Abstract
Background and Purpose
To evaluate changes in cortical excitability induced by zonisamide (ZNS) in focal epilepsy patients.
Methods
Twenty-four drug-naїve focal epilepsy patients (15 males; overall mean age 29.8 years) were enrolled. The transcranial magnetic stimulation parameters obtained using two Magstim 200 stimulators were the resting motor threshold, amplitude of the motor-evoked potential (MEP), cortical silent period, short intracortical inhibition, and intracortical facilitation. These five transcranial magnetic stimulation parameters were measured before and after ZNS, and the findings were compared.
Results
All 24 patients were treated with ZNS monotherapy (200-300 mg/day) for 8-12 weeks. After ZNS, MEP amplitudes decreased (-36.9%) significantly in epileptic hemispheres (paired t-test with Bonferroni's correction for multiple comparisons, p<0.05), whereas the mean resting motor threshold, cortical silent period, short intracortical inhibition, and intracortical facilitation were unchanged (p>0.05). ZNS did not affect cortical excitability in nonepileptic hemispheres.
Focal epilepsy is defined by its epileptogenic zone, which is limited to one or more regions of the brain. Various diagnostic tools, including neuroimaging studies, have been used to localize the epileptic focus and understand the pathophysiology of focal epilepsy.1-4 Transcranial magnetic stimulation (TMS) provides a well-established noninvasive means of investigating human motor cortex excitability.5,6 TMS may serve as a marker of excitatory/inhibitory imbalance in the cortical neurons of epilepsy patients because it detects relatively subtle alterations in the physiological state of the brain.7 Published TMS results obtained in epilepsy patients are often conflicting. TMS studies have often been conducted in chronic epilepsy patients taking at least one antiepileptic drug (AED), and AEDs have been shown to affect several TMS parameters. It is therefore often difficult to separate intrinsic cortical excitability changes resulting from the epileptic condition and those associated with drug effects in patients. Furthermore, most TMS studies have been performed on healthy subjects, and the few studies performed in drug-naïve epilepsy patients have produced disparate results.8-10 Nevertheless, a recent study on a large number of drug-naïve patients with new-onset epilepsy revealed hyperexcitability in brains affected by idiopathic generalized epilepsy (IGE) or focal epilepsy.11
Zonisamide (ZNS) is an AED with proven efficacy as an adjunctive therapeutic agent in patients with partial seizures.12-15 ZNS is generally well tolerated and has a favorable pharmacokinetic profile that permits once- or twice-daily administrations. ZNS appears to block the spread of seizure discharges and to suppress the epileptic focus, although the precise mechanism of its antiepileptic activity is unknown.16 Based on the findings of cellular and animal studies, the suggested antiepileptic mechanisms of ZNS are as follows:16,17
1) Membrane stabilization (blockade of voltage-gated sodium channels, inhibition of T-type voltage-gated calcium channels, and free-radical scavenging).
2) Neuromodulation (facilitation of dopaminergic and serotonergic transmission, and blockade of potassium-evoked glutamate-mediated excitatory synaptic transmission).
3) Neuroprotection (free-radical scavenging).
A TMS study of the effects of ZNS on cortical excitability was performed in patients with generalized epilepsy.18 After ZNS treatment, the amplitudes of motor-evoked potentials (MEPs) were significantly reduced in both hemispheres (right, -34.2%; left, -37.0%), whereas other TMS parameters were not significantly changed.
The aim of the present study was to determine the effect of ZNS monotherapy on cortical excitability in drug-naïve patients diagnosed with focal epilepsy.
We enrolled consecutive focal epilepsy patients who had not previously taken an AED. The diagnostic criteria of the International League Against Epilepsy (1989) were followed throughout. The following patient inclusion criteria were applied:
1) Clinically documented complex focal seizures or secondarily generalized tonic-clonic seizures (SGTCS) with a lateralizing sign such as unilateral somatosensory/visual aura, dystonic posturing, or version.
2) Experience of more than two spontaneous epileptic seizures.
3) Only one type of clinical seizure on history or on video-electroencephalogram (EEG) monitoring.
4) Satisfactory localization of the epileptogenic area by interictal or ictal epileptiform discharges on video-EEG monitoring during a typical seizure (including epileptic aura).
5) No multifocal or independent right- or left-hemisphere interictal epileptiform discharges on EEG.
6) No history of antiepileptic medication.
