Abstract
Background and purpose
Elevated plasma total homocysteine (tHcy) levels are reported to be associated with an increased risk of Alzheimer's disease (AD). However, the mechanism by which homocysteine contributes to the pathogenesis of AD is as yet unknown. The aim of this study was to elucidate the relationship between white matter changes (WMC) and medial temporal lobe atrophy (MTA) on brain magnetic resonance imaging (MRI), and plasma levels of tHcy in AD patients.
Methods
Seventy-two patients with a clinical diagnosis of probable AD were recruited to the study. Plasma tHcy levels, vascular risk factors, and WMC and MTA on brain MRI were evaluated in all patients. The AD patients were classified into two groups: those with no or minimal WMC (69.2±8.8 years, mean±SD, n=36) and those with moderate-to-severe WMC (74.6±4.6 years, n=36) on brain MRI.
Results
In a univariate logistic regression analysis, the risk of moderate-to-severe WMC in AD was significantly associated with increasing age, female gender, lower education level, hypertension, high plasma tHcy levels, and lower Mini-Mental State Examination (MMSE) score. Multivariate logistic regression analysis revealed only high plasma tHcy as the independent and significant risk factor for moderate-to-severe WMC [odds ratio (OR; adjusted for age, gender, education level, MMSE score, and hypertension comparing the top tertile - tHcy levels ≥12.9 µmol/L - with the bottom tertile - tHcy levels ≤9.4 µmol/L)=7.35; 95% confidence interval, confidence interval=1.36-39.84; p=0.02], and age as a borderline significant risk factor (OR=1.08, 95% CI=0.99-1.19, p=0.09) in AD patients. Plasma tHcy levels were not correlated significantly with either right or left MTA.
Elevated plasma total homocysteine (tHcy) levels are associated with an increased risk of clinical stroke and cardiovascular disease.1,2 Findings of previous studies have suggested that an elevated plasma tHcy level is associated with silent brain infarcts and white-matter lesions on magnetic resonance imaging (MRI) of the brain in healthy elderly and middle-aged adults.3-5 The presence of cerebral hyperintensities in the deep subcortical and periventricular white matter on brain MRI is a common finding in elderly people.6 Subjects with cerebral white-matter changes (WMC) may have an increased risk of either ischemic or hemorrhagic stroke.7-9 Thus, the presence of extensive WMC may be considered an indicator of subclinical vascular injury. White matter lesions are also more common in patients with Alzheimer's disease (AD) than in the nondemented elderly.10,11
It has recently been reported that increased plasma tHcy levels are associated with an increased risk of the development of neurodegenerative diseases such as AD, Parkinson's disease, and amyotrophic lateral sclerosis.12-14 Higher plasma tHcy levels appear to be associated with an increased risk of silent brain infarction on brain MRI,15 and with moderate-to-severe WMC on brain computed tomography (CT) in individuals with AD.16 Homocysteine has been shown to potentiate amyloid beta-peptide-mediated toxicity in primary neuronal cultures.17 However, the mechanisms underlying the association between high plasma tHcy with AD are uncertain and may involve vascular or neuronal pathways, or both.
Medial temporal lobe atrophy (MTA) on MRI is the first and most striking manifestation of AD.18 MTA, which is correlated with Braak staging, and plaques and tangles in the hippocampus,19 is an accepted measure of neuronal loss. To our knowledge, the association between plasma tHcy levels and MTA has not been studied in AD patients.
If homocysteine increases the risk of AD via a vascular toxic mechanism, WMC may be associated with increased plasma tHcy in AD. However, if homocysteine acts in AD pathogenesis via a cellular toxic mechanism, a higher plasma tHcy may not be associated with WMC, but rather with MTA. The objective of this study was thus to elucidate the relationship between WMC and MTA on brain MRI, and plasma tHcy levels in AD patients.
Seventy-two patients with AD were serially recruited in a memory clinic of the Department of Neurology at Inha University Hospital between January 2004 and October 2008. The participants included in the present study were those who met the criteria for probable AD established by the National Institute of Neurological and Communicative Disorders and Stroke-Alzheimer's Disease and Related Disorders Association,20 and who submitted to a brain MRI and measurement of plasma tHcy levels. Subjects were excluded if they had a history of pernicious anemia, gastric surgery, neoplasia, hepatorenal disease, malabsorption syndrome, or vegetarianism, were taking medication associated with increased tHcy levels, such as thiazide, diuretics, azathioprine, phenytoin, and methotrexate, or had abnormally low levels of serum vitamin B12 and folate.21 Subjects were also excluded if the time interval between the brain MRI and blood sampling for measuring plasma tHcy was more than 2 months.
