Abstract
Current evidence from monogenic Parkinson's disease (PD) supports the view that PD is a clinical syndrome, rather than a single disease entity, and that the heterogeneity of PD indeed reflects different pathogenesis. Recent developments in functional imaging have enabled the in vivo assessment of cellular and molecular pathology of PD with respect to temporal and topographical patterns. We propose that this new technology will be useful for linking monogenic and sporadic PD, and thus, for classifying PD based on the pathogenesis. It will be also useful in clinico-genetic studies exploring susceptibility factors and at-risk groups, which are important for neuroprotective treatment when it becomes available.
Positron emission tomography (PET) and single-photon emission computed tomography (SPECT) visualize and estimate quantitatively metabolic, biochemical or functional activities in a living brain; hence, called as functional imaging. Since SPECT and PET were first introduced in Korea in 1986 and 1994 respectively, technical advances and availability have grown at a remarkable pace. As of 2005, there are 187 SPECT cameras, 54 PET or PET/CT, 4 microPET, and 15 cyclotrons (which produce radiolabeled tracers) across the country; moreover, SPECT/CT fusion and high-resolution research tomograph (HRRT) will be introduced. In the future, it may be possible to see high-resolution Compton gamma camera and PET/MR fusion made in Korea. There is no doubt that the rapid expansion of this infrastructure will contribute enormously to efforts of neuroscience community searching for prevention or curative treatment of neurodegenerative disorders - the last frontier of medicine.
The goal of this review is to discuss the current issues on Parkinson's disease (PD), and establish the rationale for using this relatively new technology in clinical research and practice for PD.
In 1817 at the start of the Industrial Revolution, James Parkinson first reported PD in his monograph, "An Essay on the Shaking Palsy" based on his observations as a physician in East London. In 1888, Jean-Martin Charcot, then prominent French neurologist, pointed out that patients with this disorder do not have real weakness nor always have tremor, and thus, shaking palsy or paralysis agitans is a misnomer. He renamed this disorder as Parkinson's disease. In 1912, Friedrich Lewy, German neurologist in Alois Alzheimer's laboratory, first described Lewy bodies. In 1960, Hornykiewics showed that the dopamine content of the substantia nigra and corpus striatum in postmortem PD brains was extremely low, less than 10% of normal, ushering into an L-dopa era. In 1997, Polymeropoulos et al reported the first genetic cause of PD, mutations in the SNCA (α-synuclein) gene.1 Two months later, Spillantini et al showed that α-synuclein was a major component of Lewy bodies in sporadic PD, linking genetic and sporadic PD.2 It has opened the third era in PD.
The diagnosis of PD has been based on clinical and pathological features. UK PD Society Brain Bank clinical diagnostic criteria (one of most stringent criteria, widely used for research purposes; also known as QSBB criteria) consist of three steps: (1) diagnosis of parkinsonian syndrome; (2) exclusion of atypical features including significant family history and early severe dementia; (3) supportive prospective positive criteria observed during the longitudinal evaluation.3 The clinical diagnosis of PD is confirmed by postmortem pathological examinations with loss of pigmented neurons and the presence of Lewy bodies in the substantia nigra. Based on these criteria, 76% of clinically diagnosed PD was confirmed pathologically.4 This traditional way of defining PD is challenged by mounting new information derived from studies in familial PD.
Since the first report of a familial PD with mutations in α-synuclein gene in 1997, at least five more genes have been identified as a causative mutation in familial PD (Table 1).5 Subsequent clinical and pathological studies of affected people in these families revealed unexpected findings. First, patients with leucine-rich repeat kinase 2 (LRRK2) mutation showed pleomorphic pathology even within a family: Lewy bodies, tauopathy or neuronal loss without intracellular inclusions.6,7 Patients with parkin mutations showed dopamine neuronal loss without intracellular inclusions and/or tauopathy in most cases, and Lewy bodies in some with heterozygous mutation.8 Interestingly, previous pathological studies in clinically diagnosed sporadic PD also reported a few cases showing neuronal loss without intracellular inclusions9 or tauopathy.10 Second, clinical phenotypes and pathological features ranged from typical idiopathic PD to diffuse Lewy body disease between individuals with mutation in the same gene, encoding LRRK27 or α-synuclein multiplication. 11 Third, seemingly sporadic PD might in fact have a genetic basis, blurring the boundary between sporadic and familial PD.12-14 Therefore, significant family history should not exclude the diagnosis of PD,15 as in the current QSBB criteria. These observations also indicate that the presence of Lewy bodies in the postmortem brain cannot be used to "confirm" the disease16 because the pathology of PD is likely an outcome of disease process, i.e. phenotype, rather than a determining factor of the disease.
