This article has been corrected. See "Erratum: Reduced Frontal P3a Amplitude in Migraine Patients during the Pain-Free Period" in Volume 9 on page 200.
Abstract
Background and Purpose
Neuropsychological and neuroimaging studies both suggest that frontal lobe dysfunction is present in migraineurs. Since P3a abnormalities manifest in other diseases associated with attention problems, such as attention deficit hyperactivity disorder, we hypothesized that migraine patients have P3a abnormalities, particularly in the frontal region.
Methods
Event-related potentials were measured using a passive auditory oddball paradigm in 16 female migraineurs (aged 22.9±2.0 years, mean±SD) during the interictal period and in 16 age-matched healthy females (22.6±2.0 years). The amplitudes and latencies were analyzed independently using repeated-measures analysis of variance. Nonparametric statistical testing using a cluster-level randomization method was performed to localize the abnormalities.
Results
The mean P3a amplitude at frontal areas during the third trials was significantly lower in migraineurs (1.06 µV) than in controls (1.69 µV, p=0.026). P3a amplitudes were negatively correlated with the duration of the migraine history (r=-0.618, p=0.014). Cluster-based nonparametric statistical analysis showed that the amplitudes over left frontal areas were significantly lower in migraine patients than in controls.
Conclusions
A reduced P3a amplitude of migraineurs reflects attentional deficits and frontal dysfunction. The negative correlation between P3a amplitude and the duration of the migraine history suggests that attentional deficits and frontal dysfunction are either the cause or the result of headache.
Migraine is one of the most common pain disorders.1 Pain has a conceptual multidimensional basis consisting of sensory-discriminative, affective-motivational, and cognitive-evaluative components.2 In conjunction with the other components, manipulating the cognitive-evaluative component can influence pain; for example, coping strategies based on selective attention, such as distraction, sensory monitoring, and suppression, are used to reduce pain.3 Although migraine does not progress generally,4 progression of migraine is known and can be classified into clinical transformation, physiologic transformation, and anatomic progression.5
Several neuropsychological studies have evaluated the cognitive-evaluative component in migraine patients. Although the results are controversial, migraineurs were reported to have cognitive dysfunctions in memory and attention,6,7 verbal performance,8 orbitofrontal function,9 and executive function.10,11 The brains of migraineurs are also known to have structural and functional abnormalities. Some functional neuroimaging st-udies using voxel-based morphometry (VBM)11,12 and PET13,14 have revealed cortical dysfunction in migraineurs, particularly in the frontal lobe. One study also found that the gray-matter volume reduced progressively as the headache duration and frequency increased,15 representing evidence of anatomic progression.
Physiologic transformation during migraine chronification may be revealed by event-related potentials (ERPs). The ERP components are related to underlying brain activities during specific cognitive processes. In contrast to neuroimaging tools, which have excellent spatial resolutions but relatively poor temporal resolutions, ERPs allow brain activity to be detected at millisecond-level resolution.16 Among the ERP components, the P3 component represents aspects of information processing, such as attention allocation and decision making.17 P3 consists of two subcomponents called 'P3a' and 'P3b',18 which have different scalp topographies and cortical sources. P3b is associated with attention and subsequent memory process, and is located at the temporal-parietal area,18 while P3a reflects the automatic allocation of attention, and shows a more anterior distribution (i.e., in frontocentral areas).19 Studies of P3a have revealed abnormalities in attention20,21 and executive function.22 P3a could be a good component to investigate not only from the cognitive-evaluative aspect but also for detecting possible frontal dysfunction in migraineurs.
We hypothesized that migraine patients have P3a abnormalities, particularly in the frontal region, since previous neuropsychological and neuroimaging studies have suggested the presence of frontal lobe dysfunction in migraineurs. Although Wang and Schoenen23 studied the P3a amplitude in migraine, they were unable to reveal the spatial distribution of these abnormalities because they measured P3a using only a single electrode at the Cz location.
The aim of the present study was to elucidate possible abnormalities of P3a component, suggesting alterations of the cognitive-evaluative component in migraine, and to define specific brain regions of P3a abnormality in migraine by performing ERP studies using a passive auditory oddball paradigm with multichannel electroencephalography (EEG) recording covering the entire scalp. P3a abnormalities were correlated with clinical manifestations in order to identify their clinical significance.
Female subjects who fulfilled the diagnostic criteria of migraine with or without aura, according to the International Cla-ssification of Headache Disorder (2nd edition),24 were initially considered for the present study. All patients underwent a standardized interview using a structured questionnaire and clinical neurological examinations by a neurologist (J.K.Y.). Clinical data were obtained by interview, and included 1) the duration of the migraine history, 2) the average number of headaches per month during the previous year, and 3) rating the most severe headache experienced during the previous year using a visual analog scale (VAS).
