Abstract
Background and Purpose
The vertebral artery (VA) is important for the development of the transverse foramen (TF). Most studies of these structures have focused on anatomical anomalies. Therefore, we investigated quantitatively the association between the relative sizes of the TF and VA.
Methods
We recruited a consecutive series of subjects who underwent CT angiography to estimate the relative sizes of the VA and TF in axial source images. Two neurologists independently reviewed the axial CT images of 208 patients who had no history of transient ischemic attack or stroke. Averaged areas of the VA and TF were defined by the sum of the areas at each level from C3 to C6, divided by 4. Correlation analyses were adjusted for age, sex, and vascular risk factors.
Results
The mean age of the subjects was 53 years. The interobserver and intraobserver reliabilities of TF size were good. There was a linear relationship between the sizes of the VA and TF on each side (right side: r2=0.58, p<0.001; left side: r2=0.62, p<0.001). The area of the VA was significantly associated with that of the TF after adjusting for vascular risk factors.
Conclusions
The size of the VA is strongly and linearly correlated with the size of the TF. These findings suggest that measurement of the TF and VA with CT angiography is a reliable method for evaluating VA diseases, and may provide new insight into the differentiation between VA hypoplasia and atherosclerosis of the VA.
The vertebral artery (VA) usually arises from the subclavian artery and passes through the transverse foramen (TF) from the C6 vertebra to the atlas. After leaving the C1 vertebra, it pierces the dura mater and enters the cranium to form the basilar artery. During development, the VA is formed by anastomosis between the cervical sclerotome segmental arteries, concurrently with the gradual regression of the intersegmental arteries.1
The cranial vasculature plays a crucial role in forming the cranial bony structures.2,3 A unilaterally small TF is associated with hypoplasia or aplasia of the VA, and internal carotid artery (ICA) hypoplasia is usually accompanied by adjacent bony carotid canal narrowing.4 The prevalence of an anomalous VA in patients with failure of sclerotomal segmentation, such as occipitalization of the atlas or Klippel-Feil syndrome, is higher than that in the general population.5,6 Since an intact TF is a prerequisite for the correct development of the VA, evaluating the sizes of the VA and TF could provide helpful information regarding whether a small VA is the result of hypoplasia or acquired narrowing.
Our hypothesis was that the size of the VA is positively correlated with the size of the ipsilateral TF. There has been an increasing understanding of the relationship between the VA and TF, but few studies have examined quantitatively the association between these structures. Therefore, our objective was to determine whether there is a size correlation between the VA and TF.
We retrospectively enrolled asymptomatic patients who underwent neck and brain CT angiography in our hospital. Between January 2007 and March 2010 we enrolled consecutive patients aged 20-65 years who underwent neck and brain CT angiography because of dizziness and headaches that were not associated with any focal neurologic deficits or in order to evaluate unruptured intracranial aneurysms. Demographic and clinical data were collected through the review of medical records. Patients with a history of stroke, transient ischemic attack (TIA), or radiation of the neck were excluded, as were those with moyamoya disease (n=5), Takayasu's arteritis (n=2), Marfan's syndrome or other connective tissue diseases (n=3), cervical spinal cord tumors (n=2), motion artifacts (n=3), or insufficient clinical information (n=5).
This study was approved by the Institutional Review Board at Seoul National University Hospital (IRB No. H-1012-113-345).
CT scanning was performed with a 64-slice multidetector scanner (Brilliance 64, Philips Healthcare, Best, the Netherlands; or Sensation 64, Siemens Medical Solutions, Erlangen, Germany). The sequence parameter settings of the CT scanner were dependent on the CT system options and were as follows: 120 kVp tube voltage, 166-305 mA, 0.6-mm slice thickness, and 200×200 or 240×240 field of view with reconstruction between 1.0 and 1.5. For contrast-enhanced CT imaging an 18-gauge IV catheter was placed into the lesser saphenous vein just above the medial malleolus. Nonionic contrast material (80-100 mL; Ultravist 370, Schering, Berlin, Germany) was injected at a flow rate of 5 mL/s, followed by washing out with 20 mL of normal saline at the same rate. CT images were obtained from the aortic arch to the level of the vertex when the Hounsfield unit number of the ascending aorta was over 100 using the bolus-tracking method.
The axial source images obtained from CT angiography were reviewed. Source images were converted to Digital Imaging and Communications in Medicine format. Axial source images were converted with the window level and width settings of 400 and 4000 HU, respectively, in order to enable more precise visualization of the TF. The cross-sectional areas of each TF and contrast-filled VA were measured with the Medical Image Processing, Analysis, and Visualization program (version 3.0, National Institutes of Health, Bethesda, MD, USA).7 The VA and TF usually had an ovoidal cross-sectional shape, and so we measured the sizes of these structures using the semiautomatic threshold method provided by Medical Image Processing, Analysis, and Visualization program. Two trained neurologists (C. Kim and J.W. Chung) independently measured the sizes of the contrast-filled VA and TF while blinded to the clinical and demographic data. One month later, a neurologist (C. Kim) repeated the measurements with the same parameters in order to establish the test-retest reliability.