Traditional belief that PD is a relatively homogeneous disease entity, which can be separated from other similar disorders by applying stringent diagnostic criteria, has crumbled. Now, we have definite evidence for the longstanding argument that PD is a clinical syndrome.17,18 More than 100 years ago, Charcot changed the name of this disorder, "shaking palsy" that is a syndromic description, to "Parkinson's disease" that implies a homogeneous entity as a disease. After all, this revised term is turned out to be a misnomer again.
What is the impact of these new developments in everyday practice? Perhaps, the most compelling issue is whether the diagnosis of PD will be good enough for the treatment of disorder. Will this syndromic diagnosis guide us to tell patients the prognosis and definite treatment? From the clinician's viewpoint, it is most useful if a disease is defined and classified based on the pathogenesis. By analogy, the diagnosis of pneumonia is not sufficient for the treatment. The physician should understand the underlying pathogenic process, e.g. infection, autoimmune or aspiration. Then, is it possible to categorize idiopathic sporadic PD based on pathogenesis?
The study of genetics has been successful in identifying key proteins and causative mutations in familial PD. It will continue to make headway in elucidating molecular pathogenesis of familial PD. However, monogenic PD explains only 20% of the early onset PD and less than 3% of the late onset PD.19 The rest are all sporadic and idiopathic PD. Can we then relate findings in familial PD to sporadic PD?
Clinical phenotypes of familial PD, if all subtypes are lumped together, are as heterogeneous as those of sporadic PD. However, it is relatively homogeneous in each subtype of familial PD. The clinical phenotypes of dominant parkinsonism, especially Park8, are largely indistinguishable from typical late-onset idiopathic PD. The phenotype of Park1 is an aggressive dopa-responsive parkinsonism with earlier onset (mean age of 46.5 years) and more rapid progression, compared with typical idiopathic PD.20 It also shows a gene-dose effect on clinical and pathological phenotypes, more extensive with greater multiplication of α-synuclein gene.11 These findings clearly demonstrate that the mutated gene is a major, but not exclusive, factor determining clinical and pathological phenotypes. Mutations in α-synuclein gene are known to cause abnormal lipid and vesicle dynamics,21 while mutations in LRRK2 gene are to cause abnormal MAPKKK (mitogen-activated protein kinase kinase kinase) signaling.22,23
In contrast, the clinical phenotype of recessive parkinsonism (e.g. Park2, Park6 and Park7 with mutations in parkin, PTEN-induced putative kinase 1 (PINK1) and DJ-1 respectively) is typically a young-onset PD (YOPD, the onset between 20~40 years of age) with slow progression and good response to L-dopa. This triad of recessive genes is variably associated with mitochondria, and protective against mitochondrial dysfunction and oxidative stress.24,25 Although the pathological data are not available to all subtypes, at least, Park2 shows neuronal loss generally without protein aggregation, distinct from mutations in dominant genes that produce protein aggregation pathology.25 Therefore, while different gene mutations are associated with different pathogenic mechanisms and different clinical and pathological phenotypes, those with similar pathogenic mechanisms tend to show similar clinical phenotype (Fig. 1).