The exclusion criteria were as follows: 1) taking prophylactic medications for migraine, 2) a history of analgesic drug overuse, 3) general neurological or psychiatric disease, as described by the Diagnostic and Statistical Manual of Mental Disorders (4th edition), 4) a history of drug abuse or dependency, including that related to alcohol consumption and cigarette smoking, 5) a history of mixed headache types, 6) a history of a neurological disorder or abnormal findings on neuro-logic examination, or 7) impaired auditory function. However, subjects who intermittently used rescue medications, such as acetaminophen, nonsteroidal anti-inflammatory drugs, and triptans, were not excluded.
Age-matched healthy female volunteers who responded to an advertisement at Korea University participated in the study as controls. These volunteers were also interviewed using a st-ructured questionnaire and examined by a neurologist (J.K.Y.). All of the participants provided informed consents in accordance with the guidelines issued by the institutional review board of the Korea University Medical Center after they had been informed about the study procedures.
EEG recordings were made using 27 electrodes placed on the scalp in accordance with the international 10-20 system, but with extended coverage of the lower temporal region (F9/10, T9/10, and P9/10). The reference electrode was Pz, impedances were kept below 5 kΩ, and the bandpass filter setting used was 0.1-70 Hz with a sampling rate of 400 Hz. Two electrooculography channels (placed on the left and right outer canthi) were added to confirm eyeball movements and allow the related electrooculography artifacts to be removed. Participants were seated in a comfortable chair and listened to auditory stimuli through earphones. All subjects were headache free for at least 72 hours both before and after the EEG data were obtained (the latter time was verified by telephone interview).
Subjects were instructed to read a book and to ignore auditory stimuli during the task. The employed oddball paradigm was based on sequences of two tones: either a 1000-Hz standard (p=0.8, n=960) tone followed by a 1030-Hz deviant (p=0.2, n= 240) tone, or vice versa, with each tone starting on a random basis. The tone duration was 100 ms with rise and fall times of 10 ms, and the interstimulus interval was 1000 ms. Each subject received three trials of an auditory oddball task containing a mixture of 400 tones.
EEG data were analyzed using EEGLAB version 6.03b25 and Fieldtrip,26 and an open-source toolbox operated in the MAT-LAB environment (version 7.01, MathWorks, Natick, MA, USA). EEG data were re-referenced to the average reference, and the bandpass filter was set at 0.5-50 Hz. Epochs were then extracted using a time window from -200 to +800 ms relative to stimulus onset. Baselines were corrected by subtracting the root-mean-square values of prestimulus intervals from entire epoch lengths.
ERP latencies and amplitudes were measured relative to the prestimulus baseline period. The ERP negative peak that appeared between 75 and 125 ms after an auditory stimulation was used to define the N1 component, while the positive peak between 140 and 190 ms, the negative peak between 200 and 250 ms, and the positive peak between 255 and 395 ms were used to define the P2, N2, and P3a components, respectively (Figs. 1 and 2A).
The amplitudes and latencies of all ERP components were analyzed independently using repeated-measures analysis of variance (ANOVA) with a mixed design. The within-subject variables were stimulus (two values: deviant and standard), trial (three values: first, second, and third), location 1 (three values: frontal, central, and parietal), and location 2 (three values: left, middle, and right), while the between-subject variable was group (migraine vs. control). Significant results were further analyzed using Bonferroni's post-hoc analysis. Correlations between ERP amplitudes and latencies and durations of the headache history (years), headache frequencies (attacks/month), and severities of pain (VAS score, from 0 to 10) were analyzed by Pearson's test.
To confirm the statistical results and to extend topographic differences in parasagittal head areas to the entire head, ERP data from all electrodes and from each time frame were subjected to cluster-based nonparametric statistical tests using Fieldtrip.27 The type I error rate of multiple comparisons was controlled using a nonparametric randomization test incorporating the cluster-level randomization method, which identifies electrodes showing significant differences between migraine patients and controls. This method uses the cluster that differs the most between the two groups to calculate a critical value for statistical significance, under the null distribution for this test statistic, using a permutation method (the Monte Carlo approximation). Statistical significance was accepted for p values of <0.05 throughout the study.
Sixteen migraine patients (3 of whom had migraine with aura) and 16 healthy controls participated in this study. The mean age did not differ between patients (22.9±2.0 years, mean±SD) and control subjects (22.6±2.0). The duration of the migraine history in the patient group was 6.8±4.1 years. The migraine frequency was 6.1±7.5 attacks per month, and the headache severity (as scored on the VAS) was 6.8±1.1.
