Abstract
Background and Purpose
Differences in hippocampal volume (HV) were compared between chronic primary insomniacs (PIs) and good sleepers (GSs), and the relationship between HV and memory function in PIs was investigated to clarify the effect of chronic sleep deprivation on brain structure and cognition.
Methods
Twenty PIs (mean age, 50 years; 18 females) and 20 age-, gender-, and education-matched GSs were enrolled. Brain magnetic resonance imaging (MRI) was performed on a 1.5-T MRI scanner. Left and right HV and intracranial volume (ICV) were measured manually. Nighttime polysomnography and neuropsychological testing were also applied to all subjects. Group differences in HV were analyzed and the relationships between HV and sleep questionnaire data, nighttime polysomnography, and neuropsychological findings were evaluated.
Results
Compared to GSs, PIs exhibited significantly increased sleep latency and arousal index and a decreased percentage of REM sleep in nighttime polysomnography, as well as impaired verbal and visual memory, and frontal lobe function. Absolute HV and ICV did not differ significantly between PIs and GSs. In the PIs, right and left HVs were negatively correlated with the duration of insomnia and the arousal index, and positively correlated with the recognition of visual memory. In addition, free recall in verbal memory was positively correlated with left HV in PIs.
Primary insomniacs (PIs) have a chronic clinical condition that is characterized by the subjective experience of chronically disturbed sleep and sleep loss, and they show evidence of conditioned sleep difficulties and/or heightened arousal in bed.1 Although the long-term consequences of hyperarousal in PIs has not been fully evaluated, animal studies have shown that persistent exposure to stress and adrenal steroids may result in the degeneration or inhibition of neurogenesis in the hippocampus.2,3
There have been several controversial findings concerning hippocampal volume (HV) in human insomnia studies. A previous pilot study found that the bilateral HV was significantly lower in PIs than in good sleepers (GSs).4 In contrast, a recent study did not find any objective differences in the HVs of PIs, even though some patients with sleep maintenance problems were shown to have smaller HVs, as determined by wrist actigraphy.5
It has been well documented that prolonged sleep deprivation has a negative influence on cognition, even in normal sleepers.6 Experimental sleep curtailment can result in a deterioration of neurocognitive performance by disrupting the nocturnal consolidation of hippocampal-dependent memories, and previous studies have demonstrated that considerable sleep loss worsens structural brain plasticity by attenuating neurogenesis in the hippocampus.7,8 Moreover, it has been demonstrated that cognitive dysfunction occurs in PIs.9 This might be related to altered hippocampal function or structure, since the hippocampus is critically involved in cognitive performance and memory formation.10 However, the relationship between cognitive dysfunction and reduction of HV in PIs has not been elucidated.
The aims of the present study were 1) to compare HV and cognitive function between PIs and age-, gender-, and education-matched GSs, and 2) to determine whether HV is associated with clinical profiles and memory scores in PIs.
Twenty-five patients who complained of sleep onset and/or maintenance insomnia or unrefreshing sleep were enrolled between October 2009 and June 2010. They were diagnosed with PI according to the following inclusion criteria: 1) aged 20-65 years, 2) satisfying the definition of PI according to the International Classification of Sleep Disorders revision 2, and 3) an insomnia duration of ≥1 year. Thirty-one age-, gender-, and education status-matched healthy volunteers who were GSs were recruited through an advertisement in a local community.
The history of medical and sleep disorders was evaluated by clinical interview, and the history of psychiatric disorders was evaluated with the Structured Clinical Interview for the Diagnostic and Statistical Manual of Mental Disorders, Fourth Edition in all PIs and healthy GSs. The subjects completed a sleep diary for at least 2 weeks, along with the following self-completed questionnaires: the Pittsburgh Sleep Quality Index (PSQI), the Beck Depression Inventory (BDI), the Insomnia Severity Index (ISI), the Epworth Sleepiness Scale (ESS), and the Stanford Sleepiness Scale. In addition, PIs and healthy GSs underwent a physical examination, and laboratory testing to measure several factors, including electrolytes, complete blood counts, urine toxic screening tests for barbiturates, benzodiazepine, methamphetamine, cannabinoids, cocaine, and opiates, liver, thyroid, and renal function tests, nighttime polysomnography (PSG), and neuropsychological tests. GSs with obstructive sleep apnea syndrome (apnea-hypopnea index >5/hour) or periodic limb movement disorders were excluded from the study.