Blood samples were collected from the subjects after overnight fasting, and plasma tHcy was determined by high-performance liquid chromatography, as described elsewhere.22 Serum vitamin B12 and folate levels were measured using radioimmunoassay. The study protocol was reviewed and approved by the institutional review board of Inha University Hospital.
Hypertension, diabetes mellitus (DM), heart disease, hyperlipidemia, and smoking were evaluated in all patients. Hypertension was defined as a systolic blood pressure (BP) of ≥140 mmHg, a diastolic BP of ≥90 mmHg, or use of antihypertensive medication according to the World Health Organization guidelines for the management of hypertension.23 DM was defined based on current treatment with insulin, oral hypoglycemic medication, or an 8-hour fasting plasma glucose level of ≥126 mg/dL, as indicated by the American Diabetes Association.24 For the definition of hypercholesterolemia, we followed the criteria established by the National Cholesterol Education Program-Adult Treatment Panel II based on a total cholesterol level of >200 mg/dL, a low-density lipoprotein cholesterol level of >130 mg/dL, and a high-density lipoprotein cholesterol level of <35 mg/dL; hypertriglyceridemia was based on serum triglyceridemia (>200 mg/dL).25 Heart disease was defined based on a history of myocardiac infarction, angina pectoris, or heart failure, and atrial fibrillation on ECG. Patients were classified as being either current smokers or nonsmokers.
All participating patients had submitted to a brain MRI, which was performed on a 1.5-T superconducting magnet (Signa, GE Medical Systems, Milwaukee, WI, USA). Axial T1-weighted, T2-weighted, and fluid-attenuated inversion recovery (FLAIR) images were obtained at a slice thickness of 5 mm for all subjects. T1-weighted coronal images were obtained in 25 subjects and T2-weighted coronal images were obtained in 45 subjects. The degree of WMC was rated on axial T2-weighted and FLAIR images by one reader who was blind to the clinical details, using the visual scale of Fazekas et al.26 Patients were classified as having one of the following:
1) Minimal or no WMC: If there were either no or only slight periventricular hyperintensities with caps or rims with a maximum diameter of <10 mm, and either absent or single lesions of <10 mm and no confluent lesions in the deep subcortical white-matter area.
2) Moderate-to-severe WMC: If there were moderate or severe periventricular hyperintensities with caps or rims with a maximum diameter of ≥10 mm, or single or grouped lesions ≥10 mm in any diameter in the deep subcortical white-matter area.
A standardized five-point scale was used to rate right- and left-sided MTA from T1- or T2-weighted coronal images.18 The five-point scale (0=absent, 1=minimal, 2=mild, 3=moderate, 4=severe) rated atrophy based on the height of the hippocampal formation and the width of the cerebrospinal fluid spaces. All scans were assessed by one rater who was blinded to the clinical details.
Student's t-test was used to evaluate differences in age, education level, Mini-Mental State Examination (MMSE) score, and the levels of plasma tHcy, vitamin B12, and folate between AD patients with no or minimal WMC and those with moderate-to-severe WMC. The chi-square test was used to assess differences in gender, hypertension, DM, hypercholesterolemia, hypertriglyceridemia, heart disease, and smoking habit between these two groups of AD patients. Logistic regression analysis was used to assess the influence of explanatory variables on WMC. Using a dichotomous approach, the reference group comprised AD patients with no or minimal WMC on brain MRI.
Plasma tHcy levels were categorized into tertiles, and odds ratios (ORs) were calculated by dividing the prevalence ratio in each tertile by the ratio in the lowest tertile in simple and multiple logistic regression models. We analyzed the association between tertiles of plasma tHcy and moderate-to-severe WMC by multiple logistic regression adjusted for age, gender, education level, MMSE score, and hypertension. Spearman's correlation coefficient was used to evaluate the association between MTA and plasma tHcy levels.