Because we used a passive oddball paradigm in this study, reaction times and correct response rates were not obtained. ERP waveforms from 21 electrodes and ERP components are sh-own in Fig. 1. The latencies and amplitudes of the N1, P2, and N2 components did not differ between standard and deviant tones.
The mean P3a amplitude at frontal areas (F3, Fz, and F4 electrodes) during the third trial was significantly lower in migraineurs (1.06 µV) than in controls (1.69 µV, p=0.026) (Fig. 2). Because the P3a component was produced only by deviant tones, repeated-measures ANOVAs did not include the effect of 'stimulus'.
The amplitudes of P3a showed significant main effects for 'location 1' and 'location 2' (Table 1). There were marginal interactions between 'location 1' and 'group' (F1.27,37.98=3.00, p= 0.082) and between 'trial' and 'group' (F2,60=2.47, p=0.093), and no 'location 1'×'group' or 'trial'×'group' interactions in the post-hoc analysis. Latencies of the P3a component did not show any significant effects.
Fig. 2B shows that the amplitude of P3a in the frontal electrodes was asymmetric in migraineurs and symmetric in controls. Cluster-based nonparametric statistical analysis showed that the mean amplitude over left frontal areas was significantly lower in migraine patients than in controls at 260-280 ms after deviant tones (Fig. 2C).
Repeated-measures ANOVA with the group (migraineurs vs. controls) as the between-subject variables and the trial (first vs. second vs. third) and electrodes (F3 vs. Fz vs. F4) as the within-subject variables revealed a significant 'trial'×'group' interaction (F2,60=3.20, p=0.048) (Table 2). A significant difference between P3a amplitudes in patients and controls was observed only in the third trial of the experiment. Post-hoc analysis showed that the P3a amplitude for the third trial was lower in migraineurs (1.06 µV) than in controls (1.69 µV, p=0.026) at the frontal electrodes (F3, Fz, and F4) (Fig. 3). We also compared the P3a amplitude for the third trial between migraineurs and controls at each electrode (F3, Fz, and F4). At the F3 and Fz electrodes, the P3a amplitudes for the third trial were significantly lower in migraineurs than in controls (1.05±0.68 vs. 1.76±1.05 µV, p=0.031; and 1.03±0.79 vs. 1.81±0.98 µV, p= 0.019; respectively) At the F4 electrodes, the P3a amplitudes for the third trial did not differ between migraineurs and controls.
Correlation analysis showed that P3a amplitudes at the Fz electrode were negatively correlated with the duration of the migraine history (r=-0.618, p=0.014) (Fig. 4). P3a amplitudes at the F3 electrode also showed a tendency to be negatively correlated with the duration of the migraine history (r=-0.461, p=0.084). The headache frequency and the most severe headache episode (as scored on the VAS) during the previous year were not correlated with P3a amplitudes or latencies.
This study used a passive auditory oddball paradigm to compare cognitive ERP responses between migraine patients and control subjects. Our main findings are that migraine patients showed a lower P3a amplitude in the left frontal area compared to the controls, and that this was negatively correlated with the duration of the migraine history.
P3a is known to be related to involuntarily captured attention and orienting responses.18 Therefore, the reduced P3a amplitude in our study might be consistent with previous neuropsychological findings of dysfunction in involuntary attentio-nal shift.28-30 We also analyzed the location of the reduced P3a amplitude, which revealed decreases in the frontal area. This result supports previous studies showing frontal lobe dysfunction in migraineurs, such as executive dysfunction,10,11 decre-ased gray-matter density in the frontal cortex shown by VBM,11 and decreased EEG alpha activity in the frontal cortex shown by low resolution brain electromagnetic tomography.31 Pain is known to be modulated by attentional mechanisms during parallel cognitive processing, which involves the frontal cortex. 32 The frontal cortex may exert top-down influences on the periaqueductal gray matter and posterior thalamus to inhibit pain perception.33-35 Therefore, frontal dysfunction might also produce abnormal pain perception, making migraineurs susceptible to triggers such as various sensory stimuli.
While P3a in control subjects was maximal over the frontocentral region with bilateral symmetry, migraine patients show-ed an asymmetric P3a distribution (lower in the left frontal reregion), which is similar to the findings of a fMRI study.36 A recent low resolution brain electromagnetic tomography study also found that the EEG absolute power, localized to the left hemisphere, decreased with increasing headache intensity.37 Furthermore, impaired verbal memory6 and poor verbal performance8,38 in migraineurs are also indicative of left hemispheric dysfunction. Thus, it seems that the left frontal lobe might be more vulnerable in migraineurs, although the underlying mechanism is unclear.