7) No lesion in the motor cortex on brain magnetic resonance imaging (MRI). If there no MRI abnormality concordant with the location of interictal spikes was found, a long-term video-EEG monitoring or 18F-fluorodeoxyglucose-positron-emission tomography was performed.
Patients with a history of head trauma, another neurological disease, or psychiatric disorders were excluded. Applying these criteria resulted in the inclusion of 24 patients in the study (aged 29.8±6.5 years, mean±SD; epileptic focus: left in 13, right in 11; temporal in 18, frontal in 2, parietal in 2, and occipital in 1). These and other patient characteristics are given in Table 1.
Twenty-seven patients underwent a TMS study before ZNS. During the 12-week period of ZNS therapy, two patients dropped out (they arbitrarily discontinue medication) and one patient changed to another AED due to ZNS side effects. The remaining 24 patients became seizure free after 6 weeks of ZNS administration. Finally, 24 patients (15 males, 9 females) who were treated with ZNS for 8-9 weeks underwent TMS study after ZNS.
All 24 patients received ZNS as a monotherapy for 8-12 weeks. Steady-state concentrations of ZNS were achieved within 14 days.19 Before ZNS administration, all patients underwent a physical examination, routine blood test (hematology and chemistry panel including liver function tests), and brain MRI. The predetermined target dosage for ZNS was 200 mg/day, although this depended on the individual. Our intention was to administer ZNS at 100 mg/day for the first 2 weeks, and then 200 mg/day for the next 6 weeks. However, if seizures occurred in patients during the latter 6-week period, ZNS dosages were further increased by 25 mg/day for a week in order to achieve a seizure-free state for at least 2 weeks prior to the post-ZNS TMS study.
Moreover, when an intolerable adverse event was encountered due to ZNS administration, the dosage was decreased by 25 mg/day for 1 week until the symptoms subsided. During the study period, a physician examined patients fortnightly; blood testing for hematology and liver function was performed twice before medication and again at the end of the study period. Physicians documented seizure frequency in detail in a seizure diary when patients visited the outpatient clinic.
MEPs were recorded using surface electromyography (EMG) electrodes placed over the first dorsal interosseus (FDI) muscle in a belly-tendon montage. Raw EMG signals were amplified and then bandpass filtered between 20 and 10 kHz. An auditory feedback EMG signal was produced to ensure complete voluntary relaxation of the target muscle. TMS was delivered through a focal figure-of-eight-shaped magnetic coil (70 mm internal diameter) connected to two Magstim 200 magnetic stimulators via a BiStim-module (Magstim, Camarthenshire, UK). Patients were seated in an armchair with their head fixed in a plastic foam headrest. The magnetic coil was placed over the motor cortex by finding and marking a scalp site that was producing maximal MEPs in the FDI muscle when the current induced in the brain flowed posterior to anterior approximately perpendicularly to the assumed line of the central sulcus.18
1) The resting motor threshold (RMT) was defined as the lowest stimulator output intensity capable of inducing MEPs with peak-to-peak amplitude of at least 50 µV in a relaxed FDI muscle in at least four out of eight consecutive trials. A step width of 1% of maximum stimulator output was used to determine motor thresholds.
2) Peak-to-peak MEP amplitudes were measured in a relaxed FDI at three stimulus intensities (i.e., 120%, 140%, and 150% of the RMT). TMS stimuli (eight stimuli at each stimulus intensity) were delivered randomly 5 s apart, and average MEP amplitudes were calculated at each intensity.
3) Cortical silent periods (CSPs) were measured over eight trials at three stimulus intensities (i.e., 120%, 140%, and 150% of the RMT), in moderately active FDI muscles (at approximately 30% of the maximum voluntary contraction).20 TMS stimuli were delivered randomly 5 s apart, and eight stimuli were delivered at each stimulus intensity. The CSP was defined as the time between the first turning point of a stimulus-induced MEP and the first reoccurrence of rectified voluntary EMG activity. The time of the first turning point was determined by the EMG recording device. The offset times of CSPs were determined by a single blinded investigator (Joo EY). The average CSP was calculated at each stimulus intensity.21