The general criteria for excluding PIs and GSs included the following parameters: 1) abnormal sleep-wake rhythms, 2) other sleep disorders, 3) hypertension, diabetes, heart, or respiratory diseases, 4) history of cerebrovascular disease, 5) other neurological (neurodegenerative diseases, epilepsy, or head injury) or psychiatric (psychosis or current depression) diseases, 6) alcohol or illicit drug abuse, or current intake of psychoactive medications, and 7) a structural lesion on brain magnetic resonance imaging (MRI). Volunteers with a mean daily sleep duration of <7 hours were also excluded from the GS group. To exclude the possible effect of hypnotics on cognition, these drugs were prohibited for at least 1 month before the study.
Six patients whose brain MRI revealed diffuse brain atrophy or lacunar infarctions were excluded from the study. Finally, 20 PI patients and 20 GSs were included as the study cohort. Informed consent was obtained from all PIs and GSs for PSG and brain MRI, and the institutional review board of our hospital authorized the study protocol.
Subjects were asked not to drink alcohol or caffeinated beverages on the day before the sleep studies. Sleep data were recorded using a Remlogic (Embla Systems, Denver, CO, USA). Nighttime PSG was performed using a four-channel electroencephalogram (C3/A2, C4/A1, O1/A2, and O2/A1), a four-channel electrooculogram, an electromyogram of the submental, intercostal, and anterior tibialis muscles, and an electrocardiogram with surface electrodes. A thermistor (for monitoring nasal airflow), a nasal air pressure monitor, an oximeter (for measuring oxygen saturation), piezoelectric bands (for determining thoracic and abdominal wall motions), and a body position sensor were attached to all patients. Subjects were recorded on videotape using an infrared video camera and were monitored continuously by a PSG technician. Sleep architecture was scored in 30-s epochs, and sleep staging was interpreted according to the standard criteria of Rechtschaffen and Kales.11 Apnea and hypopnea episodes were defined by previously established criteria. Obstructive apnea was defined as a reduction in airflow greater than 90% that lasted at least 10 s, during which there was evidence of a persistent respiratory effort. Central apnea was defined as a reduction in airflow of more than 90% that lasted at least 10 s, during which there was no evidence of respiratory effort. Hypopnea was defined as a reduction in airflow of 30% for more than 10 s that was accompanied by oxygen desaturation of at least 4%. According to the American Sleep Disorders Association Task Force criteria, arousals were classified as breathing-related arousals (occurring within 3 s following apnea, hypopnea, or snoring) and other types of arousal (spontaneous arousal or arousal associated with periodic limb movements).
MRI scanning was performed with a GE Signa 1.5-tesla scanner (GE Medical Systems, Milwaukee, WI, USA). Fluid-attenuated inversion recovery MRI was performed with the following parameters: 1.0-mm gap, 4.0-mm thickness, repetition time/echo time (TR/TE)=10002/127.5 ms, number of excitations (NEX)=1, and oblique coronal. T1-weighted and T2-weighted MRI was performed with the following parameters: 0.3-mm gap, 3.0-mm thickness, TR/TE=5300/99 ms, flip angle (FA)=95°, NEX=3, and oblique coronal. Coronal spoiled gradient recalled (SPGR) MRI was performed with the following scanning variables: 1.6-mm thickness, no gap, 124 slices, TR/TE=30/7 ms, FA=45°, NEX=1, matrix size=256×192, and field of view=22×22 cm. The voxel dimensions in the SPGR MRI images were 0.86×0.86×1.6 mm.
Preprocessing of T1-weighted MRI data and manual volume measurements for intracranial volume (ICV) and the hippocampus were performed using a Unix-based Sun Ultra 1 Creator workstation (Sun Microsystems, Santa Clara, CA, USA) and Analyze 7.5 (Biomedical Imaging Resource, Mayo Foundation, Rochester, MN, USA). ICV was used to normalize HV and to correct for variations in individual brain sizes.12 The ICV was measured by reconstructing the original T1-weighted MRI data in order to create 5-mm-thick sagittal images, which were then magnified twofold. The cerebrum, cerebellum, and midbrain were included in the ICV volume with the outer boundary of dura mater.13-15 The lateral limits of the ICV were defined as the right- and leftmost slices of the brain parenchyma on sagittal images, and the lower tip of the cerebellum was defined as the lower limit. We increased the brightness of the image in order to improve the visual clarity of the boundary of the dura mater. Using the established measurement criteria,15 the dura mater of the cerebrum, the cerebellum, and the midbrain (except for the inferior boundary) were traced manually. In order to establish the inferior boundary on the head tilt-corrected sagittal images, a horizontal line that included the lower tip of the cerebellum was drawn across the midbrain.14 The ICV measurements have been illustrated previously.16