We found that the P3a amplitude was negatively correlated with the duration of the migraine history. Similarly, recent studies involving VBM12 and PET14 and neuropsychological evaluations7,10 showed that structural and functional declines in the cerebral cortex were negatively related to the duration of the migraine history. As stated above, frontal and attentional dysfunction may result in abnormal pain perception; in other words, frontal and attentional dysfunction represented by a reduced P3a amplitude seems to cause abnormal pain processing. This explanation is based on the attentional control of pain, since migraine is also a pain disorder. Coping strategies such as distraction, sensory monitoring, and suppression are used to reduce pain.3 Patients with chronic pain are thought to have problems with attention or information processing, which produces maladaptive behaviors such as catastrophizing cognition, resulting in chronification of pain. Since the reduced P3a amplitude in migraineurs in our study also represent abnormalities of attention and information processing, an abnormal frontal P3a might also contribute to the chronification of migraine.
On the other hand the opposite explanation is also possible, with a reduced P3a amplitude resulting from migraine rather than being a cause of migraine. Eccleston's hypothesis states that pain demands resources of attention.39 In this context, acute pain has been shown to reduce the P3 amplitude.40 Another study using fMRI showed that attention-specific networks can be modulated by pain.41 However, the migraineurs in our study showed a reduced P3a amplitude during the interictal state, and hence recurrent pain might have produced a con-stant abnormality of brain. A recent study revealed cognitive impairment in children with migraine, and suggested that chronic, recurrent migraine attacks will result in cognitive impairment. 38 They postulated their hypothesis based on the cortical42 and subcortical43 structures of migraineurs being affected by repeated activation of several cortical or subcortical neuronal networks.
While migraine is generally not considered to be a progressive disease, chronification of migraine can occur.4 One of the most important risk factors for migraine progression is the attack frequency.44 Reduced frontal P3a, as a physiologic transformation, might be a clinical marker for migraine progression irrespective of whether this abnormality is the cause or result of chronic headache-reduced frontal P3a might actually be both the cause and the result, creating a vicious cycle leading to chronification of migraine. A recent epidemiologic study45 in Korea shows that the prevalence rate of migraine is 6.1% and the prevalence was highest in women aged 40-49 years and men aged 19-29 years. Also, the incidence rate is known to peak in young ages (ages of 10-11 years in boys and ages of 14-17 years in girls).46,47 Therefore, early diagnosis and intervention may be important to preventing any deterioration in cognitive functions or migraine chronification.
The absence of habituation is generally considered to be a consistent main finding of ERP studies in migraine patients, 23,48-52 but this was not observed in migraine patients in some other studies.53-56 Furthermore, abnormal habituation has been reportedly found even in healthy controls.57,58 Polich et al.59 showed that P3 habituation could not be obtained during passive oddball paradigm. Similarly, our study also showed that P3a habituation was absent in the normal controls. In contrast, P3a amplitudes in migraineurs decreased across the trials (Fig. 3), indicating the presence of habituation; however, this phenomenon was not statistically significant.
Our study revealed that the mean P3a amplitude during the third trial was significantly lower in migraineurs than in controls, which suggests that migraineurs experience problems when cognitive stimuli are given repetitively for a sufficiently long duration.
Some limitations of the present study require consideration. First, we did not measure ERPs in conjunction with neuropsychological investigations. However, our patients were young and it was assumed that a neuropsychological study would have revealed at least normal cognitive function in them. Second, the small cohort limited the ability to examine the effects of gender and age and the differences between migraine with aura and migraine without aura. Nevertheless, we took steps to ensure that the sample was as homogeneous as possible by choosing only young females, which undoubtedly reduced the impact of confounders on our analysis.
In conclusion, our study suggests that migraineurs have a reduced P3a amplitude in the frontal region, which suggests cognitive dysfunction. Furthermore, frontal P3a amplitudes were found to be negatively related to the duration of the illness, which further suggests that frontal dysfunction in migraineurs results from repeated migraine attacks.
Figures and Tables
Fig. 1
Grand average event-related potential responses to standard and deviant auditory stimuli recorded from 21 electrodes. Red lines represent plots from migraineurs, and blue lines plots from controls. Solid lines represent plots for deviant tones, and dotted lines those for standard tones.
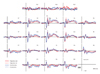
Fig. 2
Voltage topographic scalp maps of the P3a component (B) and of event-related potential (ERP) differences between patients and normal controls at 260-280 ms after deviant tones (C). Red denotes a positive and blue a negative potential. Significant electrodes are highlighted, shown by cluster-based nonparametric statistical analysis (p<0.05). The P3a component is indicated by a rectangle on the grand average ERP plot at the Fz electrode (A).
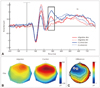
Fig. 3
P3a amplitudes of successive blocks at frontal areas (F3, Fz, and F4 electrodes). Mean P3a amplitudes only significantly differed between controls and migraineurs during the third block (p=0.026).