If one accepts the argument that the pathogenic mechanism is a major factor determining clinical and pathological phenotypes, one may assume that the pathogenic mechanism of a subtype of sporadic PD would be similar to that of familial PD with similar clinical and pathological phenotypes. It has been thought that heterogeneity in sporadic idiopathic PD may suggest the existence of subgroups within the disorder, with distinct clinical patterns and perhaps different pathogenic mechanisms.26 However, without a specific biologic marker or a test, the subtyping of sporadic PD has been entirely dependant on clinical features: specific motor symptoms (tremor predominant vs. postural instability and gait difficulty, PIGD), age at onset (young-onset vs. old-onset), accompanying dementia, or data-driven approach without a priori assumption.27,28 Fifteen years ago, Graham et al reported interesting findings in their data-driven approach, which are relevant to the current observations in familial PD. They could identity 3 distinctive subtypes in sporadic PD: the "motor only", "motor and cognitive", and "rapid progression".27 The "motor only" subtype could correspond to benign YOPD in most recessive parkinsonism, whereas the "motor and cognitive" and the "rapid progression" could correspond to Park8 and Park1 (with duplication or triplication) respectively. This example demonstrates that a parallelism may indeed exist between sporadic and familial PD with respect to the disease phenotype. Recently, new application of PET and SPECT for exploring biochemical and pathological aspects of disease,29-31 which will be discussed in the following sections, makes it possible to test this hypothesis by providing necessary biomarkers for sporadic PD (Fig. 2).
In recent years, PET and SPECT have been increasingly used for studies on familial PD. In 2001, Hilker et al in Germany reported a [18F]-dopa PET study in 5 symptomatic and 5 asymptomatic family members with parkin mutation.32 No significant differences were found between familial and sporadic PD. Interestingly, asymptomatic carriers with heterozygous mutations showed mild but significant reduction of Ki (by about 18%) without a rostrocaudal gradient. In the following year, UK Hammersmith group reported two serial observations over up to 10 years: 4 symptomatic parkin patients showed slower progression than sporadic PD, whereas 4 asymptomatic parkin carriers (2 had serial scans) showed mild reduction of Ki but without significant progression.33 In 2003, a French study reported no significant differences in [18F]-dopa findings between 19 parkin patients and 6 parkin-negative, sporadic YOPD.34 In 2005, the UK Hammersmith group confirmed their earlier observations in asymptomatic parkin heterozygotes, showing mild reduction of Ki without anteroposterior gradient in the striatum.35
[18F]-dopa PET study for patients with PINK1 mutations also showed significant reduction of [18F]-dopa Ki in the striatum, more so in the putamen.36 As in parkin mutations, asymptomatic carriers with heterozygous PINK1 mutation showed mild reduction of Ki without significant anteroposterier gradient. Recent findings of [123I]-β-CIT SPECT in a patient with PINK1 homozygous mutation was similar to those of [18F]-dopa PET.37 Recent [18F]-FP-CIT PET study in a Turkish family with DJ-1 mutations showed significant reduction of [18F]-FP-CIT binding in the striatum with significant rostrocaudal gradient in a patient with homozygous mutation, but mild reduction only, without significant rostrocaudal gradient in asymptomatic sister with homozygous mutation.38
Mutations in the LRRK2 gene cause autosomal dominant parkinsonism that is clinically and pathologically indistinguishable from typical late-onset, sporadic idiopathic PD.7,39 Moreover, the disease penetrance in LRRK2 carriers is age-dependent, increasing from 17% at age 50 years to 85% at age 70 years,40 simulating the age-dependent increase of sporadic idiopathic PD. [18F]-dopa PET in two patients with LRRK2 mutation did not show significant differences from idiopathic PD.41 The collaborative study between people in Vancouver and Mayo Jacksonville, using three presynaptic tracers of [18F]-dopa, [11C]-methylphenidate (MP, for DA transporter) and [11C]-dihydrotetrabenazine (DTBZ, for VMAT2 in the synaptic vesicle of monoaminergic terminals), showed similar findings between 4 symptomatic patients with LRRK2 mutation and sporadic idiopathic PD. Two asymptomatic carriers showed mild reduction of [11C]-DTBZ and [11C]-MP binding, but not [18F]-dopa Ki, suggestive of possible compensatory changes in the preclinical stage.42 [123I]-ioflupane SPECT study in 10 Italian patients with LRRK2 mutation (7 familial, 3 sporadic) also did not show significant differences between PD with LRRK2 mutation and without mutation.43
Functional imaging in familial PD has been conducted using only dopamine tracers. Heterogeneity of familial and sporadic PD is, however, largely accounted for by non-dopamine and Alzheimer pathology; thus, PET or SPECT with multiple tracers can explore the extent of chemical or morphological pathology in the brain. There are three potentially useful tracers, which are in line with our interests.
