Abstract
Background and Purpose
Cerebral venous flow obstruction (CVFO) is a fatal complication of traumatic brain injury. To compare the outcomes of patients with CVFO secondary to traumatic-brain-injury-induced transsinus fracture who were diagnosed early versus those diagnosed late in the therapeutic course.
Methods
In total, 403 patients with transsinus fracture were reviewed retrospectively. The patients were divided into an early-diagnosis group (n=338) and a delayed-diagnosis group (n=65). The patients submitted to 2D time-of-flight magnetic resonance venography (2D-TOF MRV) and/or CT venography (CTV), depending upon the findings of intracranial pressure monitoring, in order to identify potentially complicated CVFO. These examinations took place within 3 days of the onset of malignant intracranial hypertension symptoms in the early-diagnosis group, and after an average of 7 days in the delayed-diagnosis group. Once diagnosed, patients received intravenous thrombolytic therapy with low-dose urokinase. Patients with massive transsinus epidural hematoma, depressed fracture, or cerebral hernia were treated surgically to relieve the compression and repair any damage to the venous sinuses.
Results
Cerebral venous flow obstruction was much more severe in the delayed-diagnosis group than in the early-diagnosis group (p<0.001), and hence patients in the former group were given a higher dose of urokinase (p<0.001) for thrombolytic therapy. They were also significantly more likely to need surgery (48.1% vs. 20.6%, p=0.003) and had a higher mortality rate (37.0% vs. 4.1%, p<0.001). However, patients in both groups experienced a similarly favorable prognosis, not only with regard to functional outcome but also with respect to neuroradiological improvement, as evaluated by 2D-TOF MRV/CTV at the final follow-up (p=0.218).
Conclusions
Delayed diagnosis can result in increased risk of surgery and death in the acute phase. Thrombolytic therapy with low-dose urokinase resulted in promising improvements in both functional and neuroradiological outcomes in all of the patients in this study, regardless of the time to diagnosis.
Cerebral venous flow obstruction (CVFO), which comprises cerebral venous sinus thrombosis (CVST) and nonthrombotic cerebral venous sinus stenosis (CVSS), is an uncommon condition characterized by hemodynamic deterioration in the cerebral venous or dural sinuses, and in rare instances in the cortical veins.1 Decades of effort has successfully reduced the mortality of CVFO, the level of which has varied greatly in previous studies, from 20-78% to 4.39-10%.2-4 This condition is estimated to account for 0.5-1% of all strokes, regardless of the underlying predisposing etiology.2,5 However, its prevalence increases significantly with the accumulation of risk factors, including traumatic brain injury.1,6
Traumatic brain injury can cause CVFO by either extravascular factors such as direct compression or endothelial damage to venous sinuses induced by skull fracture or intracranial hematomas, or by intravascular factors such as hypercoagulable states produced by traumatic blood loss and excessive application of dehydration therapy with mannitol.7,8 The raised clinical awareness of this condition and improvements in neuroradiologic techniques mean that nowadays increasing numbers of CVFOs are diagnosed at a relatively early phase. A previous study found that CVST occurred in 40.7% of patients with blunt head trauma who had skull fractures extending to a dural venous or jugular bulb, and that the CVST was occlusive in 55% of these patients.9 However, with the exception of progressive headache and vomiting due to persistent intracranial hypertension, CVFO lacks typical clinical symptoms, and this means that many patients with CVFO are diagnosed late or even misdiagnosed, and are consequently subjected to unnecessary surgical procedures and prolonged morbidity.