The methodological details of hippocampal volumetry are described elsewhere.17 The entire HV was measured from the anterior head to the posterior tail, including the cornu ammonis, gyrus dentatus, hippocampus, and subiculum. The anterior boundary of the hippocampus was identified as the alveus. The lateral border of the hippocampus was delineated against the entorhinal cortex by the upper margin of the white matter of the subiculum. The posterior end of the hippocampus was taken as the point at which the tail of the hippocampus disappeared. The rater manually traced the alveus according to the defined hippocampal boundary criteria.
The hippocampus was traced manually by one neuroimaging analyst (ST Kim) who was blind to the patient diagnosis. The HV in each slice was calculated by multiplying the number of voxels contained within each trace by the voxel volume (1 mm3) and dividing by the magnification factor. The total volume of each structure was the sum of all in-slice volumes. In order to calculate the intrarater correlation coefficients, the brain images of each subject were manually traced twice. The intrarater correlation coefficients were 0.96 and 0.95 for the left and right HVs, respectively, and 0.94 for both ICVs.
A battery of neuropsychological tests and an individual standardized intelligence test were administered to all subjects. The neuropsychological tests, as described below, took 2.5 hours to perform.
Verbal memory was evaluated using the Korean California Verbal Test (K-CVLT).18 Nonverbal (visual) memory was assessed using the Rey Complex Figure Test (RCFT), which comprises an immediate recall test, a delayed recall test, and a recognition test.19 The K-CVLT involves 5 learning steps and 16 words, and immediate recall, immediate hint recall, delayed free recall, delayed hint recall, and recognition were tested 20 min after the 5 learning steps. The RCFT involves immediate recall after copying a complex figure, and free recall and recognition tested 20 min later.
The digit span tests from the Wechsler Memory Scale-Revised were administered using a standard protocol,20 both forward and backward, in order to assess verbal attention and working memory. The Corsi block-tapping tests (forward and backward) were used to examine visual attention and working memory.21 In addition, we administered Trail-Making Tests A and B and the digit symbol test.22,23
The demographic factors (age, education, and body mass index) and the sleep questionnaire data were compared between PIs and GSs using the Mann-Whitney U-test. Group differences in HV were analyzed two ways: 1) by analysis of covariance with the covariate of ICV29-31 and 2) by normalizing the absolute HV relative to ICV.32 In order to evaluate the relationships between HV and the sleep questionnaire data and nighttime PSG, a multilinear regression analysis was performed after controlling for ICV, age, and education. The differences in the neuropsychological results between PIs and GSs were analyzed using multiple regressions with the covariates of age and education.
For the relationship between HV and neuropsychological results, a multiple regression analysis was performed with the covariates of education, age, and ICV. Statistical analysis was performed using SPSS version 18.0 (IBM, Chicago, IL, USA). All tests were two-tailed, and the level of significance was set at p<0.05.
All subjects were right-handed. The mean age, gender ratio, and BMI did not differ between PIs and GSs. The mean duration of insomnia was 7.6 years, and PIs had been taking an average of two kinds of sleeping pill for a mean duration of 3.7 years. The patients had not taken any hypnotic agents, including zolpidem, alprazolam, or flurazepam, for more than 1 month before the study. Sleep quality was determined by the PSQI, which was assessed during the 2 weeks before the study, and was found to be significantly worse in PIs than in GSs. PIs reported severe insomnia symptoms (mean ISI=19.1). The ESS score was higher in PIs than in GSs (p=0.02), but it did not indicate that PIs experienced daytime sleepiness (mean ESS score=2.9). PIs reported significantly more depressed symptoms than did GSs (BDI scores: 13.8 vs. 3.5, p=0.04), and they experienced the general depressive symptoms (10.6 vs. 1.5, p=0.025) but not the somatic symptoms (3.2 vs. 2.0, p=0.510) of the BDI subscale.
None of the PIs had taken antidepressants or had been previously diagnosed with major depressive disorder. According to the self-completed questionnaires, PIs reported a statistically shorter total sleep time, decreased sleep efficiency, and increased wake after sleep onset (WASO) compared to GSs. In nighttime PSG, PIs had a longer sleep latency and more frequent arousals during sleep than did GSs.
PIs had significantly lower scores on tests of attention and frontal lobe function and impaired verbal (recognition) and visual memories (copying and free recall) relative to GSs (Table 3).