The first tracer is a cholinergic ligand. Dementia is the most important variable in the categorization of PD, and attributed to multiple mechanisms including cortical cholinergic deficits,44-46 Alzheimer pathology and cortical Lewy pathology in addition to dopamine or other monoamine deficits. 11C-labeled acetylcholine analog is a presynaptic marker, measuring the activity of acetylcholine esterase in the cortex (which reflects cholinergic terminal density). There are two types: [11C]-MP4A (methylpiperidin-4-yl acetate) developed in Japan, and [11C]-PMP (methyl-piperidin-4-yl propionate) developed in Ann Arbor, USA. Both have similar biological characteristics. PET studies using one of these ligands have been reported for patients with Alzheimer disease (AD),47-49 PD and PSP,50 PD with dementia,51 and subjects with MCI.52 These ligands are affected by acetylcholine esterase inhibitors, such as donepezil, which can be a problem for patients on this drug.53 However, [123I]-IBVM (iodobenzovesamicol), SPECT tracer, binds to acetylcholine vesicular transporter;54 thus, is not likely affected by acetylcholine esterase inhibitors. A SPECT study using this tracer in AD and PD patients was reported in 1996.54
The next tracer of interest is [11C]-OH-BTA-1, commonly called as Pittsburgh Compound-B (PIB). This PET tracer is a derivative of thioflavin-T, which is amyloid-binding histological dye.55 In 2004, Klunk et al in Pittsburgh reported marked [11C]-PIB binding in the association cortex of AD patients, which inversely correlated with [18F]-FDG (fluorodeoxyglucose) uptake.29 Postmortem pathological studies have reported that Alzheimer pathology is common in most, but not all,56 PD patients with dementia.57,58 The natural history of Alzheimer pathology in relation to Lewy pathology is not known.59 At present, [11C]-PIB PET studies for PD with dementia and dementia with Lewy bodies are being conducted in some centers. SPECT tracer for amyloid plaque is currently under development.
The third tracer of interest is [11C]-PK11195, a PET ligand originally developed for the peripheral benzodiazepine binding site (PBBS).60 Since PBBS expresses in activated microglia, it began to be used for neurodegenerative disorders after McGeer reported abundant activated microglia in the PD and AD brains.61,62 This tracer is potentially important because microglial response may be used as a marker for disease activity in PD, which is otherwise not available. Therefore, in principle, it may localize the area of brain where the disease process is active, and also may show the time course of disease activity in a given region - findings which would be highly meaningful for the study of putative neuroprotective agents. In 2005, Ouchi et al first reported a study in PD patients, using two tracers of [11C]-(R)-PK11195 and [11C]-CFT (carbomethoxy-3 beta-(4-fluorophenyl) tropane; DAT ligand).30 They found increased binding of [11C]-(R)-PK11195 in the PD patients, particularly in the midbrain, which correlated inversely with [11C]-CFT binding in the putamen. In the following year, the UK Hammersmith group reported a longitudinal [11C]-(R)-PK11195 PET study in PD patients, which did not show significant changes over 2 years.63
In summary, with a variety of tracers that can provide biochemical and molecular aspects of pathology, we are now in a position to explore longitudinal changes of pathology in PD patients. The "functional" imaging is evolving into the "pathological" imaging; thus, for the first time since James Parkinson's clinical report, it becomes a reality to combine the two major expressions of disease, i.e. clinical and pathological phenotypes, in real time.
Throughout the history, advances in scientific technology have led to new discoveries and innovative treatments in medicine. With a rapid development of computed imaging device and radiopharmaceuticals, we are now facing a new opportunity for PD, almost 200 years after first reported. Functional imaging will make it possible to categorize PD into subtypes with distinct pathogenesis. It will be also instrumental for clinicogenetic studies identifying susceptibility factors and at-risk groups, which are essential for successful neuroprotective treatment when it becomes available.
ACKNOWLEDGMENT
CSL is a Senior Scholar supported by Michael Smith Foundation for Health Research. This work is supported in part by the Desjardins family fund.
References
1. Polymeropoulos MH, Lavedan C, Leroy E, Ide SE, Dehejia A, Dutra A, et al. Mutation in the alpha-synuclein gene identified in families with Parkinson's disease. Science. 1997; 276:2045–2047. PMID: 9197268.
2. Spillantini MG, Schmidt ML, Lee VM, Trojanowski JQ, Jakes R, Goedert M. Alpha-synuclein in Lewy bodies. Nature. 1997; 388:839–840. PMID: 9278044.
3. Gibb WR, Lees AJ. The relevance of the Lewy body to the pathogenesis of idiopathic Parkinson's disease. J Neurol Neurosurg Psychiatry. 1988; 51:745–752. PMID: 2841426.