While digital subtraction angiography (DSA) was originally considered the gold standard for the diagnosis of CVFO, the most widely used noninvasive techniques for evaluating the dural sinuses and cerebral veins today are magnetic resonance venography (MRV) techniques.10 These techniques include 2D time-of-flight (TOF) MRV, 3D-TOF MRV, phase-contrast MRV, and phase-sensitive imaging MRV. Since it is easy to perform and does not require administration of a contrast agent, 2D-TOF MRV is commonly used for assessing the cerebral venous sinuses.11 Furthermore, CT venography (CTV) is often used as an adjuvant imaging method to reduce the probability of potential false-positive results that may occur with 2D-TOF MRV and to increase the accuracy of CVFO diagnosis, since some patients have congenitally normal variations in the cerebral venous flow pattern.
Based on the hypothesis that early diagnosis and management of CVFO would result in a more favorable prognosis, repeated 2D-TOF MRV and/or CTV were used in the present study to evaluate traumatic-brain-injury patients with a high risk of CVFO, and the curative effects of systematic thrombolytic therapy with low-dose urokinase were analyzed.
The medical records of 403 patients with traumatic brain injury who had transsinus fracture at sites with a high risk of CVFO (including simple transsinus linear fracture, transsinus fracture and transsinus epidural hematoma, transsinus depressed fracture) were reviewed. All of the patients or their guardians provided written informed consent to participate in this study. The patients were divided into an early-diagnosis group and a delayed-diagnosis group to enable a comparative study. Scores on the Glasgow Coma Scale (GCS), Injury Severity Scale (ISS), and Glasgow Outcome Scale (GOS) were available for all patients. The early-diagnosis group comprised 338 patients who were admitted to our hospital between January 2006 and December 2010, while the delayed-diagnosis group comprised 65 patients who were admitted to our hospital between January 2004 and December 2005. Briefly, patients in the early-diagnosis group underwent daily lumbar puncture for intracranial pressure (ICP) monitoring. The contraindications for lumbar puncture include 1) obvious obstructed circulation of cerebrospinal fluid and 2) brain hernia. In 234 and 30 patients in the early- and delayed-diagnosis groups, respectively, ICP was recorded via lumbar puncture. Plain CT and/or MRI scans were carried out in patients who suffered from deteriorated clinical signs or symptoms or whose ICP persistently increased by 50-100 mmH2O/day or remained at a high level (above 300 mmH2O) for at least 3 days despite conventional dehydration therapy with mannitol (25 g, intravenous, twice per day) in order to preclude aggravated or new cerebral hemorrhages or cerebral edema. If there were no lesions in addition to the primary lesions, potential CVFO was suspected and CTV and/or MRV examinations were performed. After the diagnosis of CVFO, which usually occurred within 3 days after admission, patients were given systematic thrombolytic therapy with low-dose urokinase. Unlike patients in the early-diagnosis group, those in the delayed-diagnosis group did not receive CTV/MRV examinations until there were manifestations of malignant intracranial hypertension, such as impaired consciousness or even coma, which occurred at an average of 7 days after admission. The detailed diagnostic and therapeutic schedules are presented in Fig. 1.
The 2D-TOF MRV was performed using a 3.0-T or 1.5-T superconductive magnetic resonance scanner with a standard head coil (NOVUS, SIEMENS, Erlangen, Germany). Scanning was conducted twice, bilaterally at 15°.11,12 Some patients underwent repeated 2D-TOF MRV and CTV to reduce the false-positive rate associated with 2D-TOF MRV. Patients were excluded if 1) they developed central respiratory or circulatory failure due to a severe disease condition, 2) they had unstable vital signs, 3) they were unable or refused to submit to 2D-TOF MRV, 4) they had no symptoms of high ICP including headache and vomiting, 5) an additional CTV examination revealed a patent sinus, or 6) they did not have increased ICP or symptoms despite having a thin transverse sinus on one side.
Cerebral venous flow obstruction was diagnosed if MRV revealed local stenosis of the venous sinuses (local filling defect, sudden thinning, and visualization of the distal end), thinning of a venous sinus to less than half of the normal diameter of the distal end in the same venous sinus (thinning observed over a long distance), or nonvisualization of the venous sinuses at the site of transsinus fracture or transsinus hematoma.