Of the 72 AD patients recruited to the study, 36 (50%) had moderate-to-severe WMC and 36 (50%) had no or minimal WMC. The time interval between the brain MRI and blood sampling for measurement of plasma tHcy was 4.2±8.8 days (mean±SD; range 0-50 days). The demographic and clinical characteristics of the two groups of patients are displayed in Table 1. The group of AD patients with moderate-to-severe WMC were older on average and contained a higher proportion of females than those with no or minimal WMC. The patients with no or minimal WMC had more years of education, higher MMSE scores, a lower prevalence of hypertension, and lower plasma levels of tHcy than those with moderate-to-severe WMC. There were no significant differences in the prevalences of DM, hypercholesterolemia, hypertriglyceridemia, heart disease, smoking habit, and levels of serum vitamin B12 and folate between the two groups of AD patients.
In a univariate logistic regression analysis, the risk of moderate-to-severe WMC was associated with increasing age [OR=1.12; 95% confidence interval (CI)=1.04-1.22; p=0.004] and hypertension (OR=3.14, 95% CI=1.20-8.24, p=0.02). The top tertile of plasma tHcy (≥12.9 µmol/L) was at risk of moderate-to-severe WMC in comparison with the bottom tertile (≤9.4 µmol/L; OR=4.86, 95% CI=1.43-16.50, p=0.01). In addition, a high education level (OR=0.83, 95% CI=0.74-0.94, p=0.003), high MMSE score (OR=0.80, 95% CI=0.70-0.91, p=0.001), and male gender (OR=0.18, 95% CI=0.05-0.60, p=0.006) showed protective effects of moderate-to-severe WMC in AD patients (Table 2).
Multivariate logistic regression analysis revealed only high plasma tHcy as an independent and significant risk factor for moderate-to-severe WMC in AD patients [OR (adjusted for age, gender, education, MMSE score, and hypertension comparing the top tertile - tHcy levels ≥12.9 µmol/L - with the bottom tertile - tHcy levels ≤9.4 µmol/L)=7.35, 95% CI=1.36-39.84, p=0.02; Table 3]. Age had a borderline association with moderate-to-severe WMC in AD patients (multivariate logistic regression: OR=1.08, 95% CI=0.99-1.19, p=0.09). Education, gender, MMSE score, and hypertension were not significantly associated with moderate-to-severe WMC in AD patients (multivariate analysis using logistic regression).
Plasma tHcy levels were not correlated significantly with atrophy of either the right (R=-0.06, p=0.62) or left (R=-0.07, p=0.54) medial temporal lobe in all AD patients. There were no significant correlations between right and left MTA, and plasma tHcy levels in any of the AD patients, regardless of the degree of WMC.
We found an independent, cross-sectional association between higher plasma tHcy levels and moderate-to-severe WMC on brain MRI in patients with AD. This is consistent with the previous finding that higher plasma tHcy levels are associated with WMC on brain CT in AD patients.16 WMC were encountered in 60% of the brains from autopsy-confirmed cases of AD, and the lesions had the characteristics of incomplete infarction.10
In the present study, older age, lower education, lower MMSE score, female gender, and hypertension were associated with moderate-to-severe WMC, as shown using univariate logistic regression analyses. However, they were not associated significantly with moderate-to-severe WMC in multivariate analyses. In addition, older age only had a borderline association with moderate-to-severe WMC (multivariate analysis). The education level of the elderly is low, and is generally lower for female elderly than for male elderly in South Korea. The significant effect of lower education (univariate analysis) might be due to the possible interactive effect with age. Since it has been shown that MMSE score is associated with education level,27 the lower education level of the AD patients with moderate-to-severe WMC might then be associated with lower MMSE scores in comparison with those with no or minimal WMC. The group of AD patients with moderate-to-severe WMC was 5 years older and had higher proportion of females in comparison with those with no or minimal WMC (88.9% vs. 58.3%, respectively). The significant effect of being female (univariate analysis) might thus be due to the possible interactive effects with age and education.