4) Intracortical inhibition (ICI) and intracortical facilitation (ICF) were determined at interstimulus intervals of 2 or 3 ms (i.e., short intracortical inhibition) and 10 or 15 ms (ICF) using a previously described protocol.22,23 The conditioning stimulus was set to 80% of the RMT, and as such produced no changes in spinal cord excitability.22 The intensities of subsequent suprathreshold test stimuli were adjusted to produce MEPs with a peak-to-peak amplitude of approximately 1.5 mV at rest in all baseline and effect measurements. TMS intensity was adjusted before and after ZNS [i.e., whether the test stimulation intensity for ICI (1.5 mV) was re-evaluated after ZNS or was the same intensity as for pre-ZNS]. Eight trials of single control test stimuli and eight paired stimuli of each ISI were recorded; stimuli were delivered 5 s apart in random order, as generated by the computer program. An average of eight trials was used to define the peak-to-peak amplitudes of MEPs. A conditioned response is defined as the mean amplitude of the conditioned responses belonging to the ISI, and is expressed as a percentage of the mean amplitude of the unconditioned test responses.
All five motor cortex excitability parameters were measured before (before ZNS) and 3.5 hours after (after ZNS) ZNS administration, adjusted to the pharmacokinetics of the ZNS. The plasma ZNS concentration peaked at 2-5 hours after administering single oral doses of 200-mg ZNS in healthy volunteers.24,25 However, actual serum concentrations of ZNS during TMS sessions were not measured in the present study because no assay method for measuring ZNS is currently available in Korea.
TMS parameters were obtained in both epileptic and nonepileptic hemispheres. TMS parameters in these hemispheres were compared to document interhemisphere differences before ZNS and after ZNS. None of the 24 patients had a seizure during the 5 days before the TMS studies were performed.
The investigators who took measurements and analyzed the raw data were blinded to the experiment details until all data had been acquired. Stimulation sessions lasted approximately 1.5-2 hours. Consent was obtained from all patients after the study protocol had been explained to them. The Institutional Review Board at Samsung Medical Center authorized the informed consent form and the study protocol.
To investigate the effect of ZNS on cortical excitability, the paired t-test was used to compare paired TMS parameters (RMT, MEP, CSP, and ICI/ICF) before and after ZNS administration. The parametric paired t-test or the nonparametric Wilcoxon's signed rank test was used for normally and not-normally distributed TMS parameters measured before and after ZNS administration. Multiple comparisons were performed using Bonferroni's correction. The Mann-Whitney test was used to perform interhemisphere comparisons of TMS parameters (epileptic and nonepileptic hemispheres). Repeated-measures ANOVA was used to compare the changes in MEP or CSP values induced by ZNS at different stimulus intensities and in ICI or ICF values by the interstimulus interval. The level of statistical significance in all analyses was set at p<0.05. Data are expressed as mean±SEM values.
The patients were 29.3±6.3 years old (range 21-41 years) and had suffered from epilepsy for 7.6±3.3 months (2-14 months). All patients underwent brain MRI and 99mTc-ethylcysteinate dimer single-photon-emission computed tomography within 1 year of diagnosis. Four of the 24 patients exhibited brain MRI abnormalities. The scalp EEG recording confirmed the location of the epileptic focus in all cases.
Four of the patients showed atrophy and signal changes in the unilateral hippocampus on MRI, and definite interictal epileptiform discharges were recorded in the ipsilateral temporal region to hippocampal atrophy during routine EEG recordings. Of these patients, three (1, 2, and 3 patients) had a history of complex focal seizures (dialeptic or automotor seizures after abdominal aura), and the remaining patient (4 patient) had complex partial seizures followed by occasional secondarily generalized SGTCS.
Eight patients with a history of secondarily generalized SGTCS exhibited EEG seizures as well as interictal spikes recorded in the left temporal (5-10 patients) or right temporal lobes (11 and 12 patients) during routine EEG recordings.
Eleven patients with normal MRI findings underwent long-term video-EEG monitoring to confirm the epileptic focus. Four of these patients (13-16 patients) exhibited typical complex focal seizures (dialeptic or automotor seizures with a preceding abdominal aura) followed by secondarily generalized tonic-clonic seizures arising from the ipsilateral mesial temporal area during their monitoring periods. Two patients (17 and 18 patients) exhibited auditory aura followed by complex focal seizures arising from the left lateral temporal region. 19 Patient exhibited visual aura and brief complex focal seizures followed by secondarily generalized SGTCS with ictal repetitive spikes arising from left occipital lobe. In two patients with a history of nocturnal generalized SGTCS, video-EEG monitoring revealed frequent interictal sharp waves and ictal EEG discharges on the left (20 patient) or right (21 patient) frontal lobe during sleep. 22 and 23 patients, who suffered from somatosensory auras (paresthesia or tingling sensation in unilateral face or arm), followed by complex focal seizures (version of head to the contralateral side) and secondarily generalized SGTCS exhibited frequent spikes or polyspikes and ictal EEG discharges on the contralateral parietal region to the side of somatosensory auras.