All patients diagnosed with CVFO were treated with urokinase, which was administered either within 48 hours after admission or 24 hours after surgery. The dosage of urokinase ranged from 20000 to 50000 U/day for children and from 100000 to 400000 U/day for adults. Adult patients were also given antiplatelet aggregation treatment with oral aspirin (100 mg once daily in the evening) for 1-3 months after urokinase treatment was discontinued. For patients with a severe disease condition [ICP >400 mmH2O (29.4 mm Hg) or nonvisualization of the venous sinuses on 2D-TOF MRV, particularly with regard to the superior sagittal sinus], and especially those with depressed fracture, low-molecular-weight heparin calcium (6000 U, subcutaneous, twice daily) was given due to damage to the endothelial cells of the venous sinuses. Clotting time and prothrombin time were closely monitored during the treatment. The dose and duration of urokinase were adjusted dynamically with reference to improvement in clinical symptoms and reduction of ICP (measured by lumbar puncture). The patients were monitored intensively during hospitalization. Their response to urokinase therapy was checked on days 10-15 after the initial dose, and then again on day 45. After discharge from hospital, they were usually followed up monthly for at least 3 months.
For patients with transsinus depressed fracture, especially with a depression depth of >0.5 cm, urgent surgical intervention was performed to relieve the compression and repair any damage to the venous sinuses. Patients with mild hematoma did not require specific treatment. Patients with severe hematoma (hemorrhage volume of about 15 mL) could be treated with twist-drill drainage followed by injection of urokinase for clot lysis; if the hemorrhage volume was >20 mL and the patient's clinical signs or symptoms persistently deteriorated, hematoma evacuation was performed by decompressive surgery.
Data were analyzed using SPSS 15.0 statistics software (SPSS Inc., Chicago, IL, USA). Continuous data are presented as mean±SD values, and categorical data as n (%) values. The two-sample t-test was performed to compare differences between groups for continuous data, and the Pearson chi-square test was performed to compare difference between groups for categorical data. Fisher's exact test was also considered if there were fewer than five cells. Statistical assessments were two-tailed and considered significant at p<0.05.
The demographics and clinical characteristics of the 403 patients enrolled in this study are presented in Table 1. There were no significant differences between the early-diagnosis (n=338) and delayed-diagnosis (n=65) groups with respect to mean age (34.8±16.7 years vs. 37.2±12.8 years, p=0.273), gender (male/female, 251/87 vs. 42/23; p=0.148), types of transsinus fracture (transsinus linear fracture, 203 vs. 36 cases; transsinus depressed fracture, 110 vs. 20; and transsinus fracture and epidural hematoma, 25 vs. 9; p=0.230), baseline evaluation scales on admission (GCS: 12.5±2.3 vs. 13.0±1.7, p=0.096; ISS: 18.5±10.3 vs. 16.8±9.3, p=0.266; and GOS: 4.3±0.4 vs. 4.2±0.8, p=0.130), or ICP (220.4±78.2 vs. 228.2±65.2 mmH2O, p=0.352). However, at the time of CVFO diagnosis, GCS score (12.3±2.6 vs. 9.7±3.4, p<0.001) and ICP (236.6±89.8 vs. 355.0±91.5 mmH2O, p<0.001) were significantly worse in the delayed-diagnosis group than in the early-diagnosis group.
After MRV/CTV examinations, a diagnosis of CVFO was made in 194 early-diagnosis patients and 27 delayed-diagnosis patients. In addition, the incidence of CVFO was correlated with the type of skull fracture (χ2=63.364, contingency coefficient C=0.369, p<0.001). Regardless of the grouping of the study population, CVFO was found in 92 patients (38.5%) with transsinus linear fracture alone, 102 (78.5%) with transsinus depressed fracture, and 27 (79.4%) with transsinus fracture and epidural hematoma. Although the incidence of CVFO was markedly lower in the delayed-diagnosis group than in the early-diagnosis group (41.5%, 27/65 vs. 57.4%, 194/338; p=0.027), the extent of the obstruction was much more serious in the former (local stenosis of venous sinuses, 18.5% vs. 43.3%; thinning of venous sinuses, 