4. Hughes AJ, Daniel SE, Blankson S, Lees AJ. A clinicopathologic study of 100 cases of Parkinson's disease. Arch Neurol. 1993; 50:140–148. PMID: 8431132.


5. Farrer MJ. Genetics of Parkinson disease: paradigm shifts and future prospects. Nat Rev Genet. 2006; 7:306–318. PMID: 16543934.


6. Wszolek ZK, Pfeiffer RF, Tsuboi Y, Uitti RJ, McComb RD, Stoessl AJ, et al. Autosomal dominant parkinsonism associated with variable synuclein and tau pathology. Neurology. 2004; 62:1619–1622. PMID: 15136696.


7. Zimprich A, Biskup S, Leitner P, Lichtner P, Farrer M, Lincoln S, et al. Mutations in LRRK2 cause autosomal-dominant parkinsonism with pleomorphic pathology. Neuron. 2004; 44:601–607. PMID: 15541309.


8. Pramstaller PP, Schlossmacher MG, Jacques TS, Scaravilli F, Eskelson C, Pepivani I, et al. Lewy body Parkinson's disease in a large pedigree with 77 Parkin mutation carriers. Ann Neurol. 2005; 58:411–422. PMID: 16130111.
9. Hughes AJ, Daniel SE, Kilford L, Lees AJ. Accuracy of clinical diagnosis of idiopathic Parkinson's disease: a clinico-pathological study of 100 cases. J Neurol Neurosurg Psychiatry. 1992; 55:181–184. PMID: 1564476.


10. Rajput AH, Rozdilsky B, Rajput A. Accuracy of clinical diagnosis in parkinsonism--a prospective study. Can J Neurol Sci. 1991; 18:275–278. PMID: 1913360.
11. Singleton A, Gwinn-Hardy K. Parkinson's disease and dementia with Lewy bodies: a difference in dose? Lancet. 2004; 364:1105–1107. PMID: 15451205.


12. Ozelius LJ, Senthil G, Saunders-Pullman R, Ohmann E, Deligtisch A, Tagliati M, et al. LRRK2 G2019S as a cause of Parkinson's disease in Ashkenazi Jews. N Engl J Med. 2006; 354:424–425. PMID: 16436782.
13. Lesage S, Durr A, Tazir M, Lohmann E, Leutenegger AL, Janin S, et al. LRRK2 G2019S as a cause of Parkinson's disease in North African Arabs. N Engl J Med. 2006; 354:422–423. PMID: 16436781.
14. Tomiyama H, Li Y, Funayama M, Hasegawa K, Yoshino H, Kubo SI, et al. Clinicogenetic study of mutations in LRRK2 exon 41 in Parkinson's disease patients from 18 countries. Mov Disord. 2006; 21:1102–1108. Apr 18 [Epub]. PMID: 16622854.
15. Gosal D, Ross OA, Wiley J, Irvine GB, Johnston JA, Toft M, et al. Clinical traits of LRRK2-associated Parkinson's disease in Ireland: a link between familial and idiopathic PD. Parkinsonism Relat Disord. 2005; 11:349–352. PMID: 16102999.


16. Fishman PS, Oyler GA. Significance of the parkin gene and protein in understanding Parkinson's disease. Curr Neurol Neurosci Rep. 2002; 2:296–302. PMID: 12044248.


17. Calne DB. Is "Parkinson's disease" one disease? J Neurol Neurosurg Psychiatry. 1989; 2(Suppl):18–21. PMID: 2666575.


18. Fahn S. Description of Parkinson's disease as a clinical syndrome. Ann N Y Acad Sci. 2003; 991:1–14. PMID: 12846969.


19. Klein C. Implications of genetics on the diagnosis and care of patients with Parkinson disease. Arch Neurol. 2006; 63:328–334. PMID: 16533959.


20. Golbe LI, Di Iorio G, Bonavita V, Miller DC, Duvoisin RC. A large kindred with autosomal dominant Parkinson's disease. Ann Neurol. 1990; 27:276–282. PMID: 2158268.


21. Welch K, Yuan J. Alpha-synuclein oligomerization: a role for lipids? Trends Neurosci. 2003; 26:517–519. PMID: 14522142.
22. West AB, Moore DJ, Biskup S, Bugayenko A, Smith WW, Ross CA, et al. Parkinson's disease-associated mutations in leucine-rich repeat kinase 2 augment kinase activity. Proc Natl Acad Sci U S A. 2005; 102:16842–16847. PMID: 16269541.
23. Gloeckner CJ, Kinkl N, Schumacher A, Braun RJ, O'Neill E, Meitinger T, et al. The Parkinson disease causing LRRK2 mutation I2020T is associated with increased kinase activity. Hum Mol Genet. 2006; 15:223–232. PMID: 16321986.


24. Shen J, Cookson MR. Mitochondria and dopamine: new insights into recessive parkinsonism. Neuron. 2004; 43:301–304. PMID: 15294138.
25. Cookson MR. The biochemistry of Parkinson's disease. Annu Rev Biochem. 2005; 74:29–52. PMID: 15952880.