A recent study revealed that high plasma tHcy levels, but not hypertension and age, were only associated with an increased risk of developing silent brain infarcts in AD.15 WMC on brain CT were not associated with hypertension, but rather with high plasma tHcy levels.16 A longitudinal study showed that BP values were high 10-15 years before the onset of AD or WMC on brain CT, but then decreased in the more recent years before dementia onset.28 Dysfunction in central BP autoregulatory mechanisms due to chronic hypertension could lead to a reduction in cerebral blood flow at BP levels considered normal for normotensive subjects.29 AD patients with moderate-to-severe WMC might have a much higher BP prior to the drop that occurs before the onset of AD, and a concomitant dysfunction of BP autoregulatory mechanisms. A longitudinal study is needed to evaluate the relationship between hypertension and WMC in AD.
The association between higher plasma tHcy levels and moderate-to-severe WMC in AD suggests that the cause of the pathogenic process involving homocysteine is diffuse vascular damage. It has been shown that higher plasma tHcy levels in middle-aged women are an independent risk factor for lacunar infarcts, as observed using CT later in life,30 and that elevated tHcy levels are associated with peripheral arterial disease.31 The mechanism by which homocysteine might cause vascular damage is unclear. Experimental evidence suggests that homocysteine promotes atherogenesis by facilitating oxidative arterial injury, damaging the vascular matrix, and augmenting the proliferation of vascular smooth muscle.32
There are several theories as to the mechanisms by which vascular disorders influence the progress of AD. For example, it is presumed that amyloid deposition is caused by a vascular disorder.33 Alternatively, a vascular event may cause the progression of subclinical AD to a clinical stage.34 Another potential mechanism through which tHcy could affect WMC in AD may be a failure of myelin synthesis through hypomethylation of post-transcriptional myelin basic protein.35
We found no significant correlation between MTA and plasma tHcy levels in the AD patients. In two reports, a cross-sectional association has been described between plasma tHcy levels and hippocampal atrophy on MRI among nondemented elderly subjects,36,37 but a recent cohort study of a community-based sample did not confirm this association.3 To our knowledge, the present study is the first to describe an association between higher plasma tHcy levels and MTA in AD patients. The MTA rating scale has been validated on T1-weighted coronal images.18 However, MTA was rated on T1- or T2-weighted coronal images in our study; therefore we cannot rule out the possibility that the MTA is exaggerated in some patients.
Homocysteine has been shown to potentiate amyloid beta-peptide-mediated toxicity in primary neuronal cultures.17 Excitotoxicity by homocysteine and its derivatives such as homocysteic acid and homocysteic sulfinic acid may occur via stimulation of N-methyl-D-aspartate receptors and subsequent damage to neuronal DNA, thus increasing the susceptibility to apoptotic cell death, as demonstrated in cultures of hippocampal neurons.38 However, it is unlikely that homocysteine would reach concentrations high enough to trigger direct neurotoxic effects in vivo.13 Homocysteine may not be neurotoxic by itself, but might enhance the brain's vulnerability to concomitant neurotoxic stimuli such as beta amyloid.39 Our finding was not compatible with the notion that higher plasma levels of tHcy may contribute to the pathogenesis of AD via a direct neurotoxic effect.
Our study has the limitations of including only a small sample and having a cross-sectional design. Therefore, we cannot state categorically whether higher plasma tHcy levels are a cause or a coexistent finding of moderate-to-severe WMC in AD. A long-term prospective study is needed to clarify this matter.
The mechanism by which homocysteine may contribute to the pathogenesis of AD remains to be established. Our results support the theory that the vascular pathway can mediate the observed association between elevated plasma tHcy levels and AD.
Figures and Tables
Table 1
Differences in demographic and clinical variables between AD patients with no or minimal white matter change (WMC) and those with moderate-to-severe WMC on brain MRI
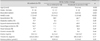
Acknowledgments
This study was supported by a grant of the Inha Univeristy College of Medicine (31555-01), Incheon, Republic of Korea.
References
1. Bostom AG, Silbershatz H, Rosenberg IH, Selhub J, D'Agostino RB, Wolf PA, et al. Nonfasting plasma total homocysteine levels and all-cause and cardiovascular disease mortality in elderly Framingham men and women. Arch Intern Med. 1999. 159:1077–1080.