29.6% vs. 46.3%; and no visualization of venous sinuses, 51.9% vs. 10.3%; p<0.001). MRV/CTV visualization of the venous sinuses, stenosis of which was the most serious condition, was not possible in about half of the patients with CVFO. In addition, there was no significant difference between the study groups with regard to the extent of the obstruction in the right transverse sinus (p=0.054) or the presence of a sigmoid sinus or torcular Herophili (p=0.792) (Table 2). However, the ratio of the most serious CVFO characterized by nonvisualization of venous sinuses seemed to be much higher for the superior sagittal sinus (8/13 vs. 5/28, p=0.019) and the left transverse sinus or sigmoid sinus (2/4 vs. 6/101, p=0.005) in the delayed-diagnosis group than in the early-diagnosis group. Moreover, the results revealed a statistical correlation between the risk of requiring surgery and the extent of venous sinus obstruction in the delayed-diagnosis group (χ2=16.629, contingency coefficient C=0.617, p<0.001) but not in the early-diagnosis group (χ2=5.161, contingency coefficient C=0.161, p=0.076).
Cerebral venous flow obstruction patients in the delayed-diagnosis group were given a greater dose of urokinase (265.4±85.5×104 U vs. 138.8±105.7×104 U, p<0.001) for thrombolytic therapy, were significantly more likely to require surgery (13/27, 48.1% vs. 40/194, 20.6%; p=0.003), and had a higher mortality (7/65, 10.8% vs. 8/338, 2.4%; p=0.001) compared with those in the early-diagnosis group. The durations of treatment were 11.0±4.2 days (range: 2-28 days) and 16.0±5.6 days (range: 7-25 days) in the early and delayed-diagnosis groups, respectively (p<0.001), and the urokinase dosages were 12.0±5.5×104 U/day (range: 2-40×104 U/day) and 15.6±7.2×104 U/day (range: 5-40×104 U/day), respectively (p=0.002) (Table 1).
After the diagnosis of CVFO and subsequent thrombolytic therapy with low-dose urokinase, most patients in both study groups exhibited both functional recovery and neuroradiological improvement. Urokinase thrombolysis was well tolerated. Eighteen patients died, the majority as a result of brain hernia (12 cases) and the rest from a massive cerebral infarction (6 cases). The overall mortality rates were 2.4% (8/338) and 10.8% (7/65) in the early and delayed-diagnosis groups, respectively (p=0.001) (Table 1). All of the surviving patients in both of the study groups achieved a satisfactory recovery, as demonstrated by significantly resolved clinical symptoms, normalized ICP, and increased GCS and GOS scores (Table 3).
The early-diagnosis group included 74 patients with CVFO who were followed up with MRV prior to discharge. Nineteen patients were followed up twice with MRV (once prior to discharge and then again 1 month thereafter), and nine were followed up three times with MRV (prior to discharge, and then 1 and 3 months thereafter). In the delayed-diagnosis group, 15 patients were followed up adequately at the time of discharge, and 7 patients were followed up for as long as 3 months after discharge. The main reason for losing patients to follow-up was lack of motivation to visit the clinic for a follow-up assessment due to improvement of clinical symptoms. Furthermore, some of the patients lived some distance from our hospital and so chose to be rechecked in their local hospital. The results of the MRV examination at the time of discharge are given in Table 3. Improvement in CVFO was observed in more than 80% of the patients in both study groups. Characteristic neuroradiological images obtained from three patients who suffered from severe CVFO resulting from transsinus linear fracture, transsinus depressed facture, and transsinus epidural hematoma are shown in Figs. 2, 3, 4.
The clinical significance of early diagnosis and management for CVFO for patients with traumatic brain injury and complicated transsinus fractures was investigated by comparing the effects of therapy on two groups of patients with different chronological diagnosis schedules. The results suggest that the CVFO was much more severe (p<0.001) in the early-diagnosis group than in the delayed-diagnosis group, and as a result the latter patients were given a greater dose of urokinase for thrombolytic therapy. Delayed diagnosis resulted in a significant increase in the risk of requiring surgery and a higher mortality. However, after thrombolytic therapy with a low dose of urokinase, patients in both groups had a favorable prognosis not only with respect to functional outcome, but also for neuroradiological outcome (as evaluated by MRV/CTV examination at their final follow-up).