Fig. 1 shows a schematic representation of the measuring method for the cross-sectional areas of the VA and TF. The TF is usually cylindrical in shape, with its smallest diameter in its midportion; we therefore measured the size of the TF in the cross-sectional plane. The VA was usually divided into four segments.
We collected demographic and clinical data, including gender, age, and current prescription drugs from the medical records. Subjects were considered hypertensive if they were taking antihypertensive medications, if their systolic blood pressure was ≥140 mm Hg, or if their diastolic blood pressure was ≥90 mm Hg. Subjects were diagnosed with diabetes if they were taking medical treatments for diabetes, if they had a fasting serum glucose level of ≥126 mg/dL, or if they had a non-fasting random serum glucose level of ≥200 mg/dL. Subjects were considered to have hyperlipidemia if they had a fasting total cholesterol level of ≥240 mg/dL or if they were being treated with a lipid-lowering agent. A current smoker was defined as a person smoking one or more cigarettes per day within the last 6 months.
All statistical analyses were performed using the SPSS statistical package for Windows, version 18.0 (SPSS Inc., Chicago, IL, USA). The intra- and interobserver agreements of the measured transverse foraminal areas were calculated using intraclass correlation coefficients (ICCs). The distribution of age was skewed to the left, and was thus transformed with a natural logarithmic scale in order to achieve an approximation of a normal distribution. The Kolmogorov-Smirnov test was used to test for normality. Paired t-tests were used to determine the side-to-side differences in the sizes of the TF and VA. The left VA was usually larger than the right, and so a correlation and regression analysis was performed by splitting the VA into right and left VAs. Pearson's correlation analysis was performed to test whether there was a linear association between the areas of the TF and VA and between the area of the TF and the natural-log-transformed age. Whether the area of the VA is more strongly associated with the ipsilateral than the contralateral area of the TF was assessed with z-statistics using the MedCalc software program (MedCalc Software, Mariakerke, Belgium).
Multiple linear regression analysis was used to assess the correlations between the area of the TF, the area of the VA, the natural-log-transformed age, sex, and vascular risk factors (hypertension, diabetes, hyperlipidemia, and current smoker). The area of the VA was used as a dependent variable. All variables deemed to be significant (p<0.10) using Spearman's correlation analysis were used as independent variables in multiple linear regression analysis with the enter method. The level of statistical significance (two-sided) was set at p<0.05.
In total, 208 patients were included in this study. Table 1 provides the demographic and clinical characteristic for the study population and information on the dominancy of VAs. Although we included only patients without stroke/TIA, 89 (42.8%) had hypertension, 42 (20.2%) had hyperlipidemia, 25 (12.0%) had diabetes, and 46 (22%) were current smokers. The averaged areas of the TF and VA were normally distributed. The inter- and intraobserver ICCs of the measured TF areas were 0.84 (95% CI, 0.772-0.892) and 0.90 (95% CI, 0.859-0.935), respectively. Table 2 gives a side-by-side comparison of VA and TF areas at the different vertebral levels examined. The VAs and TFs were larger on the left than on the right at each level. In addition, the areas of both the VA and TF gradually decreased with increasing level on the vertebral column.
There was a linear relationship between the averaged crosssectional areas of the TF and contrast-filled VA on each side (right side: r2=0.58, p<0.001; left side: r2=0.62, p<0.001) (Fig. 2A and B). However, the association between the natural-log-transformed age and cross-sectional area for the TF was not statistically significant (right side: r2=0.12, p=0.102; left side: r2=0.14, p=0.051; Fig. 2C and D).
Comparative analysis of the correlation coefficient using MedCalc software revealed that areas of the ipsilateral VA were more closely related with those of the ipsilateral TF than those of the contralateral TF (z-statistic: 11.085, p<0.0001 on the right side; z-statistic: 12.205, p<0.0001 on the left side).
A multiple linear regression analysis showed that the cross-sectional area for the contrast-filled VA was associated with the cross-sectional area for the corresponding TF after adjusting for age (natural-log transformed), sex, hypertension, diabetes, hyperlipidemia, and smoking status (Table 3).
The findings of our study show that the size of the TF is a key determinant of that of the VA, irrespective of vascular risk factors. Furthermore, the left TF was larger than the right TF, which is in line with the similar asymmetry of the VAs. We found that measurement of the TF using CT angiography source images is a reliable method with an excellent ICC.