A 24-year-old male (24 patient)with a history of episodes of left-arm clonic seizures without loss of consciousness and nocturnal generalized SGTCS had interictal spikes in the right frontocentral area on sleep EEG recordings. The clinical information and 99mTc-ethylcysteinate dimer single-photon-emission computed tomography findings are summarized in Table 1.
Fourteen of our 24 patients (58%) achieved a seizure-free state at a ZNS dosage of 200 mg/day between treatment weeks 6 and 8. Eight patients suffered habitual complex partial seizures or simple partial seizures at ZNS 200 mg/day; three of these became seizure free at 250 mg/day, and the other five became seizure free at 300 mg/day. Two patients were seizure free at 200 mg/day, but required dosage reduction to 150 or 175 mg/day due to a loss of appetite, weight loss, and dizziness. Nevertheless, these patients maintained a seizure-free state. No patient experienced an unexpected serious adverse event.
Parametric statistical analyses were used in the study because we were able to assume that the data were normally distributed after the normality testing (Table 2).
The RMT did not change after ZNS administration in either hemisphere (p>0.05, paired t-test with multiple comparisons). Peak-to-peak MEP amplitudes increased with the stimulus intensity. ZNS significantly reduced the MEP amplitudes in epileptic hemispheres at all stimulus intensities (p=0.005-0.006). ZNS did not suppress the MEP amplitudes in nonepileptic hemispheres (Fig. 1). The CSP lengthened approximately linearly with intensity, but ZNS did not change the CSP of epileptic and nonepileptic hemispheres. Short intracortical inhibition and ICF were unchanged by ZNS in both hemispheres. Repeated-measures ANOVA did not reveal a significant main effect for either intensity or ISI (p>0.05).
We found that therapeutic doses of ZNS significantly decreased the MEP amplitudes only in the epileptic hemisphere of focal epilepsy patients. Mean MEP amplitudes in focal epilepsy patients before ZNS showed a tendency to be higher in the epileptic hemisphere than in the nonepileptic hemisphere at all stimulus intensities, although the difference did not reach statistical significance. Some authors noted that the effect of AEDs on MEP size appeared to be not so clearly related to different modes of action.7 Others have argued that only MEP amplitude is affected and that it must therefore be the most sensitive measure of AED action.18,26
In the present study, ZNS significantly decreased MEP amplitudes only in epileptic hemispheres, and not in nonepileptic hemispheres. There is some evidence from animal experiments that MEPs are suppressed in the hemipshere ipsilateral to the epileptic focus. In a limbic seizure model induced by a unilateral microinjection of kainic acid into the rat amygdala, ZNS at 100 mg/kg significantly decreased the local cerebral glucose utilization in the ipsilateral sensorimotor cortex and hippocampus, in contrast to normal saline injection.27 During limbic status epilepticus, ZNS markedly attenuated seizures in the sensorimotor cortex, and a high uptake of 14C-ZNS was noted ipsilaterally in the sensorimotor cortex, parietal cortex, and thalamus in an autoradiographic study.28 These experiments demonstrate that ZNS inhibits seizure propagation from the epileptic hemisphere in focal epilepsy and suggest that the cortical excitability of epileptic hemispheres is more susceptible to attenuation by ZNS. However, those authors recognized that despite various studies of the effects of ZNS, the mechanism of its anticonvulsive action remains unclear.
We have previously reported the effects of ZNS on cortical excitability in patients with IGE.18 With a similar dosage administered for a similar period, we found that ZNS decreased MEP amplitudes bilaterally in IGE. One of the mechanisms of action of ZNS that supports its efficacy in generalized seizures is blockage of the low-threshold T-type calcium channels, which may confer efficacy against absence seizures.29-32 It is not uncommon to find disappearance of generalized epileptiform discharges after treatment with ZNS in patients with IGE.33,34 These findings support the bilateral reduction of MEPs in patients with IGE and unilateral decline in epileptic hemispheres in focal epilepsy patients after ZNS administration.