2. Bostom AG, Rosenberg IH, Silbershatz H, Jacques PF, Selhub J, D'Agostino RB, et al. Nonfasting plasma total homocysteine levels and stroke incidence in elderly persons: the Framingham Study. Ann Intern Med. 1999. 131:352–355.


3. Seshadri S, Wolf PA, Beiser AS, Selhub J, Au R, Jacques PF, et al. Association of plasma total homocysteine levels with subclinical brain injury: cerebral volumes, white matter hyperintensity, and silent brain infarcts at volumetric magnetic resonance imaging in the Framingham Offspring Study. Arch Neurol. 2008. 65:642–649.
4. Vermeer SE, van Dijk EJ, Koudstaal PJ, Oudkerk M, Hofman A, Clarke R, et al. Homocysteine, silent brain infarcts, and white matter lesions: the Rotterdam Scan Study. Ann Neurol. 2002. 51:285–289.


5. Wright CB, Paik MC, Brown TR, Stabler SP, Allen RH, Sacco RL, et al. Total homocysteine is associated with white matter hyperintensity volume: the Northern Manhattan Study. Stroke. 2005. 36:1207–1211.


6. Pantoni L, Garcia JH. The significance of cerebral white matter abnormalities 100 years after Binswanger's report. A review. Stroke. 1995. 26:1293–1301.


7. Inzitari D. Leukoaraiosis: an independent risk factor for stroke? Stroke. 2003. 34:2067–2071.
8. Wong TY, Klein R, Sharrett AR, Couper DJ, Klein BE, Liao DP, et al. Cerebral white matter lesions, retinopathy, and incident clinical stroke. JAMA. 2002. 288:67–74.


9. Yamauchi H, Fukuda H, Oyanagi C. Significance of white matter high intensity lesions as a predictor of stroke from arteriolosclerosis. J Neurol Neurosurg Psychiatry. 2002. 72:576–582.


10. Brun A, Englund E. A white matter disorder in dementia of the Alzheimer type: a pathoanatomical study. Ann Neurol. 1986. 19:253–262.


11. Jellinger KA, Attems J. Prevalence and impact of cerebrovascular pathology in Alzheimer's disease and parkinsonism. Acta Neurol Scand. 2006. 114:38–46.


12. Seshadri S, Beiser A, Selhub J, Jacques PF, Rosenberg IH, D'Agostino RB, et al. Plasma homocysteine as a risk factor for dementia and Alzheimer's disease. N Engl J Med. 2002. 346:476–483.


13. Martignoni E, Tassorelli C, Nappi G, Zangaglia R, Pacchetti C, Blandini F. Homocysteine and Parkinson's disease: a dangerous liaison? J Neurol Sci. 2007. 257:31–37.


14. Zoccolella S, Simone IL, Lamberti P, Samarelli V, Tortelli R, Serlenga L, et al. Elevated plasma homocysteine levels in patients with amyotrophic lateral sclerosis. Neurology. 2008. 70:222–225.


15. Matsui T, Nemoto M, Maruyama M, Yuzuriha T, Yao H, Tanji H, et al. Plasma homocysteine and risk of coexisting silent brain infarction in Alzheimer's disease. Neurodegener Dis. 2005. 2:299–304.


16. Hogervorst E, Ribeiro HM, Molyneux A, Budge M, Smith AD. Plasma homocysteine levels, cerebrovascular risk factors, and cerebral white matter changes (leukoaraiosis) in patients with Alzheimer disease. Arch Neurol. 2002. 59:787–793.


17. White AR, Huang X, Jobling MF, Barrow CJ, Beyreuther K, Masters CL, et al. Homocysteine potentiates copper- and amyloid beta peptide-mediated toxicity in primary neuronal cultures: possible risk factors in the Alzheimer's-type neurodegenerative pathways. J Neurochem. 2001. 76:1509–1520.


18. Scheltens P, Leys D, Barlhof F, Huglo D, Weinstein HC, Vermersch P, et al. Atrophy of medial temporal lobes on MRI in "probable" Alzheimer's disease and normal ageing: diagnostic value and neuropsychological correlates. J Neurol Neurosurg Psychiatr. 1992. 55:967–972.