The clinical importance of cranial bony structures was originally identified in patients with ICA hypoplasia, who exhibited a small bony carotid canal on CT images. It is important to be able to discriminate ICA hypoplasia from acquired narrowing of the ICA, because in contrast to patients with atherosclerotic narrowing, patients with ICA hypoplasia usually have a benign course.4,10,11 However, it is difficult to define VA hypoplasia (VAH) because no consensus criteria exist for this condition,12-17 and few studies have investigated the association between the VA and its corresponding TF. Our study shows clearly that the size of the VA is directly proportional to the size of the TF. We should also emphasize that simultaneous evaluation of the VA and TF is important for differentiating between other VA pathologies and VAH, because a small VA with a considerably large TF might represent consistent evidence of diffuse narrowing or acquired shrinkage of the VA.
The incidence of an abnormal entrance to the VA that does not pass through the sixth TF is 5-10%,18,19 and a TF that is filled with the VA is usually larger than an unfilled TF.18 Hypoplasia or atresia of the VA is usually associated with abnormal variations in the TF.2 Our quantitative results support these observations. During early embryogenesis of the aortic arch and great vessels, the intersegmental arteries of the dorsal aorta develop to supply the growing somites.20 Segmental arteries from the first to sixth cervical areas become anastomosed longitudinally with each other, and the seventh segmental artery connects these primitive VAs to the subclavian artery concurrently with the gradual involution of the intersegmental arteries connected to the dorsal aorta.1 Therefore, we suggest that this close developmental relationship between the VA and TF persists into the late adult period.
We identified herein that the areas of the TF and VA gradually decreased with increasing level on the vertebral column, even within a VA V2 segment. The incidence of VAH varies with sex, the population studied, and the diagnostic methods used. Most researchers define VAH as merely a small VA on angiography, and more than half of patients exhibit VA asymmetry. Therefore, older patients or those with concurrent atherosclerotic risk factors are easily diagnosed with VAH. Moreover, defining VAH by measuring the rostral segment of the VA might tend to overestimate the incidence of VAH. Therefore, the TF may be a reliable anatomic landmark for standardizing the measurement of VA size.
Some limitations of the present study should be noted. First, the retrospective observational study design in a single-center tertiary hospital may have resulted in bias when assessing the data. Second, we planned to include asymptomatic patients without vertebrobasilar diseases. However, more than half of the subjects had one or more conventional vascular risk factors. Nevertheless, the relatively young age and the absence of TIA or stroke in our subjects may have strengthened our results. Third, no conventional angiographic data were available to compare the size of the measured variables. Digital subtraction angiography is still accepted as the gold standard for assessing craniocervical vessels. However, it is an invasive procedure in which periprocedural morbidity occurs in up to 4% of cases.21,22 It is inappropriate to use digital subtraction angiography as a diagnostic method in asymptomatic subjects. Finally, our results cannot be generalized directly to other subjects. Acquired erosion of the TF is associated with VA tortuosity,23 neurofibroma, and schwannoma,24,25 as well as acquired connective-tissue diseases.26,27 However, the incidence of VA tortuosity is approximately 3%23 and that of the other conditions is considerably lower.
In summary, the TF area was positively correlated with the corresponding (ipsilateral) VA area. A small VA relative to the TF may be a clue for acquired shrinkage or narrowing of the VA. In addition to conventional cerebral angiography, the VA/TF area ratio may be a valuable diagnostic tool for evaluating VA disease.
Figures and Tables
Fig. 1
Measurements of the transverse foramen and vertebral artery. A: Axial source image of the cervical vertebrae. B: Schematic drawing of the transverse foramen (arrow) and the contrast-filled vertebral artery (curved arrow).
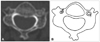
Fig. 2
Scatter plot showing the relationships between the areas of the vertebral artery and transverse foramen, and age (log-transformed). A positive relationship was observed between the areas of the vertebral artery and transverse foramen (A and B), but the association between the log-transformed age and the area of the transverse foramen was not significant (C and D; Pearson's correlation, significant at <0.05). Log age indicates values for log-transformed age.
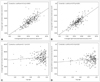
Acknowledgements
This study was supported by a research grant from the Korean Health 21 R&D Project, Ministry of Health and Welfare, Republic of Korea (no. A102065).
References
1. Hähnel S, Hartmann M, Jansen O, Sartor K. Persistent hypoglossal artery: MRI, MRA and digital subtraction angiography. Neuroradiology. 2001. 43:767–769.