RMT findings in different studies have yielded disparate findings: some have found that RMTs were reduced in untreated IGE patients,35 some have found normal RMTs,36,37 and others have found increased RMTs.38 In the present study, the mean RMT appeared to be higher in the epileptic hemisphere than in the nonepileptic hemisphere at all stimulus intensities, but this finding did not reach statistical significance. Moreover, the mean RMT was not changed after treatment of ZNS in either the epileptic or nonepileptic hemisphere. Another study of 27 drug-naïve focal epilepsy patients revealed a significant interhemisphere RMT difference (i.e., higher values in the epileptic hemisphere).11 Their frontal lobe epilepsy patients also did not have definite MRI abnormalities (while it was not clear whether their epileptic foci in the frontal lobe were located in the motor cortex or not). Three of our 24 patients also had no MRI lesion, although an epileptic focus in the left or right frontal lobe was determined by long-term video-EEG monitoring. However, it is unlikely that epileptic focus per se affected the RMT in this study, because foci were located far beyond the motor cortex (i.e., two in the prefrontal area and one in the orbitofrontal cortex). It could be speculated that not only motor cortex involvement of epileptic focus but also seizure propagation to the motor cortex may induce RMT changes. Overall, the differential results from various TMS studies may be attributable to the location of the epileptic focus, seizure type, the time from last seizure, and the frequency and propagation pattern of the seizures.
TMS has been used to characterize the changes in motor cortex excitability associated with AED administration (mostly single dose) in healthy volunteers.39,40 A single oral dose of carbamazepine in healthy volunteers was found to increase the RMT without changing either ICI or ICF,41 whereas 1 week of carbamazepine in patients with neuropathic pain did not change the RMT.42 Furthermore, single oral doses of lamotrigine in healthy volunteers increased the RMT without changing either ICI or ICF,40 but chronic lamotrigine treatment in a group of healthy volunteers undergoing a 5-week session elevated the RMT and ICI, and these changes occurred in parallel with an increase in plasma levels.43 The ability to transfer short-term effects to the longer term is limited given known changes in the rate of metabolism, drug-induced changes in receptor densities or receptor coupling efficiencies, and sensitization.43 Thus, the short- and long-term effects of an AED may differ. Furthermore, the findings of TMS studies may differ between healthy volunteers and epilepsy patients. In the present study, we investigated the effects of the long-term administration of ZNS on the brains of drug-naïve epilepsy patients, and it is evident based on the findings of previous studies that the short- and long-term effects of ZNS might be quite different.
The findings of the present TMS study suggest that ZNS alters cortical excitability in brains with focal epilepsy. The MEP amplitudes were significantly reduced after ZNS administration only in epileptic hemispheres, and they may be useful for evaluating ZNS-induced changes in cortical excitability. Other TMS parameters were unaffected by ZNS.
Figures and Tables
Fig. 1
Changes in motor evoked potential (MEP) amplitudes after zonisamide (ZNS) administration in epilepsy patients. After ZNS treatment, MEP amplitudes were significantly reduced at 120%, 140%, and 150% of the resting motor threshold (RMT) stimulation only in epileptic hemispheres. *Paired t-test after applying Bonferroni's correction for multiple comparisons (p<0.05). Data are presented as medians (horizontal lines through the boxes) with the first and third quartiles (lower and upper lines, respectively) and the smallest and largest values (whiskers extending from the ends of the boxes). Pre-ZNS: before ZNS administration, Post-ZNS: after the ZNS administration, RMT: resting motor threshold.
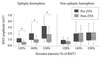
Table 1
Clinical characteristics of the patients
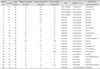
*Number of seizures before the first transcranial magnetic stimulation (i.e., before zonisamide treatment).
MRI: magnetic resonance imaging, ECD-SPECT: 99mTc-ethylcysteinate dimer single-photon-emission computed tomography, rCBF: regional cerebral blood flow, HIS: high signal intensity in the unilateral hippocampus on brain MRI, HA: hippocampal atrophy in the unilateral hippocampus on brain MRI, R: right, L: left.
Acknowledgements
This study was supported by a grant (M103KV010016-08K2201-01610) from the Brain Research Center of the 21st Century Frontier Research Program funded by the Ministry of Science and Technology of the Republic of Korea and by the Samsung Medical Center Clinical Research Development Program grain, CRS 109-19-1.
References
1. Joo EY, Hong SB, Han HJ, Tae WS, Kim JH, Han SJ, et al. Postoperative alteration of cerebral glucose metabolism in mesial temporal lobe epilepsy. Brain. 2005. 128:1802–1810.