The ICV did not differ between the two groups (1506.8±111.1 cm3 in PIs vs. 1487.9±331.5 cm3 in GSs, mean±SD; p=0.110, two-tailed t-test). The absolute volumes of the left and right hippocampi did not differ between PIs and GSs (left: 3000.3±214.7 mm3 vs. 2897.4±390.5 mm3, p=0.265; right: 3265.2±255.1 mm3 vs. 3151.3±295.7 mm3, p=0.185). After controlling for the ICV, the left and right HVs also did not differ between PIs and GSs (left: p=0.499; right: p=0.570).
In PIs, left or right HV was negatively correlated with a longer insomnia duration (left HV: r=-0.872, p<0.001; right HV: r=-0.868, p<0.001) and with a higher arousal index in nighttime PSG (left HV: r=-0.435, p=0.045; right HV: r=-0.409, p=0.026) (Fig. 1).
A multiple linear regression analysis showed that HV was negatively correlated with the duration of insomnia (left HV: β=-0.669, p<0.001; right HV: β=-0.545, p=0.001) and the arousal index (left HV: β=-0.296, p=0.028; right HV: β=-0.431, p=0.015). A positive correlation was found between short-delay free recall in the K-CVLT and the left HV (r=0.759, p=0.029) and between recognition in the RCFT and the left (r=0.969, p=0.006) and right (r=0.913, p=0.031) HV in the PIs (Table 4).
The GSs exhibited no significant correlations between HV and any of the other parameters, including demographic characteristics, PSG data, or neuropsychological results.
We compared HV and cognitive function between chronic PIs and GSs and investigated the correlation between HV and demographic characteristics and various factors measured from sleep studies and neuropsychological tests.
We found no definite difference in ICV and in absolute and ICV-normalized HV between PIs and GSs. However, the duration of insomnia and the arousal index in nighttime PSG exhibited significant negative correlations with HV in PIs.
There have been inconsistent reports on HV changes in PIs compared with GSs. One previous pilot study found that HV was significantly smaller in PIs than in GSs,4 but a subsequent cross-sectional study did not.5 Several factors could have contributed to this discrepancy. First, the studies used different anatomical landmarks to delineate the boundary of the hippocampus. Riemann et al.4 included the fimbra, the alveus, and the hippocampus-amygdala transition area (HATA) when determining HV, but these regions were not included in the work of Winkelman et al.5 The present study included the alveus but excluded the fimbra and the HATA because the fimbra is regarded as white matter and the HATA is not easy to delineate consistently between subjects.
Second, the different subsets of patients examined in the studies may also play a role. The mean duration of insomnia was longer of the study by Reiman et al. (11.6 years) than in our study (7.6 years) and that of Winkelman et al.5 A longer duration of insomnia might negatively influence hippocampal function and HV. The finding of no difference in HVs between PIs and GSs in the present study was similar to the findings of Winkelman et al.5 However, our patients reported much more severe insomnia symptoms and a longer duration of disease than did those of Winkelman et al.,5 which is attributable to our study involving physician-referred patients with chronic insomnia while the other study selecting patients from community recruits who complained of insomnia symptoms. The mean PSQI (19.1 vs. 12.1) and ISI (19.1 vs. 16.7) seemed to be higher in the present study than in that of Winkelman et al. We observed that left and right HVs in PIs were significantly and negatively correlated with the duration of insomnia, a finding that was not reported by Winkelman et al.5
Winkelman et al.5 reported that smaller HVs were associated with a greater amount of WASO and a reduced sleep efficiency, as measured by actigraphy. We found that a higher arousal index-which was measured in nighttime PSG-was related to a smaller HV. These findings suggest that sleep quality affects the anatomy of the hippocampus even if the absolute HV did not differ from that of normal controls. It has been shown that reduced sleep efficiency is correlated with higher cortisol levels in the evening and nighttime periods.33 Furthermore, patients with Cushing's disease have been reported to have hippocampal atrophy, with the degree of atrophy being correlated with the mean cortisol level.34 Although serum cortical levels were not obtained during nighttime in the present study, our findings of a close relationship between poor sleep quality and smaller HVs in PIs may support a functional impairment of the hypothalamus-pituitary-adrenal gland axis in PIs.
It was well known that HVs are significantly smaller in patients with major depression than in normal controls.35 In the present study, PIs reported more depressive symptoms than GSs (BDI scores: 13.8 vs. 3.5, p=0.04). Although we excluded patients with major depression from the patient group, they might have had dysphoria, which could affect the reduced HVs. However, the BDI score was not correlated with HV in PIs (as assessed by multiple regression analyses).