References
1. Lipton RB, Bigal ME. Migraine: epidemiology, impact, and risk factors for progression. Headache. 2005. 45:Suppl 1. S3–S13.


2. Schneider F, Karoly P. Conceptions of the pain experience: the emergence of multidimensional models and their implications for contemporary clinical-practice. Clin Psychol Rev. 1983. 3:61–86.


3. Hasenbring M. Attentional control of pain and the process of chronification. Prog Brain Res. 2000. 129:525–534.


4. Goadsby PJ. Is migraine a progressive disorder? Considering the clinical implications of new research data on migraine and brain lesions. Med J Aust. 2005. 182:103–104.
5. Lipton RB, Bigal ME. Looking to the future: research designs for study of headache disease progression. Headache. 2008. 48:58–66.


6. Aguggia M, Saracco MG. Pathophysiology of migraine chronification. Neurol Sci. 2010. 31:Suppl 1. S15–S17.


7. Le Pira F, Zappalà G, Giuffrida S, Lo Bartolo ML, Reggio E, Morana R, et al. Memory disturbances in migraine with and without aura: a strategy problem? Cephalalgia. 2000. 20:475–478.


8. Calandre EP, Bembibre J, Arnedo ML, Becerra D. Cognitive disturbances and regional cerebral blood flow abnormalities in migraine patients: their relationship with the clinical manifestations of the illness. Cephalalgia. 2002. 22:291–302.


9. Waldie KE, Hausmann M, Milne BJ, Poulton R. Migraine and cognitive function: a life-course study. Neurology. 2002. 59:904–908.


10. Gómez-Beldarrain M, Carrasco M, Bilbao A, García-Moncó JC. Orbitofrontal dysfunction predicts poor prognosis in chronic migraine with medication overuse. J Headache Pain. 2011. 12:459–466.


11. Camarda C, Monastero R, Pipia C, Recca D, Camarda R. Interictal executive dysfunction in migraineurs without aura: relationship with duration and intensity of attacks. Cephalalgia. 2007. 27:1094–1100.


12. Schmitz N, Arkink EB, Mulder M, Rubia K, Admiraal-Behloul F, Schoonman GG, et al. Frontal lobe structure and executive function in migraine patients. Neurosci Lett. 2008. 440:92–96.


13. Kim JH, Suh SI, Seol HY, Oh K, Seo WK, Yu SW, et al. Regional grey matter changes in patients with migraine: a voxel-based morphometry study. Cephalalgia. 2008. 28:598–604.


