Abstract
Praxis, the ability to perform skilled or learned movements is essential for daily living. Inability to perform such praxis movements is defined as apraxia. Apraxia can be further classified into subtypes such as ideomotor, ideational and limb-kinetic apraxia. Relevant brain regions have been found to include the motor, premotor, temporal and parietal cortices. Apraxia is found in a variety of highly prevalent neurological disorders including dementia, stroke and Parkinsonism. Furthermore, apraxia has been shown to negatively affect quality of life. Therefore, recognition and treatment of this disorder is critical. This article provides an overview of apraxia and highlights studies dealing with the neurophysiology of this disorder, opening up novel perspectives for the use of motor training and noninvasive brain stimulation as treatment.
A typical daily life involves carrying out a variety of movements. Movements can be divided into those requiring use of tools and those that do not.1 Food preparation and housework are examples of the former, often requiring the use of kitchen tools and home appliances. Dressing, cleansing and grooming require multi-step sequential actions and at times, fine movements of the upper limb. Needless to say, the ability to perform such actions essential to daily living is integral to one's functional independence.
Praxis is defined as the ability to perform such skilled or learned movements. Apraxia refers to the inability to carry out such praxis movements in the absence of elementary motor, sensory or coordination deficits that could serve as the primary cause. Apraxia can be further classified into subtypes such as ideomotor, ideational and limb-kinetic apraxia. This phenomenon is seen in a variety of neurological disorders such as dementia, stroke and Parkinsonism. Prior to the advance in neurophysiology, knowledge on praxis or apraxia relied on clinical observations of human subjects. With recent advances in applications of these methods including noninvasive brain stimulation, knowledge on the subject of apraxia has expanded. Such insight may very likely open up possibilities for the use of neuromodulation as a way to treat this disorder, in conjunction with motor training that has also been attempted as treatment. I aim to provide an overview of apraxia with an emphasis on the neurophysiology involving various brain regions that appear to contribute to human action.
Much of the conceptual knowledge of apraxia was established by Hugo Liepmann, a pioneer in the field of cognitive neurology who described this phenomenon in a stroke patient after studying his manual gestures. Liepmann studied a 48-year-old man who had sustained a left hemispheric stroke and found that he was unable to button a shirt or light a cigar, even after the paresis had largely resolved. However, Liepmann noted that the patient was able to carry out spontaneous movements such using a spoon while eating, perform simple gestures on command, or pantomime. He also made the observation that patients with left hemispheric lesions but not those with right hemispheric lesions were unable to perform praxis movements. Along with the observations made in patients with lesions of the corpus callosum, Liepmann concluded that “motor planning” occurred in the motor area of the left hemisphere. He proposed a model of information, specifically a movement formula, flowing anteriorly from posterior brain regions, i.e., the occipital and parietal lobes, to the motor cortex (Fig. 1).2
Norman Geschwind,34 a behavioral neurologist, also presented a model for apraxia, which was based on the disconnection of the left premotor cortex and Wernicke's area. He proposed that the disruption occurred due to involvement of the superior longitudinal fasciculus connecting these two areas. Other authors have presented similar models of praxis as well.5 More recently, Buxbaum proposed a model involving the left frontal and inferior parietal lobules, where the representations of object identity reside in the ventral stream and spatial body representations in the dorsal system.67
Liepmann further classified apraxia into the following major subtypes; ideomotor, ideational and limb-kinetic apraxia. Apraxias have also been viewed with regard to task-specificity; task-specific apraxias such as dressing apraxia, sitting apraxia, apraxia of eyelid opening and apraxia of gait have been noted.8 These task-specific apraxic disorders will be mentioned briefly below.