Cognition-especially for attention and frontal lobe function-was significantly worse in PIs than in GSs in the present study. Task assessments of shifting attention and working memory generally reveal deficits among insomnia groups.36-40 When a task of sustained attention required a response choice, insomniacs exhibit consistent attention deficits.36-39,41,42 Neuroimaging data have revealed a close relationship between insomnia and functional and anatomical damage of various brain regions. A significant association was found between insomnia duration and left dorsomedial prefrontal damage in 192 patients with focal brain lesions.43 The gray-matter volume in the left orbitofrontal cortex was found to be a reduced in chronic insomniacs, with this being strongly correlated with the subjective severity of insomnia.44 The cerebral glucose metabolic rates during wakefulness in the bilateral prefrontal and left superior temporal cortices, the thalamus, the hypothalamus, and the brainstem reticular were found to be lower in chronic insomniacs than in controls.45 These findings may support the impaired attention and frontal lobe function found in PIs compared to GSs in this study.
Another finding in the present study was that both verbal and nonverbal memory functions were significantly worse in PIs than in GSs. It was previously reported that PIs did not have a performance deficit on tasks of visual and verbal memory tests.46 Some researchers have argued that the word-list and word-pair paradigms used in most studies are not very sensitive to subtle memory deficits.47,48 In the present study, the direct comparison of memory scores with age-, gender-, and education level-matched normal controls, and the significantly positive correlation between HV and verbal and visual memory scores may support objective memory deficits in chronic insomniacs.
It is well known that sleep plays an important role in the formation and consolidation of memories49 and that memory consolidation is impaired in patients with chronic insomnia.50 The significantly negative correlation between arousal index and HV in the present study suggests that fragmented sleep is closely related to HV reduction.
A meta-analysis of results from 33 studies had shown widely varying results regarding the relationship between memory performance and HVs in participants without neurological or psychiatric disorders.51 Further study is necessary to reach a consensus regarding the relationship between memory and hippocampal volume in GSs.
Our study was subject to some limitations. PIs have more depressive symptoms than GSs, and their depression or dysphoria may have affected the neuropsychological results.52 Memory deficit in PIs might be directly influenced by impaired attention and frontal lobe function53 rather than via hippocampal dysfunction. We are aware that the sample in this study was too small to allow us to draw a definitive conclusion, but there was a trend toward HV being smaller in PIs than in GSs. Studies with larger samples should evaluate more accurately the effect of insomnia on brain structure.
In summary, we found that HV in PIs was negatively correlated with the duration of insomnia and the arousal index. PIs exhibited significantly impaired attention, frontal lobe function, and memory, and their verbal and nonverbal memory scores were positively correlated with HV. These findings suggest that chronic sleep deprivation associated with insomnia impairs memory and frontal lobe function, and that insomnia duration and poor sleep quality contribute to a bilateral reduction in HV.
Figures and Tables
Fig. 1
Scatter plots of left or right hippocampal volume and duration of insomnia and arousal index in patients with chronic insomnia. Higher values on the arousal index correspond to poor sleep quality. Left or right hippocampal volume was negatively correlated with the insomnia duration (left: r=-0.872, p<0.001; right: r=-0.868, p<0.001) (A) and with the arousal index in nighttime polysomnography (left: r=-0.435, p=0.045; right: r=-0.409, p=0.026) (B).
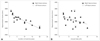
Acknowledgements
This study was supported by a Grant (2010K000817) from Brain Research Center of the 21st Century Frontier Research Program funded by the Ministry of Education, Science, and Technology, and by a Grant of the Korean Health Technology R&D Project, Ministry for Health, Welfare & Family Affairs, Republic of Korea (No. A110097), and by the Global Frontier R&D Program on <Human-centered Interaction for Coexistence> funded by the National Research Foundation of Korea grant funded by the Korean Government (MEST) (NRF-M1AXA 003-2011-0031688).
References
1. Roth T, Roehrs T, Pies R. Insomnia: pathophysiology and implications for treatment. Sleep Med Rev. 2007. 11:71–79.