Cerebral venous flow obstruction is commonly characterized by interruption of blood flow signals, filling defects, or irregular stenosis.7 These neuroradiological abnormalities can be determined by DSA, which is the current gold-standard method of detecting the circulation status of the cerebral arteries and veins. DSA imaging may reveal stenosis of the intracranial venous sinuses, slight or no visualization, thickening or dilation of the cortical veins, and a prolonged circulation time in arteries and veins.13 However, because it is an invasive technique and requires the patient to submit to local anesthesia, newer neuroradiological techniques such as MRV and CTV are increasingly being used for the diagnosis of CVST and CVSS.14-16 DSA is also more time-consuming than 2D-TOF MRV and so is not suitable for prompt management if the disease condition changes. A recent study compared time-resolved imaging of contrast kinetics MRV with 2D-TOF MRV and 3D contrast-enhanced MRV to assess which technique was better for visualizing normal cerebral veins and dural venous sinuses.10 It was found that time-resolved imaging of contrast kinetics MRV was comparable to 3D contrast-enhanced MRV and provided better visualization than 2D-TOF MRV. Therefore, although one advantage of 2D-TOF MRV is that it does not require contrast enhancement, thus reducing the risk of unexpected situations in patients with severe trauma, a disadvantage is that it does not provide optimal visualization of veins and venous sinuses. In the present study, 2D-TOF MRV revealed interruption of blood flow signals in venous sinuses and at the narrowed sites, which are usually found at the sites of fracture or hematoma. This suggests that a thrombus is more likely to form at the site subjected to the highest external force, which is directly related to the damage to the endothelial cells of the venous sinuses. In addition, in depressed fracture, the fractured bone may press against or even pierce into the brain tissue, which together with pressure from epidural hematoma comprise the risk factors for CVFO. For patients with these conditions, early diagnosis with MRV is essential. Once CVFO is present, the depressed bones should be repositioned as soon as possible and the epidural hematoma should be removed so as to relieve the mechanical pressure on the cerebral venous sinuses.
Furthermore, congenital variations in the venous sinuses, such as asymmetric lumen and interruption of blood flow, occur in 24% of the normal population, with 90% of them found in the transverse sinuses of the nondominant hemisphere.17 Given that an asymmetric lumen and interruption of blood flow are features used in the diagnosis of CVFO following traumatic brain injury, the diagnostic criteria for CVFO should be well designed so that there is sufficient capability to simultaneously distinguish between congenital variations of the venous sinuses and potential CVFO. In the present study, CTV was performed in addition to MRV in order to decrease the likelihood of false-positive results and increase the accuracy of the diagnosis of CVFO. In our opinion, the goal of treatment for traumatic brain injury is to help patients to survive the brain edema stage while avoiding cerebral herniation or other life-threatening complications due to the increased ICP. Relief of venous-sinus obstruction is thus a key step in the comprehensive treatment of traumatic brain injury; it is beneficial for early intervention in CVFO and avoidance of cerebral herniation or other life-threatening complications due to malignant intracranial hypertension following thrombosis progression.
