume and weight between 2 groups were compared in Total, LP, MP and SP fractions. In LP fractions, wet volume section of Group A and Group B showed 86.46%, 93.28% respectively. The difference was statistically significant (P = 0.0059) between two groups. Group B (84.79%) in the dry volume also showed significantly higher percentages than Group A (77.91%) (P = 0.0272). Group B in weight also showed higher percentage than Group A. However, the difference was not statistically significant. In MP fractions, Group A and Group B each showed 7.25%, 3.82% in the wet volume section and 14.00%, 12.19% in the dry volume section. Group A was significantly greater than Group B in wet and dry volume sections (P = 0.0029, P = 0.0312). However, there was no significant difference in the weight %. In SP fractions, Group A was acquired the significantly higher value only in wet volume % (P = 0.0023).
Wet volume was significantly greater than dry volume in the LP fractions of Group A (P = 0.0006) and Group B (P = 0.0040). However, in the MP (Group A: P = 0.0012, Group B : P = 0.0020) and SP fractions (Group A: P = 0.0061, Group B: P = 0.0115), dry volumes were greater than wet volumes.
In summed wet volumes % of the MP and LP fractions (the particle sizes ≥ 250 µm) of Group B, a statistically significant increase (P = 0.0023) was found compared with Group A. On the other hand, wet volumes, dry volumes, weights, wet volumes %, and dry volume % in the summed SP and MP fractions (the particle sizes ≤ 500 µm) were greater in Group A than in Group B (P = 0.0045; P = 0.0324; P = 0.0432; P = 0.0059; and P = 0.0272, respectively).
Sufficient width and depth of alveolar ridge are important in implant placement. Sometimes, implant fixtures were not completely covered by bony architecture. In cases only small size of dehiscence is found, a localized autogenous bone graft using implant drilling debris is recommended rather than performing major surgery using autogenous bone from the mandibular ramus or chin11-13).
When bone debris is collected for this purpose, every possible factor should be considered to achieve appropriate graft material. Several recent studies have focused on the risk of osseous damage at elevated drilling speeds during implant surgery16-18). Also, the possibilities of bone necrosis of drilling debris by mechanical manipulation, pressure, and temperature have been investigated19). A drilling speed of less than 2000 rpm with an irrigation system was not found to generate excessive heat during drilling20,21). Furthermore, Reingewirtz et al.22) recommended the use of less than 800 rpm in dense bone to prevent overheating. Anitua et al.23) suggested that drilling at a speed of only 50 rpm could reduce damage to the host tissue and obtain a mass of living bone.
Based on above evidence, drill speeds of 1,500 rpm (Group A) and 800 rpm (Group B) were selected in this experiment. No significant difference was found between the two groups in the total weight and volume of collected debris. In this study, mean dry volume per site in Groups A and B was 0 .173 ± 0 .025 mL (1.73 ± 0.25/10 drillings) and 0.163 ± 0.018 mL (1.63 ± 0.18/10 drillings) respectively. In Savant's study24), 0.195 ± 0.099 mL of implant debris dry volume was obtained per site. These differences came from the use of different drilling depth and collecting devices. In Savant's study, drilling depth was longer than this study and they used bone collector which could not collect small size of bone debris.
Many studies suggested that the particle size of the grafting material could effect on the osteogenic ability. Lee et al.25) suggested that the autogenous drilling dusts showed better results in regeneration for dehiscence-type defects. Zaner and Yukna14) argued that the appropriate particle size of graft material for periodontal surgery was 300 to 500 µm. Moreover, Mellonig26), in an in vitro study, concluded that particle sizes between 250 and 1,000 µm are significantly better than particle sizes of ≤ 250 µm in terms of bone growth rate. Shapoff et al.27) studied the relation between particle size and enhanced osteogenesis and concluded that; (1) particle sizes between 125 µm and 1,000 µm had increased surface area, which promotes bone formation, (2) an increase in surface area, with an increase in osteoclastic activity, could result in enhanced osteogenic induction, and (3) graft particles in the range of 125 to 1,000 µm could stimulate osteogenesis by increasing the number of pores. It was also suggested that an increase in particle surface-to-volume ratio increases bone morphogenic protein levels. Sampath and Reddi28) reported that the subcutaneous implantation of coarse powders (74 to 420 µm) of demineralized bone matrix results in the local differentiation of bone, whereas the implantation of a 44 to 77 µm sized particles does not. In the present study, total wet volume was not different in two groups. However, the percentage of >250 µm particle was higher in 800rpm than in 1500 rpm significantly.
The results of the present study showed that wet volume was greater than dry volume only in the LP fractions (> 500 µm) of both groups. In a previous clinical study, wet-conditioned bone particles were found to have a larger volume than dry bone particles24). These volumetric changes must be considered during the use of commercial xenograft or allograft products because graft material was soaked in saline for easy handling during the GBR procedure.
Despite of the small number of bone samples and small amount of acquired bone debris, this study showed that the drilling speed influenced the composition of particle size in collected drilling bone debris. The drilling in 800 rpm produced the more percentage of large particles than the drilling in 1,500 rpm. However, the drilling speed didn't effect on total volume of and weight of bone debris. Further, other factors, i.e. drill's shape, dillt's sequential procedure, should be investigated under in vitro and in vivo.
Figures and Tables
Figure 1
(A) Drilling sites in the bovine mandible. To reduce errors, drill holes were blocked with wax before the next drilling. (B) Two sieves (250 µm and 500 µm) were used tofractionate particles by size.
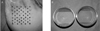
Figure 2
Microscopic view of bone debris. (A) Wood shaving-like particles shown in LP fraction. (B) Particles shown in MP and SP fraction. (Original magnification × 40).
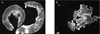
Figure 3
Mean fractional percentages for (A) wet volume, (B) dry volume and (C) particle weights from 10 units. LP=particles > 500 µm, MP=particles between 250 and 500 µm, SP=particles < 250 µm.
*: statistically significant between Group A and B (P < 0.05).
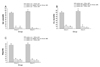
References
1. Lee Y, Han S, Um H, Kim D. Histologic observation of regenerated bone in human intraosseous Lesion following guided tissue regeneration with calcium carbonate implant and autogenous bone graft. J Korean Acad Periodontol. 1998. 28:263–273.