14. Afridi SK, Giffin NJ, Kaube H, Friston KJ, Ward NS, Frackowiak RS, et al. A positron emission tomographic study in spontaneous migraine. Arch Neurol. 2005. 62:1270–1275.


15. Kim JH, Kim S, Suh SI, Koh SB, Park KW, Oh K. Interictal metabolic changes in episodic migraine: a voxel-based FDG-PET study. Cephalalgia. 2010. 30:53–61.


16. Schmitz N, Admiraal-Behloul F, Arkink EB, Kruit MC, Schoonman GG, Ferrari MD, et al. Attack frequency and disease duration as indicators for brain damage in migraine. Headache. 2008. 48:1044–1055.


17. Woodman GF. A brief introduction to the use of event-related potentials in studies of perception and attention. Atten Percept Psychophys. 2010. 72:2031–2046.


18. Polich J, Kok A. Cognitive and biological determinants of P300: an integrative review. Biol Psychol. 1995. 41:103–146.


19. Polich J. Updating P300: an integrative theory of P3a and P3b. Clin Neurophysiol. 2007. 118:2128–2148.


20. Volpe U, Mucci A, Bucci P, Merlotti E, Galderisi S, Maj M. The cortical generators of P3a and P3b: a LORETA study. Brain Res Bull. 2007. 73:220–230.


21. Baving L, Rellum T, Laucht M, Schmidt MH. Children with oppositional-defiant disorder display deviant attentional processing independent of ADHD symptoms. J Neural Transm. 2006. 113:685–693.


22. Banaschewski T, Brandeis D, Heinrich H, Albrecht B, Brunner E, Rothenberger A. Association of ADHD and conduct disorder--brain electrical evidence for the existence of a distinct subtype. J Child Psychol Psychiatry. 2003. 44:356–376.


23. Anderson NE, Baldridge RM, Stanford MS. P3a amplitude predicts successful treatment program completion in substance-dependent individuals. Subst Use Misuse. 2011. 46:669–677.


24. Wang W, Schoenen J. Interictal potentiation of passive "oddball" auditory event-related potentials in migraine. Cephalalgia. 1998. 18:261–265.


25. Headache Classification Subcommittee of the International Headache Society. The International Classification of Headache Disorders: 2nd edition. Cephalalgia. 2004. 24:Suppl 1. 9–160.
26. Delorme A, Makeig S. EEGLAB: an open source toolbox for analysis of single-trial EEG dynamics including independent component analysis. J Neurosci Methods. 2004. 134:9–21.


27. Oostenveld R, Fries P, Maris E, Schoffelen JM. FieldTrip: Open source software for advanced analysis of MEG, EEG, and invasive electrophysiological data. Comput Intell Neurosci. 2011. 2011:156869.


28. Maris E, Oostenveld R. Nonparametric statistical testing of EEG- and MEG-data. J Neurosci Methods. 2007. 164:177–190.


29. Vannatta K, Getzoff EA, Powers SW, Noll RB, Gerhardt CA, Hershey AD. Multiple perspectives on the psychological functioning of children with and without migraine. Headache. 2008. 48:994–1004.


30. Moutran AR, Villa TR, Diaz LA, Noffs MH, Pinto MM, Gabbai AA, et al. Migraine and cognition in children: a controlled study. Arq Neuropsiquiatr. 2011. 69:192–195.


31. Mulder EJ, Linssen WH, Passchier J, Orlebeke JF, de Geus EJ. Interictal and postictal cognitive changes in migraine. Cephalalgia. 1999. 19:557–565. discussion 541.


32. Clemens B, Bánk J, Piros P, Bessenyei M, Veto S, Tóth M, et al. Three-dimensional localization of abnormal EEG activity in migraine: a low resolution electromagnetic tomography (LORETA) study of migraine patients in the pain-free interval. Brain Topogr. 2008. 21:36–42.


33. Petrovic P, Petersson KM, Ghatan PH, Stone-Elander S, Ingvar M. Pain-related cerebral activation is altered by a distracting cognitive task. Pain. 2000. 85:19–30.


34. Valet M, Sprenger T, Boecker H, Willoch F, Rummeny E, Conrad B, et al. Distraction modulates connectivity of the cingulo-frontal cortex and the midbrain during pain--an fMRI analysis. Pain. 2004. 109:399–408.