The value of thrombolytic therapy with urokinase for patients with CVFO appears to be controversial.18-20 A systematic review11 found that although safe, there was a lack of evidence that urokinase was beneficial; however, the clinical effect of thrombolytic therapy has been demonstrated in both case reports and case series.20,21 The results of the present study suggest that early diagnosis and early administration of low-dose urokinase and concomitant dehydration (for treating hematoma/hydrocephalus and to reduce the ICP) can effectively halt the malignantly progressing intracranial hyperpressure and improve the microcirculation status. Moreover, low-dose urokinase is extremely unlikely to cause intracranial hemorrhage (prevalence of 1.03% in the present study), demonstrating that urokinase thrombolytic therapy is both well tolerated by patients and safe.
The disease status should be closely monitored during urokinase treatment. Levels of coagulation factors can be measured in a timely manner to avoid the severe consequences of intracranial hemorrhage. For patients requiring depressive craniotomy, fresh plasma and cryoprecipitate may be applied before surgery to counter the effects of urokinase; hemostatics including para-aminomethylbenzoic acid are not recommended because of the risk of thrombosis. After surgery, levels of coagulation factors are measured at 8-hour intervals; urokinase thrombolytic therapy should not be administered until the endogenous coagulation factors return to normal levels. Only two of the patients in this study developed a second intracranial hemorrhage after urokinase treatment. The present findings suggest that urokinase is safe for patients with CVFO only if coagulation function is monitored closely. The dose of urokinase and duration the treatment should be determined on the basis of recovery of clinical symptoms and reduction of ICP (as assessed by lumbar puncture).
The advantages of early diagnosis and early application of low-dose urokinase for treatment of CVFO are 1) avoidance of high-dose urokinase-induced intracranial hemorrhage,12 2) protection from thrombosis-progression-induced severe consequences, 3) improvement in the blood flow in the veins and venous sinuses, and 4) reduction of the pathological increase in ICP. This therapy provides an important method for lowering ICP in the comprehensive treatment of CVFO.
There is a large space available for supratentorial compensation. A parietal transsinus epidural hematoma usually causes displacement of the sinus sagittalis superior and obstruction of venous return, but seldom results in complete obstruction of the venous sinuses. Simple transsinus fracture seldom causes thrombosis of the sinus sagittalis superior.8,22,23 In contrast, only a small space is available for infratentorial compensation, and a transsinus epidural hematoma usually causes thrombosis of the transverse sigmoid sinus. It is difficult to establish a collateral circulation rapidly following thrombosis of the sinus sagittalis, and the venous return usually passes through the potential cortical veins into the transverse sinus or deep drainage veins, often causing a malignant increase in ICP.24 Once a unilateral thrombosis is formed in the transverse sigmoid sinus, compensation may occur via the contralateral sinuses. Therefore, the increase in ICP may be not evident and a malignant increase in ICP is seldom detected. In a middle parieto-occipital fracture, and especially a depressed fracture, thrombosis at the posterior two-thirds of the sinus sagittalis superior and subsequent malignant increase in ICP should be prevented. The recovery of CVFO is usually obtained by 1) recanalization of the venous sinus, 2) enlargement of the caliber of the venous sinuses, 3) establishment of a collateral circulation, and 4) compensation by the contralateral sinuses. In the present study it was also found that the compensation-enhanced venous flow in the contralateral sinuses in patients who suffered from CVFO induced by a unilateral thrombosis of the transverse sigmoid sinus may become low, with accompanying changes in hemodynamics following recanalization of the affected transverse sinus.
Together the present findings indicate that attention should be paid to CVFO following traumatic brain injury, especially in patients with concomitant transsinus epidural hematoma or transsinus depressed fracture. Early diagnosis is needed for timely treatment in individual patients. Prompt surgical intervention to relieve mechanical pressure is recommended. The results further suggest that venous administration of low-dose urokinase is a key strategy for improving the overall prognosis.