26. Jankovic J, McDermott M, Carter J, Gauthier S, Goetz C, Golbe L, et al. Variable expression of Parkinson's disease: a base-line analysis of the DATATOP cohort. The Parkinson Study Group. Neurology. 1990; 40:1529–1534. PMID: 2215943.
27. Graham JM, Sagar HJ. A data-driven approach to the study of heterogeneity in idiopathic Parkinson's disease: identification of three distinct subtypes. Mov Disord. 1999; 14:10–20. PMID: 9918339.
28. Lewis SJ, Foltynie T, Blackwell AD, Robbins TW, Owen AM, Barker RA. Heterogeneity of Parkinson's disease in the early clinical stages using a data driven approach. J Neurol Neurosurg Psychiatry. 2005; 76:343–348. PMID: 15716523.


29. Klunk WE, Engler H, Nordberg A, Wang Y, Blomqvist G, Holt DP, et al. Imaging brain amyloid in Alzheimer's disease with Pittsburgh Compound-B. Ann Neurol. 2004; 55:306–319. PMID: 14991808.


30. Ouchi Y, Yoshikawa E, Sekine Y, Futatsubashi M, Kanno T, Ogusu T, et al. Microglial activation and dopamine terminal loss in early Parkinson's disease. Ann Neurol. 2005; 57:168–175. PMID: 15668962.


31. Hilker R, Thomas AV, Klein JC, Weisenbach S, Kalbe E, Burghaus L, et al. Dementia in Parkinson disease: functional imaging of cholinergic and dopaminergic pathways. Neurology. 2005; 65:1716–1722. PMID: 16344512.


32. Hilker R, Klein C, Ghaemi M, Kis B, Strotmann T, Ozelius LJ, et al. Positron emission tomographic analysis of the nigrostriatal dopaminergic system in familial parkinsonism associated with mutations in the parkin gene. Ann Neurol. 2001; 49:367–376. PMID: 11261512.
33. Khan NL, Brooks DJ, Pavese N, Sweeney MG, Wood NW, Lees AJ, et al. Progression of nigrostriatal dysfunction in a parkin kindred: an [18F]dopa PET and clinical study. Brain. 2002; 125:2248–2256. PMID: 12244082.
34. Thobois S, Ribeiro MJ, Lohmann E, Durr A, Pollak P, Rascol O, et al. Young-onset Parkinson disease with and without parkin gene mutations: a fluorodopa F 18 positron emission tomography study. Arch Neurol. 2003; 60:713–718. PMID: 12756135.
35. Khan NL, Scherfler C, Graham E, Bhatia KP, Quinn N, Lees AJ, et al. Dopaminergic dysfunction in unrelated, asymptomatic carriers of a single parkin mutation. Neurology. 2005; 64:134–136. PMID: 15642918.


36. Khan NL, Valente EM, Bentivoglio AR, Wood NW, Albanese A, Brooks DJ, et al. Clinical and subclinical dopaminergic dysfunction in PARK6-linked parkinsonism: an 18F-dopa PET study. Ann Neurol. 2002; 52:849–853. PMID: 12447943.
37. Albanese A, Valente EM, Romito LM, Bellacchio E, Elia AE, Dallapiccola B. The PINK1 phenotype can be indistinguishable from idiopathic Parkinson disease. Neurology. 2005; 64:1958–1960. PMID: 15955954.


38. Hering R, Strauss KM, Tao X, Bauer A, Woitalla D, Mietz EM, et al. Novel homozygous p.E64D mutation in DJ1 in early onset Parkinson disease (PARK7). Hum Mutat. 2004; 24:321–329. PMID: 15365989.


39. Paisan-Ruiz C, Saenz A, Lopez de Munain A, Marti I, Martinez Gil A, Marti-Masso JF, et al. Familial Parkinson's disease: clinical and genetic analysis of four Basque families. Ann Neurol. 2005; 57:365–372. PMID: 15732106.
40. Kachergus J, Mata IF, Hulihan M, Taylor JP, Lincoln S, Aasly J, et al. Identification of a novel LRRK2 mutation linked to autosomal dominant parkinsonism: evidence of a common founder across European populations. Am J Hum Genet. 2005; 76:672–680. PMID: 15726496.


41. Hernandez DG, Paisan-Ruiz C, McInerney-Leo A, Jain S, Meyer-Lindenberg A, Evans EW, et al. Clinical and positron emission tomography of Parkinson's disease caused by LRRK2. Ann Neurol. 2005; 57:453–456. PMID: 15732108.
42. Adams JR, van Netten H, Schulzer M, Mak E, McKenzie J, Strongosky A, et al. PET in LRRK2 mutations: comparison to sporadic Parkinson's disease and evidence for presymptomatic compensation. Brain. 2005; 128:2777–2785. PMID: 16081470.