3. Montaron MF, Drapeau E, Dupret D, Kitchener P, Aurousseau C, Le Moal M, et al. Lifelong corticosterone level determines age-related decline in neurogenesis and memory. Neurobiol Aging. 2006. 27:645–654.


4. Riemann D, Voderholzer U, Spiegelhalder K, Hornyak M, Buysse DJ, Nissen C, et al. Chronic insomnia and MRI-measured hippocampal volumes: a pilot study. Sleep. 2007. 30:955–958.


5. Winkelman JW, Benson KL, Buxton OM, Lyoo IK, Yoon S, O'Connor S, et al. Lack of hippocampal volume differences in primary insomnia and good sleeper controls: an MRI volumetric study at 3 Tesla. Sleep Med. 2010. 11:576–582.


6. Johnson LC, Chernik DA. Sedative-hypnotics and human performance. Psychopharmacology (Berl). 1982. 76:101–113.


7. Guzman-Marin R, Suntsova N, Methippara M, Greiffenstein R, Szymusiak R, McGinty D. Sleep deprivation suppresses neurogenesis in the adult hippocampus of rats. Eur J Neurosci. 2005. 22:2111–2116.


8. Mirescu C, Peters JD, Noiman L, Gould E. Sleep deprivation inhibits adult neurogenesis in the hippocampus by elevating glucocorticoids. Proc Natl Acad Sci U S A. 2006. 103:19170–19175.


9. Fortier-Brochu E, Beaulieu-Bonneau S, Ivers H, Morin CM. Insomnia and daytime cognitive performance: a meta-analysis. Sleep Med Rev. 2012. 16:83–94.