Ideomotor apraxia is a subtype of apraxia that is commonly seen in patients with stroke or neurodegenerative disorders. It is defined as a disorder of gesture performance upon verbal command, despite having intact knowledge of tasks. For example, the patient might be able to describe how to use a spoon, but not able to demonstrate the actual use. This typically results in the patient failing to pantomime a transitive act (“Show me how you would use a screwdriver”). Ideational apraxia, on the other hand, is characterized by inability to conceptualize a task, despite intact identification of the tools. When presented with a stamp and an envelope, one might be able to name the objects, but unable to demonstrate how to mail an envelope using those objects. In this situation, the examiner finds that the patient is unable to correctly sequence a series of actions required in a specific type of activity. Limb-kinetic apraxia is another major subtype of apraxia that indicates the loss of the ability to make precise, independent but coordinated finger and hand movements, resulting in inaccurate or clumsy movements.9 Examples of tasks requiring fine motor performance including buttoning or coin rotation, the latter of which has been proposed as a test of motor dexterity.10
Examples of task-specific apraxia include dressing or sitting apraxia, apraxia of eyelid opening and gait apraxia.1112 Apraxia of eyelid opening and apraxia of gait are now more strictly considered to be disorders misclassified as apraxia.13 Apraxia of eyelid opening is characterized as difficulty with voluntary eye opening. One can test for this by having the patient voluntarily close their eyes, and open instantaneously when the examiner shouts “open!” The patient with apraxia of eyelid opening will not be able to open the eyes immediately. This disorder can be misdiagnosed as focal eye dystonia or blepharospasm, conditions where there is forceful eye closure. Apraxia of gait is a somewhat nonspecific term often used to refer to various gait patterns, including gait ignition failure and freezing of gait. The pathophysiology of these disorders is not well understood, although some studies have found brain regions relevant in other types of apraxia to be involved in apraxia of eyelid opening, suggesting that these disorders may share common neural systems.
Apraxia is seen in various neurological disorders, including stroke and neurodegenerative disorders such as Alzheimer's disease, Parkinson's disease and atypical Parkinsonism [common examples are corticobasal syndrome (CBS); previously termed corticobasal degeneration, or progressive supranuclear palsy (PSP)].113 In patients with left hemispheric stroke, apraxia has been reported to be prevalent in approximately one-third of this population.14 In clinical practice, it is not uncommon that more than one type of apraxia is present in a single affected patient.15
Despite the relatively straightforward recognition of apraxia, there is no widely-used, standardized method to evaluate for this phenomenon. To properly identify apraxia, both transitive (movements involving tools) and intransitive (communicative or gestural) movements should be used for evaluation. When errors and problems with accuracy occur, the examiner must carefully analyze the difficulties. Does the patient have intact knowledge of tools and objects in terms of their function? Are there problems with focal or distal limb control? Is the patient able to carry out the correct sequence of actions required for the intended movement? “Body part apraxia” can also be seen in apraxic patients when they are asked to pantomime transitive movements, such as brushing their hair. In this case, the patient will use their hand as a brush itself, and comb their fingers through their hair. This has been presumed to result from a loss of internal representation of tools, and relatively intact intransitive representations.16 Other types of error can occur as well, such as additions, deletions, perseverations, substitutions and lack of a response. Testing of apraxia should be done in both upper limbs, as both can be affected.15 Testing for apraxia in the lower limbs and oro-buccal muscles can be revealing as well.1718 The test of oral and limb apraxia (TOLA) is one suitable for evaluation of apraxia affecting those body regions, but has limitations in terms of ignoring performance when seeing or using tools.15
Several testing methods for apraxia have been developed to date, most of which are lacking in validity and sensitivity. Most scales are not quick to use and therefore not commonly applied in the clinical setting. The De Renzi ideomotor apraxia test is a 24-item scale tested in patients with left or right brain damage, developed for the assessment of ideomotor apraxia.19 More recently, a comprehensive test termed the test of upper limb apraxia (TULIA) was developed, which includes non-symbolic (meaningless), intransitive (communicative) and transitive (tool-related) gestures.20 This 48-item test was found to have good reliability and validity, and a more concise, bedside 12-item test based on TULIA was further developed (Apraxia Screen of TULIA; AST), shown to have high specificity and sensitivity (Refer to Appendix 2 of http://jnnp.bmj.com/content/82/4/389.long).