2. Becker W, Schenk R, Higuchi K, Lekholm U, Becker BE. Variations in bone regeneration adjacent to implants augmented with barrier membranes alone or with demineralized freeze-dried bone or autologous grafts: a study in dogs. Int J Oral Maxillofac Implants. 1995. 10:143–154.
3. Block MS, Kent JN, Kallukaran FU, Thunthy K, Weinberg R. Bone maintenance 5 to 10 years after sinus grafting. J Oral Maxillofac Surg. 1998. 56:706–714.


4. Marx RE. Clinical application of bone biology to mandibular and maxillary reconstruction. Clin Plast Surg. 1994. 21:377–392.


5. Seol Y, Lee J, Kye S, et al. The effect of PDGF-BB loaded TCP/chitosan microgranules on new bone formation. J Korean Acad Periodontol. 2002. 32:489–500.


6. The effect of PDFG-BB loaded biodeqradable membrane on early healinq stage in quided tissue reqeneration. J Korean Acad Periodontol. 1999. 29:507–519.
7. Cheong H, Kim K, Park Y, et al. Osteogenic effects of polyethyleneimine-condensed BMP-2 genes in vitro and in vivo. J Korean Acad Periodontol. 2007. 37:859–869.


8. Nkenke E, Radespiel-Troger M, Wiltfang J, et al. Morbidity of harvesting of retromolar bone grafts: a prospective study. Clin Oral Implants Res. 2002. 13:514–521.


9. Schlegel KA, Fichtner G, Schultze-Mosgau S, Wiltfang J. Histologic findings in sinus augmentation with autogenous bone chips versus a bovine bone substitute. Int J Oral Maxillofac Implants. 2003. 18:53–58.
10. Karacaoglan N, Uysal OA. Use of iliac bone graft for saddle nose deformity. Auris Nasus Larynx. 1998. 25:49–57.


11. Lauer G, Schilli W. Collected implant cavity borings used as peri-implant osseous augmentation material. Int J Oral Maxillofac Implants. 1994. 9:437–443.
12. Widmark G, Ivanoff CJ. Augmentation of exposed implant threads with autogenous bone chips: prospective clinical study. Clin Implant Dent Relat Res. 2000. 2:178–183.


13. Young MP, Worthington HV, Lloyd RE, et al. Bone collected during dental implant surgery: a clinical and histological study. Clin Oral Implants Res. 2002. 13:298–303.


14. Zaner DJ, Yukna RA. Particle size of periodontal bone grafting materials. J Periodontol. 1984. 55:406–409.


15. Kainulainen VT, Kainulainen TJ, Oikarinen KS, Carmichael RP, Sandor GK. Performance of six bone collectors designed for dental implant surgery. Clin Oral Implants Res. 2006. 17:282–287.


16. Iyer S, Weiss C, Mehta A. Effects of drill speed on heat production and the rate and quality of bone formation in dental implant osteotomies. Part II: Relationship between drill speed and healing. Int J Prosthodont. 1997. 10:536–540.
17. Iyer S, Weiss C, Mehta A. Effects of drill speed on heat production and the rate and quality of bone formation in dental implant osteotomies. Part I: Relationship between drill speed and heat production. Int J Prosthodont. 1997. 10:411–414.
18. Sharawy M, Misch CE, Weller N, Tehemar S. Heat generation during implant drilling: the significance of motor speed. J Oral Maxillofac Surg. 2002. 60:1160–1169.


19. Hoegel F, Mueller CA, Peter R, Pfister U, Suedkamp NP. Bone debris: dead matter or vital osteoblasts. J Trauma. 2004. 56:363–367.


20. Eriksson RA, Adell R. Temperatures during drilling for the placement of implants using the osseointegration technique. J Oral Maxillofac Surg. 1986. 44:4–7.


21. Marx RE, Miller RI, Ehler WJ, Hubbard G, Malinin TI. A comparison of particulate allogeneic and particulate autogenous bone grafts into maxillary alveolar clefts in dogs. J Oral Maxillofac Surg. 1984. 42:3–9.


22. Reingewirtz Y, Szmukler-Moncler S, Senger B. Influence of different parameters on bone heating and drilling time in implantology. Clin Oral Implants Res. 1997. 8:189–197.


23. Anitua E, Carda C, Andia I. A novel drilling procedure and subsequent bone autograft preparation: a technical note. Int J Oral Maxillofac Implants. 2007. 22:138–145.
24. Savant TD, Smith KS, Sullivan SM, Owen WL. Bone volume collected from dental implant sites during osteotomy. J Oral Maxillofac Surg. 2001. 59:905–907.


25. Lee SH, Yoon HJ, Park MK, Kim YS. Guided bone regeneration with the combined use of resorbable membranes and autogenous drilling dust or xenografts for the treatment of dehiscence-type defects around implants: an experimental study in dogs. Int J Oral Maxillofac Implants. 2008. 23:1089–1094.
26. Mellonig JT. Decalcified freeze-dried bone allograft as an implant material in human periodontal defects. Int J Periodontics Restorative Dent. 1984. 4:40–55.