43. Isaias IU, Benti R, Goldwurm S, Zini M, Cilia R, Gerundini P, et al. Striatal dopamine transporter binding in Parkinson's disease associated with the LRRK2 Gly2019Ser mutation. Mov Disord. 2006; 21:1144–1147. May 2 [Epub]. PMID: 16671078.
44. Whitehouse PJ, Hedreen JC, White CL 3rd, Price DL. Basal forebrain neurons in the dementia of Parkinson disease. Ann Neurol. 1983; 13:243–248. PMID: 6847136.


45. Perry EK, Curtis M, Dick DJ, Candy JM, Atack JR, Bloxham CA, et al. Cholinergic correlates of cognitive impairment in Parkinson's disease: comparisons with Alzheimers disease. J Neurol Neurosurg Psychiatry. 1985; 48:413–421. PMID: 3998751.


46. Dubois B, Danze F, Pillon B, Cusimano G, Lhermitte F, Agid Y. Cholinergic-dependent cognitive deficits in Parkinson's disease. Ann Neurol. 1987; 22:26–30. PMID: 3631918.


47. Iyo M, Namba H, Fukushi K, Shinotoh H, Nagatsuka S, Suhara T, et al. Measurement of acetylcholinesterase by positron emission tomography in the brains of healthy controls and patients with Alzheimer's disease. Lancet. 1997; 349:1805–1809. PMID: 9269216.


48. Kuhl DE, Koeppe RA, Minoshima S, Snyder SE, Ficaro EP, Foster NL, et al. In vivo mapping of cerebral acetylcholinesterase activity in aging and Alzheimer's disease. Neurology. 1999; 52:691–699. PMID: 10078712.


49. Shinotoh H, Namba H, Fukushi K, Nagatsuka S, Tanaka N, Aotsuka A, et al. Progressive loss of cortical acetylcholinesterase activity in association with cognitive decline in Alzheimer's disease: a positron emission tomography study. Ann Neurol. 2000; 48:194–200. PMID: 10939570.


50. Shinotoh H, Namba H, Yamaguchi M, Fukushi K, Nagatsuka S, Iyo M, et al. Positron emission tomographic measurement of acetylcholinesterase activity reveals differential loss of ascending cholinergic systems in Parkinson's disease and progressive supranuclear palsy. Ann Neurol. 1999; 46:62–69. PMID: 10401781.


51. Bohnen NI, Kaufer DI, Ivanco LS, Lopresti B, Koeppe RA, Davis JG, et al. Cortical cholinergic function is more severely affected in parkinsonian dementia than in Alzheimer disease: an in vivo positron emission tomographic study. Arch Neurol. 2003; 60:1745–1748. PMID: 14676050.
52. Herholz K, Weisenbach S, Kalbe E, Diederich NJ, Heiss WD. Cerebral acetylcholine esterase activity in mild cognitive impairment. Neuroreport. 2005; 16:1431–1434. PMID: 16110265.


53. Shinotoh H, Aotsuka A, Fukushi K, Nagatsuka S, Tanaka N, Ota T, et al. Effect of donepezil on brain acetylcholinesterase activity in patients with AD measured by PET. Neurology. 2001; 56:408–410. PMID: 11171913.


54. Kuhl DE, Minoshima S, Fessler JA, Frey KA, Foster NL, Ficaro EP, et al. In vivo mapping of cholinergic terminals in normal aging, Alzheimer's disease, and Parkinson's disease. Ann Neurol. 1996; 40:399–410. PMID: 8797529.


55. Klunk WE, Wang Y, Huang GF, Debnath ML, Holt DP, Mathis CA. Uncharged thioflavin-T derivatives bind to amyloid-beta protein with high affinity and readily enter the brain. Life Sci. 2001; 69:1471–1484. PMID: 11554609.


56. Heilig CW, Knopman DS, Mastri AR, Frey W 2nd. Dementia without Alzheimer pathology. Neurology. 1985; 35:762–765. PMID: 2986048.


57. Boller F, Mizutani T, Roessmann U, Gambetti P. Parkinson disease, dementia, and Alzheimer disease: clinicopathological correlations. Ann Neurol. 1980; 7:329–335. PMID: 7377758.