Figures and Tables
Fig. 1
Diagnostic and therapeutic flow chart for patients in the early-diagnosis and delayed-diagnosis groups. CVFO: cerebral venous flow obstruction, ICP: intracranial pressure, LP: lumbar puncture, MRV: magnetic resonance venography, UK: urokinase.
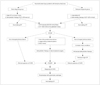
Fig. 2
Urokinase treatment for thrombosis of the left transverse sinus and sigmoid sinus in a traumatic-brain-injury patient. The CT images in the top row show multiple linear fractures in the occipital bone (yellow arrows). The MRI images in the middle row show thrombosis of the left transverse sinus and sigmoid sinus before urokinase treatment (red arrows). The MRV image does not allow visualization of the left transverse sinus, sigmoid sinus, or internal jugular vein; however, a considerable collateral circulation is evident. The MRI images in the bottom row show the disappearance of CVST (red arrows). The MRV image shows satisfactory recanalization of the left transverse sinus, sigmoid sinus, and internal jugular vein, and disappearance of the collateral circulation (blue arrow). CVST: cerebral venous sinus thrombosis, MRV: magnetic resonance venography.
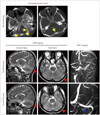
Fig. 3
Urokinase treatment for thrombosis of the superior sagittal sinus in a traumatic-brain-injury patient. The CT images in the upper row show a depressed fracture in the middle of parietal bone before surgical treatment (yellow arrows). MRI images show the compression of a depressed fracture on the superior sagittal sinus before urokinase treatment (red arrows). The MRV image shows nonvisualization of the superior sagittal sinus. The CT images in the lower row show the repositioning of the fracture after surgery. The MRI images show that compression has been relieved (red arrows). The MRV image shows satisfactory recanalization of the superior sagittal sinus after surgery and urokinase treatment (blue arrow). MRV: magnetic resonance venography, UK: urokinase.
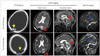
Fig. 4
Recanalization of the right transverse sinus and sigmoid sinus in a patient with transsinus epidural hematoma after urokinase thrombolysis. Images were obtained from a female patient who had suffered a traumatic brain injury 3 hours before admission. Plain CT scans revealed an epidural hematoma crossing the right transverse sinus and sigmoid sinus (A). She received emergent twist-drill drainage for evacuation of an epidural hematoma on admission. Thereafter, the hematoma was gradually evacuated to relieve the mechanical compression (B). However, she still complained of persistent headache and occasional vomiting. A lumbar puncture was performed, which revealed a pathologically high ICP (300 mmH2O) and suggested the presence of CVFO. Subsequent CTV and MRV examinations revealed nonvisualization of the right transverse sinus and sigmoid sinus (C, E). This patient was given thrombolytic therapy with urokinase at a dosage of 100000 U/day. After 3 days of this medication her clinical symptoms were totally alleviated and her ICP had decreased to 115 mmH2O. Subsequent reexamination with CTV and MRV suggested that the involved venous sinuses had recanalized (D, F). CVFO: cerebral venous flow obstruction, CTV: CT venography, ICP: intracranial pressure, MRV: magnetic resonance venography, UK: urokinase.
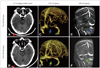
Table 2
Distribution and obstructive extent of cerebral venous flow obstruction in both groups
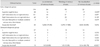
*Significantly different as comparing among venous sinuses (p<0.05), †Significantly different as comparing between early diagnosis group and delayed diagnosis group (p<0.05), ‡Correlation between surgical treatment and obstructive extent of cerebral venous flow obstruction in early diagnosis group, χ2=5.161, p=0.076, contingency coefficient C=0.161, §Correlation between surgical treatment and obstructive extent of cerebral venous flow obstruction in delayed diagnosis group, χ2=16.629, p=0.000, contingency coefficient C=0.617.
References
1. Saadatnia M, Fatehi F, Basiri K, Mousavi SA, Mehr GK. Cerebral venous sinus thrombosis risk factors. Int J Stroke. 2009; 4:111–123.