19. Burton EJ, Barber R, Mukaetova-Ladinska EB, Robson J, Perry RH, Jaros E, et al. Medial temporal lobe atrophy on MRI differentiates Alzheimer's disease from dementia with Lewy bodies and vascular cognitive impairment: a prospective study with pathological verification of diagnosis. Brain. 2009. 132:195–203.


20. McKhann G, Drachman D, Folstein M, Katzman R, Price D, Stadlan EM. Clinical diagnosis of Alzheimer's disease: report of the NINCDS-ADRDA Work Group under the auspices of Department of Health and Human Services Task Force on Alzheimer's disease. Neurology. 1984. 34:939–944.


21. Ueland PM, Refsum H. Plasma homocysteine, a risk factor for vascular disease: plasma levels in health, disease, and drug therapy. J Lab Clin Med. 1989. 114:473–501.
22. Ueland PM, Refsum H, Stabler SP, Malinow MR, Andersson A, Allen RH. Total homocysteine in plasma or serum: methods and clinical applications. Clin Chem. 1993. 39:1764–1779.


23. Guideline subcommittee. 1999 World Health Organization-International Society of Hypertension guidelines for the management of hypertension. J Hypertens. 1999. 17:151–183.
24. Report of the expert committee on the diagnosis and classification of diabetes mellitus. Diabetes Care. 1997. 20:1183–1197.
25. National cholesterol education program. Second report of the expert panel on detection, evaluation, and treatment of high blood cholesterol in adults (adult treatment panel II). Circulation. 1994. 89:1333–1445.
26. Fazekas F, Chawluk JB, Alavi A, Hurtig HI, Zimmerman RA. MR signal abnormalities at 1.5 T in Alzheimer's dementia and normal aging. AJR AM J Roentgenol. 1987. 149:351–356.


27. O'Connor DW, Pollitt PA, Treasure FP, Brook CP, Reiss BB. The influence of education, social class and sex on Mini-Mental State scores. Psychol Med. 1989. 19:771–776.
28. Skoog I, Lernfelt B, Landahl S, Palmertz B, Andreasson LA, Nilsson L, et al. 15-year longitudinal study of blood pressure and dementia. Lancet. 1996. 347:1141–1145.


29. Pantoni L, Garcia JH. Pathogenesis of leukoaraiosis: a review. Stroke. 1997. 28:652–659.
30. Zylberstein DE, Skoog I, Björkelund C, Guo X, Hultén B, Andreasson LA, et al. Homocysteine levels and lacunar brain infarcts in elderly women: the prospective population study of women in Gothenburg. J Am Geriatr Soc. 2008. 56:1087–1091.


31. Garofolo L, Barros N Jr, Miranda F Jr, D'Almeida V, Cardien LC, Ferreira SR. Association of increased levels of homocysteine and peripheral arterial disease in a Japanese-Brazilian population. Eur J Vasc Endovasc Surg. 2007. 34:23–28.


32. Hankey GJ, Eikelboom JW. Homocyseine and vascular disease. Lancet. 1999. 354:407–413.
33. Sun X, He G, Qing H, Zhou W, Dobie F, Cai F, et al. Hypoxia facilitates Alzheimer's disease pathogenesis by up-regulating BACE1 gene expression. Proc Natl Acad Sci U S A. 2006. 103:18727–18732.


34. Snowdon DA, Greiner LH, Mortimer JA, Riley KP, Greiner PA, Markesbery WR. Brain infarction and the clinical expression of Alzheimer disease. The Nun Study. JAMA. 1997. 277:813–817.


35. Weir DG, Scott JM. Brain function in the elderly: role of vitamin B12 and folate. Br Med Bull. 1999. 55:669–682.


36. den Heijer T, Vermeer SE, Clarke R, Oudkerk M, Koudstaal PJ, Hofman A, et al. Homocysteine and brain atrophy on MRI of non-demented elderly. Brain. 2003. 126:170–175.


37. Williams JH, Pereira EA, Budge MM, Bradley KM. Minimal hippocampal width relates to plasma homocysteine in community-dwelling older people. Age Ageing. 2002. 31:440–444.