58. Jellinger K. Alzheimer pathology in Parkinson's disease. Neurology. 1989; 39:874–875. PMID: 2725894.


59. Jellinger KA, Seppi K, Wenning GK, Poewe W. Impact of coexistent Alzheimer pathology on the natural history of Parkinson's disease. J Neural Transm. 2002; 109:329–339. PMID: 11956955.


60. Charbonneau P, Syrota A, Crouzel C, Valois JM, Prenant C, Crouzel M. Peripheral-type benzodiazepine receptors in the living heart characterized by positron emission tomography. Circulation. 1986; 73:476–483. PMID: 3004781.


61. McGeer PL, Itagaki S, Akiyama H, McGeer EG. Rate of cell death in parkinsonism indicates active neuropathological process. Ann Neurol. 1988; 24:574–576. PMID: 3239957.


62. McGeer PL, McGeer EG. The inflammatory response system of brain: implications for therapy of Alzheimer and other neurodegenerative diseases. Brain Res Brain Res Rev. 1995; 21:195–218. PMID: 8866675.


63. Gerhard A, Pavese N, Hotton G, Turkheimer F, Es M, Hammers A, et al. In vivo imaging of microglial activation with [11C](R)-PK11195 PET in idiopathic Parkinson's disease. Neurobiol Dis. 2006; 21:404–412. PMID: 16182554.
64. Bonifati V, Rizzu P, van Baren MJ, Schaap O, Breedveld GJ, Krieger E, et al. Mutations in the DJ-1 gene associated with autosomal recessive early-onset parkinsonism. Science. 2003; 299:256–259. PMID: 12446870.
65. Canet-Aviles RM, Wilson MA, Miller DW, Ahmad R, McLendon C, Bandyopadhyay S, et al. The Parkinson's disease protein DJ-1 is neuroprotective due to cysteine-sulfinic acid-driven mitochondrial localization. Proc Natl Acad Sci U S A. 2004; 101:9103–9108. PMID: 15181200.
66. Lee VM, Goedert M, Trojanowski JQ. Neurodegenerative tauopathies. Annu Rev Neurosci. 2001; 24:1121–1159. PMID: 11520930.


Figure 1
In Parkinson's disease (PD), pathological and clinical features are two major phenotypes, largely determined by pathogenic mechanisms. Pathological phenotype includes the molecular type (e.g. α-synuclein, or tauopathy) and topographical distribution. Clinical phenotype includes the time course (e.g. age at onset, the rate of progression) and symptomatology (e.g. parkinsonism, dementia, or autonomic dysfunction). (1) Molecular pathology reflects underlying pathogenic mechanisms.25 (2) Regional selectivity of pathology is derived from selective vulnerability of affected neurons to the pathogenic process.66 (3) Temporal course of PD also reflects underlying pathogenic mechanisms. For example, mutations in parkin, DJ-1 or PINK1 are associated with young-onset PD with slow progression. Park1 shows a gene-dose effect, more aggressive with greater multiplication (see the text). (4) Symptomatology is determined by the location of pathology. For instance, nigral tauopathy produces parkinsonism, indistinguishable from Lewy body PD.10
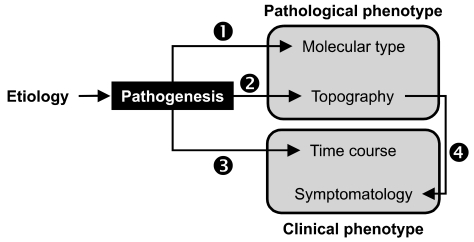
Figure 2
Stepped approach to explore the pathogenesis of sporadic PD. The basic tenet underlying this approach is that subgroups of familial and sporadic PD with similar clinical and pathological phenotypes are likely to share pathogenic mechanisms. (1) Define the spectrum of clinical phenotype, findings of pathological imaging, and genotype in familial monogenic PD. (2) Identify a cluster of sporadic PD, whose clinical phenotype and pathological imaging findings are similar to those of a subtype of familial PD. (3) Epidemiological and clinico-genetic studies are conducted in this relatively homogeneous subgroup of sporadic PD to identify genetic susceptibility factors and gene-environmental interactions.
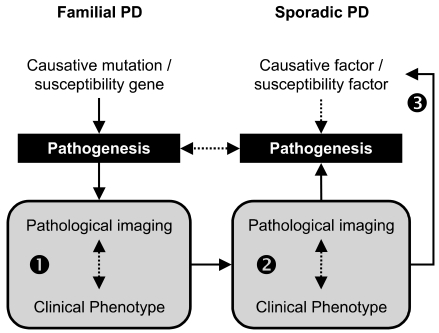