2. Gosk-Bierska I, Wysokinski W, Brown RD Jr, Karnicki K, Grill D, Wiste H, et al. Cerebral venous sinus thrombosis: incidence of venous thrombosis recurrence and survival. Neurology. 2006; 67:814–819.


3. Borhani Haghighi A, Edgell RC, Cruz-Flores S, Feen E, Piriyawat P, Vora N, et al. Mortality of cerebral venous-sinus thrombosis in a large national sample. Stroke. 2012; 43:262–264.


4. Guenther G, Arauz A. Cerebral venous thrombosis: a diagnostic and treatment update. Neurologia. 2011; 26:488–498.


6. McBane RD 2nd, Tafur A, Wysokinski WE. Acquired and congenital risk factors associated with cerebral venous sinus thrombosis. Thromb Res. 2010; 126:81–87.


7. Elsankari S, Czosnyka M, Lehmann P, Meyer ME, Deramond H, Balédent O. Cerebral blood and CSF flow patterns in patients diagnosed for cerebral venous thrombosis - an observational study. J Clin Imaging Sci. 2012; 2:41.


8. Fuentes S, Metellus P, Levrier O, Adetchessi T, Dufour H, Grisoli F. Depressed skull fracture overlying the superior sagittal sinus causing benign intracranial hypertension. Description of two cases and review of the literature. Br J Neurosurg. 2005; 19:438–442.


9. Delgado Almandoz JE, Kelly HR, Schaefer PW, Lev MH, Gonzalez RG, Romero JM. Prevalence of traumatic dural venous sinus thrombosis in high-risk acute blunt head trauma patients evaluated with multidetector CT venography. Radiology. 2010; 255:570–577.


10. Yiğit H, Turan A, Ergün E, Koşar P, Koşar U. Time-resolved MR angiography of the intracranial venous system: an alternative MR venography technique. Eur Radiol. 2012; 22:980–989.


11. Widjaja E, Shroff M, Blaser S, Laughlin S, Raybaud C. 2D time-of-flight MR venography in neonates: anatomy and pitfalls. AJNR Am J Neuroradiol. 2006; 27:1913–1918.
12. Lewin JS, Masaryk TJ, Smith AS, Ruggieri PM, Ross JS. Time-of-flight intracranial MR venography: evaluation of the sequential oblique section technique. AJNR Am J Neuroradiol. 1994; 15:1657–1664.
13. Qu H, Yang M. Early imaging characteristics of 62 cases of cerebral venous sinus thrombosis. Exp Ther Med. 2013; 5:233–236.


14. Linn J, Pfefferkorn T, Ivanicova K, Müller-Schunk S, Hartz S, Wiesmann M, et al. Noncontrast CT in deep cerebral venous thrombosis and sinus thrombosis: comparison of its diagnostic value for both entities. AJNR Am J Neuroradiol. 2009; 30:728–735.


15. Grosman H, St Louis EL, Gray RR. The role of CT and DSA in cranial sino-venous occlusion. Can Assoc Radiol J. 1987; 38:183–189.
16. Fukusumi A, Okudera T, Takahashi S, Taoka T, Sakamoto M, Nakagawa H, et al. Anatomical evaluation of the dural sinuses in the region of the torcular herophili using three dimensional CT venography. Acad Radiol. 2010; 17:1103–1111.


17. Alper F, Kantarci M, Dane S, Gumustekin K, Onbas O, Durur I. Importance of anatomical asymmetries of transverse sinuses: an MR venographic study. Cerebrovasc Dis. 2004; 18:236–239.


18. D'Alise MD, Fichtel F, Horowitz M. Sagittal sinus thrombosis following minor head injury treated with continuous urokinase infusion. Surg Neurol. 1998; 49:430–435.
19. Novak Z, Coldwell DM, Brega KE. Selective infusion of urokinase and thrombectomy in the treatment of acute cerebral sinus thrombosis. AJNR Am J Neuroradiol. 2000; 21:143–145.
20. Wasay M, Bakshi R, Kojan S, Bobustuc G, Dubey N, Unwin DH. Nonrandomized comparison of local urokinase thrombolysis versus systemic heparin anticoagulation for superior sagittal sinus thrombosis. Stroke. 2001; 32:2310–2317.


21. Nimjee SM, Powers CJ, Kolls BJ, Smith T, Britz GW, Zomorodi AR. Endovascular treatment of venous sinus thrombosis: a case report and review of the literature. J Neurointerv Surg. 2011; 3:30–33.


22. Zhao X, Rizzo A, Malek B, Fakhry S, Watson J. Basilar skull fracture: a risk factor for transverse/sigmoid venous sinus obstruction. J Neurotrauma. 2008; 25:104–111.