35. Lorenz J, Minoshima S, Casey KL. Keeping pain out of mind: the role of the dorsolateral prefrontal cortex in pain modulation. Brain. 2003. 126:1079–1091.


36. Brighina F, Piazza A, Vitello G, Aloisio A, Palermo A, Daniele O, et al. rTMS of the prefrontal cortex in the treatment of chronic migraine: a pilot study. J Neurol Sci. 2004. 227:67–71.


37. Zakzanis KK, Mraz R, Graham SJ. An fMRI study of the Trail Making Test. Neuropsychologia. 2005. 43:1878–1886.


38. Faber PL, Tei S, Chen C, Hsiao P, Lehmann D. 3. Brain LORETA functional imaging, EEG spectral power, and self-rated headache pain. Clin Neurophysiol. 2011. 122:e2.


39. Parisi P, Verrotti A, Paolino MC, Urbano A, Bernabucci M, Castaldo R, et al. Headache and cognitive profile in children: a cross-sectional controlled study. J Headache Pain. 2010. 11:45–51.


40. Eccleston C, Crombez G. Pain demands attention: a cognitive-affective model of the interruptive function of pain. Psychol Bull. 1999. 125:356–366.


41. Houlihan ME, McGrath PJ, Connolly JF, Stroink G, Allen Finley G, Dick B, et al. Assessing the effect of pain on demands for attentional resources using ERPs. Int J Psychophysiol. 2004. 51:181–187.


42. Seminowicz DA, Davis KD. Pain enhances functional connectivity of a brain network evoked by performance of a cognitive task. J Neurophysiol. 2007. 97:3651–3659.


43. Seidel S, Hartl T, Weber M, Matterey S, Paul A, Riederer F, et al. Quality of sleep, fatigue and daytime sleepiness in migraine - a controlled study. Cephalalgia. 2009. 29:662–669.


44. Kruit MC, Launer LJ, Overbosch J, van Buchem MA, Ferrari MD. Iron accumulation in deep brain nuclei in migraine: a population-based magnetic resonance imaging study. Cephalalgia. 2009. 29:351–359.


45. Valeriani M, Galli F, Tarantino S, Graceffa D, Pignata E, Miliucci R, et al. Correlation between abnormal brain excitability and emotional symptomatology in paediatric migraine. Cephalalgia. 2009. 29:204–213.


46. Siniatchkin M, Kropp P, Gerber WD. What kind of habituation is impaired in migraine patients? Cephalalgia. 2003. 23:511–518.


47. Evers S, Quibeldey F, Grotemeyer KH, Suhr B, Husstedt IW. Dynamic changes of cognitive habituation and serotonin metabolism during the migraine interval. Cephalalgia. 1999. 19:485–491.


48. Evers S, Bauer B, Grotemeyer KH, Kurlemann G, Husstedt IW. Event-related potentials (P300) in primary headache in childhood and adolescence. J Child Neurol. 1998. 13:322–326.


49. Evers S, Bauer B, Suhr B, Husstedt IW, Grotemeyer KH. Cognitive processing in primary headache: a study on event-related potentials. Neurology. 1997. 48:108–113.


50. Demarquay G, Caclin A, Brudon F, Fischer C, Morlet D. Exacerbated attention orienting to auditory stimulation in migraine patients. Clin Neurophysiol. 2011. 122:1755–1763.


51. Zohsel K, Hohmeister J, Flor H, Hermann C. Altered pain processing in children with migraine: an evoked potential study. Eur J Pain. 2008. 12:1090–1101.


52. Chen W, Shen X, Liu X, Luo B, Liu Y, Yu R, et al. Passive paradigm single-tone elicited ERPs in tension-type headaches and migraine. Cephalalgia. 2007. 27:139–144.


53. Gerber WD, Stephani U, Kirsch E, Kropp P, Siniatchkin M. Slow cortical potentials in migraine families are associated with psychosocial factors. J Psychosom Res. 2002. 52:215–222.


54. Buodo G, Palomba D, Sarlo M, Naccarella C, Battistella PA. Auditory event-related potentials and reaction times in migraine children. Cephalalgia. 2004. 24:554–563.