10. Bird CM, Burgess N. The hippocampus and memory: insights from spatial processing. Nat Rev Neurosci. 2008. 9:182–194.


11. Kruggel F. MRI-based volumetry of head compartments: normative values of healthy adults. Neuroimage. 2006. 30:1–11.


12. Lemieux L, Salek-Haddadi A, Krakow K. The nature of MR signal changes. Radiology. 2003. 226:922–923. author reply 923-925.
13. MacLullich AM, Ferguson KJ, Deary IJ, Seckl JR, Starr JM, Wardlaw JM. Intracranial capacity and brain volumes are associated with cognition in healthy elderly men. Neurology. 2002. 59:169–174.


14. Eritaia J, Wood SJ, Stuart GW, Bridle N, Dudgeon P, Maruff P, et al. An optimized method for estimating intracranial volume from magnetic resonance images. Magn Reson Med. 2000. 44:973–977.


15. Chey J, Na DG, Tae WS, Ryoo JW, Hong SB. Medial temporal lobe volume of nondemented elderly individuals with poor cognitive functions. Neurobiol Aging. 2006. 27:1269–1279.


16. Tae WS, Kim SS, Lee KU, Nam EC, Kim KW. Validation of hippocampal volumes measured using a manual method and two automated methods (FreeSurfer and IBASPM) in chronic major depressive disorder. Neuroradiology. 2008. 50:569–581.


17. Kim JK, Kang Y. Normative study of the Korean-California Verbal Learning Test (K-CVLT). Clin Neuropsychol. 1999. 13:365–369.
18. Lu PH, Boone KB, Cozolino L, Mitchell C. Effectiveness of the Rey-Osterrieth Complex Figure Test and the Meyers and Meyers recognition trial in the detection of suspect effort. Clin Neuropsychol. 2003. 17:426–440.


19. Wechsler D. Wechsler Memory Scale--Revised Manual. 1987. San Antonio: The Psychological Corporation, Harcourt Brace Jovanovich.
20. Zola-Morgan S, Squire LR, Amaral DG. Human amnesia and the medial temporal region: enduring memory impairment following a bilateral lesion limited to field CA1 of the hippocampus. J Neurosci. 1986. 6:2950–2967.


21. Reitan RM. Trail Making Test: Manual for Administration and Scoring. 1992. Tucson: Reitan Neuropsychology Laboratory.
22. Lee YH, Song JY. A study of the reliability and the validity of the BDI, SDS, and MMPI-D scales. Korean J Clin Psychol. 1991. 10:98–113.
23. Heaton RK, Chelune GJ, Talley JL, Kay GG, Curtiss G. Wisconsin Card Sorting Test Manual Revised and Expanded. 1993. Odessa, FL: Psychological assessment resources, Inc..
24. Lee JH, Kang YW, Na DL. Efficiencies of stroop interference indexes in healthy older adults and dementia patients. Korean J Clin Psychol. 2000. 19:807–818.
25. Kang YW, Na DL. Seoul Neuropsychological Screening Battery. 2003. Seoul: Haman Brain Research & Consulting.
26. Raven JC. Coloured Progressive Matrices Sets A, Ab, B. Manual Sections 1 & 2. 1995. Oxford: Oxford Psychological Press.
27. Kim HH, Na DL. Korean Boston Naming Test (K-BNT). 1997. Seoul: Hakjisa.
28. Free SL, Bergin PS, Fish DR, Cook MJ, Shorvon SD, Stevens JM. Methods for normalization of hippocampal volumes measured with MR. AJNR Am J Neuroradiol. 1995. 16:637–643.
29. O'Brien LM, Ziegler DA, Deutsch CK, Kennedy DN, Goldstein JM, Seidman LJ, et al. Adjustment for whole brain and cranial size in volumetric brain studies: a review of common adjustment factors and statistical methods. Harv Rev Psychiatry. 2006. 14:141–151.
30. Tae WS, Hong SB, Joo EY, Han SJ, Cho JW, Seo DW, et al. Structural brain abnormalities in juvenile myoclonic epilepsy patients: volumetry and voxel-based morphometry. Korean J Radiol. 2006. 7:162–172.