2. Lee HW, Hong SB, Tae WS. Opposite ictal perfusion patterns of subtracted SPECT. Hyperperfusion and hypoperfusion. Brain. 2000. 123:2150–2159.


3. Luders HO, Engle J, Munari C. Engel J, editor. General principles. Surgical Treatment of the Epilepsies. 1993. New York: Raven Press;137–153.
4. Tae WS, Joo EY, Kim JH, Han SJ, Suh YL, Kim BT, et al. Cerebral perfusion changes in mesial temporal lobe epilepsy: SPM analysis of ictal and interictal SPECT. Neuroimage. 2005. 24:101–110.


5. Manganotti P, Bongiovanni LG, Zanette G, Turazzini M, Fiaschi A. Cortical excitability in patients after loading doses of lamotrigine: a study with magnetic brain stimulation. Epilepsia. 1999. 40:316–321.


6. Ziemann U, Rothwell JC, Ridding MC. Interaction between intracortical inhibition and facilitation in human motor cortex. J Physiol. 1996. 496:873–881.


7. Hallett M, Chokroverty S. Magnetic Stimulation in Clinical Neurophysiology. 2005. Philadelphia: Elsevier.
8. Delvaux V, Alagona G, Gérard P, De Pasqua V, Delwaide PJ, Maertens de Noordhout A. Reduced excitability of the motor cortex in untreated patients with de novo idiopathic "grand mal" seizures. J Neurol Neurosurg Psychiatry. 2001. 71:772–776.


9. Reutens DC, Berkovic SF, Macdonell RA, Bladin PF. Magnetic stimulation of the brain in generalized epilepsy: reversal of cortical hyperexcitability by anticonvulsants. Ann Neurol. 1993. 34:351–355.


10. Werhahn KJ, Lieber J, Classen J, Noachtar S. Motor cortex excitability in patients with focal epilepsy. Epilepsy Res. 2000. 41:179–189.


11. Badawy RA, Curatolo JM, Newton M, Berkovic SF, Macdonell RA. Changes in cortical excitability differentiate generalized and focal epilepsy. Ann Neurol. 2007. 61:324–331.


12. Brodie MJ, Duncan R, Vespignani H, Solyom A, Bitenskyy V, Lucas C. Dose-dependent safety and efficacy of zonisamide: a randomized, double-blind, placebo-controlled study in patients with refractory partial seizures. Epilepsia. 2005. 46:31–41.


13. Faught E, Ayala R, Montouris GG, Leppik IE. Zonisamide 922 Trial Group. Randomized controlled trial of zonisamide for the treatment of refractory partial-onset seizures. Neurology. 2001. 57:1774–1779.


14. Park SP, Kim SY, Hwang YH, Lee HW, Suh CK, Kwon SH. Long-term efficacy and safety of zonisamide monotherapy in epilepsy patients. J Clin Neurol. 2007. 3:175–180.


15. Sackellares JC, Ramsay RE, Wilder BJ, Browne TR 3rd, Shellenberger MK. Randomized, controlled clinical trial of zonisamide as adjunctive treatment for refractory partial seizures. Epilepsia. 2004. 45:610–617.


16. Sobieszek G, Borowicz KK, Kimber-Trojnar Z, Małtek R, Piskorska B, Czuczwar SJ. Zonisamide: a new antiepileptic drug. Pol J Pharmacol. 2003. 55:683–689.
17. Leppik IE. Zonisamide: chemistry, mechanism of action, and pharmacokinetics. Seizure. 2004. 13:Suppl 1. S5–S9. discussion S10.


18. Joo EY, Kim SH, Seo DW, Hong SB. Zonisamide decreases cortical excitability in patients with idiopathic generalized epilepsy. Clin Neurophysiol. 2008. 119:1385–1392.


19. Eisai Pharmaceuticals Inc. Zonegran Zonisamide Capsules Prescribing Information. 2004. Teaneck, NJ: Eisai Pharmaceuticals Inc..
20. Tergau F, Wanschura V, Canelo M, Wischer S, Wassermann EM, Ziemann U, et al. Complete suppression of voluntary motor drive during the silent period after transcranial magnetic stimulation. Exp Brain Res. 1999. 124:447–454.


21. Ziemann U, Chen R, Cohen LG, Hallett M. Dextromethorphan decreases the excitability of the human motor cortex. Neurology. 1998. 51:1320–1324.