2. Vasudeva N, Kumar R. Absence of foramen transversarium in the human atlas vertebra: a case report. Acta Anat (Basel). 1995. 152:230–233.


3. Taitz C, Nathan H, Arensburg B. Anatomical observations of the foramina transversaria. J Neurol Neurosurg Psychiatry. 1978. 41:170–176.


4. Ide C, De Coene B, Mailleux P, Baudrez V, Ossemann M, Trigaux JP. Hypoplasia of the internal carotid artery: a noninvasive diagnosis. Eur Radiol. 2000. 10:1865–1870.


5. Tokuda K, Miyasaka K, Abe H, Abe S, Takei H, Sugimoto S, et al. Anomalous atlantoaxial portions of vertebral and posterior inferior cerebellar arteries. Neuroradiology. 1985. 27:410–413.


6. Yamazaki M, Okawa A, Hashimoto M, Aiba A, Someya Y, Koda M. Abnormal course of the vertebral artery at the craniovertebral junction in patients with Down syndrome visualized by three-dimensional CT angiography. Neuroradiology. 2008. 50:485–490.


7. Bazin PL, Cuzzocreo JL, Yassa MA, Gandler W, McAuliffe MJ, Bassett SS, et al. Volumetric neuroimage analysis extensions for the MIPAV software package. J Neurosci Methods. 2007. 165:111–121.


8. Heary RF, Albert TJ, Ludwig SC, Vaccaro AR, Wolansky LJ, Leddy TP, et al. Surgical anatomy of the vertebral arteries. Spine (Phila Pa 1976). 1996. 21:2074–2080.


9. Gabrielsen TO. Size of vertebral artery and of foramen transversarium of axis An anatomic study. Acta Radiol Diagn (Stockh). 1969. 9:285–291.
10. Kikuchi K, Kowada M, Kojima H. Hypoplasia of the internal carotid artery associated with spasmodic torticollis: the possible role of altered vertebrobasilar haemodynamics. Neuroradiology. 1995. 37:362–364.


11. Caplan LR, Baker R. Extrancranial occlusive vascular disease: does size matter? Stroke. 1980. 11:63–66.


12. Chuang YM, Huang YC, Hu HH, Yang CY. Toward a further elucidation: role of vertebral artery hypoplasia in acute ischemic stroke. Eur Neurol. 2006. 55:193–197.


13. Fisher CM, Gore I, Okabe N, White PD. Atherosclerosis of the carotid and vertebral arteries - Extracranial and intracranial. J Neuropathol Exp Neurol. 1965. 24:455–476.


14. Saito K, Kimura K, Nagatsuka K, Nagano K, Minematsu K, Ueno S, et al. Vertebral artery occlusion in duplex color-coded ultrasonography. Stroke. 2004. 35:1068–1072.


15. Seidel E, Eicke BM, Tettenborn B, Krummenauer F. Reference values for vertebral artery flow volume by duplex sonography in young and elderly adults. Stroke. 1999. 30:2692–2696.


16. Peterson C, Phillips L, Linden A, Hsu W. Vertebral artery hypoplasia: prevalence and reliability of identifying and grading its severity on magnetic resonance imaging scans. J Manipulative Physiol Ther. 2010. 33:207–211.


17. Perren F, Poglia D, Landis T, Sztajzel R. Vertebral artery hypoplasia: a predisposing factor for posterior circulation stroke? Neurology. 2007. 68:65–67.


18. Hong JT, Park DK, Lee MJ, Kim SW, An HS. Anatomical variations of the vertebral artery segment in the lower cervical spine: analysis by three-dimensional computed tomography angiography. Spine (Phila Pa 1976). 2008. 33:2422–2426.


19. Bruneau M, Cornelius JF, Marneffe V, Triffaux M, George B. Anatomical variations of the V2 segment of the vertebral artery. Neurosurgery. 2006. 59:ONS20–ONS24. discussion ONS20-ONS24.


20. Sanelli PC, Tong S, Gonzalez RG, Eskey CJ. Normal variation of vertebral artery on CT angiography and its implications for diagnosis of acquired pathology. J Comput Assist Tomogr. 2002. 26:462–470.


21. Bendszus M, Koltzenburg M, Burger R, Warmuth-Metz M, Hofmann E, Solymosi L. Silent embolism in diagnostic cerebral angiography and neurointerventional procedures: a prospective study. Lancet. 1999. 354:1594–1597.


22. Hankey GJ, Warlow CP, Sellar RJ. Cerebral angiographic risk in mild cerebrovascular disease. Stroke. 1990. 21:209–222.


23. Curylo LJ, Mason HC, Bohlman HH, Yoo JU. Tortuous course of the vertebral artery and anterior cervical decompression: a cadaveric and clinical case study. Spine (Phila Pa 1976). 2000. 25:2860–2864.


24. Peyre M, Ozanne A, Bhangoo R, Pereira V, Tadié M, Lasjaunias P, et al. Pseudotumoral presentation of a cervical extracranial vertebral artery aneurysm in neurofibromatosis type 1: case report. Neurosurgery. 2007. 61:E658. discussion E658.
25. Yünten N, Alper H, Calli C, Selcuki D, Ustün EE. Cervical osseous changes associated with vertebral artery tortuosity. J Neuroradiol. 1998. 25:136–139.