31. Jack CR Jr, Twomey CK, Zinsmeister AR, Sharbrough FW, Petersen RC, Cascino GD. Anterior temporal lobes and hippocampal formations: normative volumetric measurements from MR images in young adults. Radiology. 1989. 172:549–554.


32. Vgontzas AN, Bixler EO, Lin HM, Prolo P, Mastorakos G, Vela-Bueno A, et al. Chronic insomnia is associated with nyctohemeral activation of the hypothalamic-pituitary-adrenal axis: clinical implications. J Clin Endocrinol Metab. 2001. 86:3787–3794.


33. Brown ES, Rush AJ, McEwen BS. Hippocampal remodeling and damage by corticosteroids: implications for mood disorders. Neuropsychopharmacology. 1999. 21:474–484.


34. Campbell S, Marriott M, Nahmias C, MacQueen GM. Lower hippocampal volume in patients suffering from depression: a meta-analysis. Am J Psychiatry. 2004. 161:598–607.


35. Edinger JD, Means MK, Carney CE, Krystal AD. Psychomotor performance deficits and their relation to prior nights' sleep among individuals with primary insomnia. Sleep. 2008. 31:599–607.


36. Varkevisser M, Kerkhof GA. Chronic insomnia and performance in a 24-h constant routine study. J Sleep Res. 2005. 14:49–59.


37. Altena E, Van Der Werf YD, Strijers RL, Van Someren EJ. Sleep loss affects vigilance: effects of chronic insomnia and sleep therapy. J Sleep Res. 2008. 17:335–343.


38. Hauri PJ. Cognitive deficits in insomnia patients. Acta Neurol Belg. 1997. 97:113–117.
39. Edinger JD, Glenn DM, Bastian LA, Marsh GR. Slow-wave sleep and waking cognitive performance II: Findings among middle-aged adults with and without insomnia complaints. Physiol Behav. 2000. 70:127–134.
40. Sugerman JL, Stern JA, Walsh JK. Daytime alertness in subjective and objective insomnia: some preliminary findings. Biol Psychiatry. 1985. 20:741–750.


41. Schneider-Helmert D. Twenty-four-hour sleep-wake function and personality patterns in chronic insomniacs and healthy controls. Sleep. 1987. 10:452–462.


42. Koenigs M, Holliday J, Solomon J, Grafman J. Left dorsomedial frontal brain damage is associated with insomnia. J Neurosci. 2010. 30:16041–16043.


43. Altena E, Vrenken H, Van Der Werf YD, van den Heuvel OA, Van Someren EJ. Reduced orbitofrontal and parietal gray matter in chronic insomnia: a voxel-based morphometric study. Biol Psychiatry. 2010. 67:182–185.


44. Nofzinger EA, Buysse DJ, Germain A, Price JC, Miewald JM, Kupfer DJ. Functional neuroimaging evidence for hyperarousal in insomnia. Am J Psychiatry. 2004. 161:2126–2128.


45. Shekleton JA, Rogers NL, Rajaratnam SM. Searching for the daytime impairments of primary insomnia. Sleep Med Rev. 2010. 14:47–60.


46. Uttl B. Measurement of individual differences: lessons from memory assessment in research and clinical practice. Psychol Sci. 2005. 16:460–467.
47. Uttl B, Graf P, Richter LK. Verbal Paired Associates tests limits on validity and reliability. Arch Clin Neuropsychol. 2002. 17:567–581.


48. Walker MP, Stickgold R. Sleep-dependent learning and memory consolidation. Neuron. 2004. 44:121–133.


49. Backhaus J, Junghanns K, Born J, Hohaus K, Faasch F, Hohagen F. Impaired declarative memory consolidation during sleep in patients with primary insomnia: Influence of sleep architecture and nocturnal cortisol release. Biol Psychiatry. 2006. 60:1324–1330.


50. Van Petten C. Relationship between hippocampal volume and memory ability in healthy individuals across the lifespan: review and meta-analysis. Neuropsychologia. 2004. 42:1394–1413.