22. Kujirai T, Caramia MD, Rothwell JC, Day BL, Thompson PD, Ferbert A, et al. Corticocortical inhibition in human motor cortex. J Physiol. 1993. 471:501–519.


23. Reis J, Tergau F, Hamer HM, Müller HH, Knake S, Fritsch B, et al. Topiramate selectively decreases intracortical excitability in human motor cortex. Epilepsia. 2002. 43:1149–1156.


24. Ito T, Yamaguchi T, Miyazaki H, Sekine Y, Shimizu M, Ishida S, et al. Pharmacokinetic studies of AD-810, a new antiepileptic compound. Phase I trials. Arzneimittelforschung. 1982. 32:1581–1586.
25. Taylor CP, McLean JR, Bockbrader HN, Budannan RA, Xarasaua T, Meyazaki M, et al. Meldrum BS, Porter RJ, editors. Zonisamide (AD-810, CI-912). New Anticonvulsant Drugs. 1986. London: John Libbey;277–294.
26. Sohn YH, Kaelin-Lang A, Jung HY, Hallett M. Effect of levetiracetam on human corticospinal excitability. Neurology. 2001. 57:858–863.


27. Takano K, Tanaka T, Fujita T, Nakai H, Yonemasu Y. Zonisamide: electrophysiological and metabolic changes in kainic acid-induced limbic seizures in rats. Epilepsia. 1995. 36:644–648.


28. Akaike K, Tanaka S, Tojo H, Fukumoto S, Imamura S, Takigawa M. Regional accumulation of 14C-zonisamide in rat brain during kainic acid-induced limbic seizures. Can J Neurol Sci. 2001. 28:341–345.


29. Futatsugi Y, Riviello JJ Jr. Mechanisms of generalized absence epilepsy. Brain Dev. 1998. 20:75–79.


30. Kim D, Song I, Keum S, Lee T, Jeong MJ, Kim SS, et al. Lack of the burst firing of thalamocortical relay neurons and resistance to absence seizures in mice lacking alpha(1G) T-type Ca(2+) channels. Neuron. 2001. 31:35–45.


31. Kito M, Maehara M, Watanabe K. Mechanisms of T-type calcium channel blockade by zonisamide. Seizure. 1996. 5:115–119.


32. Suzuki S, Kawakami K, Nishimura S, Watanabe Y, Yagi K, Seino M, et al. Zonisamide blocks T-type calcium channel in cultured neurons of rat cerebral cortex. Epilepsy Res. 1992. 12:21–27.


33. Marinas A, Villanueva V, Giráldez BG, Molins A, Salas-Puig J, Serratosa JM. Efficacy and tolerability of zonisamide in idiopathic generalized epilepsy. Epileptic Disord. 2009. 11:61–66.
34. Szaflarski JP. Effects of zonisamide on the electroencephalogram of a patient with juvenile myoclonic epilepsy. Epilepsy Behav. 2004. 5:1024–1026.


35. Reutens DC, Berkovic SF. Increased cortical excitability in generalised epilepsy demonstrated with transcranial magnetic stimulation. Lancet. 1992. 339:362–363.


36. Macdonell RA, King MA, Newton MR, Curatolo JM, Reutens DC, Berkovic SF. Prolonged cortical silent period after transcranial magnetic stimulation in generalized epilepsy. Neurology. 2001. 57:706–708.


37. Manganotti P, Zanette G. Contribution of motor cortex in generation of evoked spikes in patients with benign rolandic epilepsy. Clin Neurophysiol. 2000. 111:964–974.


38. Gianelli M, Cantello R, Civardi C, Naldi P, Bettucci D, Schiavella MP, et al. Idiopathic generalized epilepsy: magnetic stimulation of motor cortex time-locked and unlocked to 3-Hz spike-and-wave discharges. Epilepsia. 1994. 35:53–60.


39. Chen R, Samii A, Caños M, Wassermann EM, Hallett M. Effects of phenytoin on cortical excitability in humans. Neurology. 1997. 49:881–883.


40. Ziemann U, Lönnecker S, Steinhoff BJ, Paulus W. Effects of antiepileptic drugs on motor cortex excitability in humans: a transcranial magnetic stimulation study. Ann Neurol. 1996. 40:367–378.


41. Ziemann U, Lönnecker S, Steinhoff BJ, Paulus W. The effect of lorazepam on the motor cortical excitability in man. Exp Brain Res. 1996. 109:127–135.