Such bedside tests may allow for a quick and reliable apraxia assessment, which may be clinically applicable and therefore useful for assessing the severity of ideomotor apraxia. These apraxia tests include tasks involving imitation, which can be disturbed in either left or right brain damage. In left brain damage, imitation of hand postures is often disturbed, whereas imitation of finger or foot postures is disturbed in those with right brain damage.18 There is still a need for tests that test other elements of praxis, such as the actual use of tool and selection of the appropriate task for a given tool, particularly when having more than one to choose from (for example, a knife to cut bread can also be used to stab).
Standardized testing methods for limb-kinetic apraxia are lacking, in comparison to ideomotor apraxia. In addition to having the subject perform buttoning or coin-rotation tasks, limb-kinetic apraxia can be assessed by having one perform a pegboard test. This test is sometimes used in neuropsychological testing, and involves lifting one peg at a time, placing it in a hole, and moving on to the next peg, repeating this action.8
Movement types can be largely classified into transitive movements (i.e., those requiring tools), and intransitive movements which are gestural, which can further be divided into meaningful and meaningless movements. Meaningful movements are those that are communicative, such as waving goodbye, whereas meaningless movements are those that are not representational, such as touching one's chin. Patients are often more impaired in the performance of transitive movements, than intransitive ones.2122 As a result, the majority of efforts to probe the neural basis of praxis has been conducted in subjects pantomiming and using tools.
Much of today's fundamental knowledge on apraxia is based on classic observations made in clinical practice. As apraxia is present in patients with chronic left hemispheric strokes and Alzheimer's disease, these patient populations are often targets of study with regard to this phenomenon.23242526 Some patients with lesions involving the corpus callosum such as those following a callosotomy, have been noted to be apraxic with regard to the left hand, but not the right. These observations indicate that the left hemisphere is relevant for praxis, and that it may be due to retrieval of representations stored in this hemisphere.15 Patients may also be differentially affected depending on the type of neurological disorder. For example, patients with left hemispheric stroke have been noted to have more difficulties with pantomiming and transitive movements, and less with imitating gestures and intransitive movements. Other studies report that whereas patients with left brain damage appear to have difficulties with tool use but intact knowledge regarding function and gestures, patients with Alzheimer's disease show deficits in function and gestures but preserved tool use.262728
Praxis movements are complex, involving different aspects of cognition and movement; consequently, several brain regions are considered to be relevant. The conceptual knowledge for actions appears to involve brain regions such as the left premotor, prefrontal, middle temporal and parietal areas.29 Neuroimaging studies such as those using positron emission tomography (PET) and functional magnetic resonance imaging (fMRI) have probed the neural correlates of praxis, with studies widely varying in which aspect of praxis to focus on. Functional neuroimaging studies have shown that the left temporal lobe is involved in retrieval of semantic memory that plays a role in praxis.303132 Evidence also suggests that the left premotor cortex is also involved in retrieval of knowledge regarding tools and their actions.333435 The left inferior and/or superior parietal lobule have also been shown to be involved in grasping movements aimed at tools.30363738 The parietal cortex appears to be relevant in the integration of perceptual spatiotemporal information as well, such as estimating whether two objects of different sizes may collide with each other, or selecting which grasp to use when a particular object can be used in various ways (e.g., knife to stab, or slice bread).3739 Functional imaging studies suggest that there is a stronger left lateralization in posterior parietal and premotor cortices for planning intransitive movements.334041
Anatomical studies have further shown interconnections between these praxis-relevant brain regions, such as connections among the supramarginal gyrus with the ventral premotor cortex, inferior frontal gyrus, middle temporal gyrus and intraparietal areas.4243 Moreover, electroencephalography (EEG) studies have confirmed parietal and sensorimotor activity to occur prior to the onset of praxis movements, in contrast with premotor and sensorimotor activity seen prior to simple movements.4445
Reports on the location of brain lesions in specific subtypes of apraxia are available, allowing one to ponder on the underlying pathophysiology of various major subtypes of apraxia. For example, ideomotor apraxia has been found to occur in left hemispheric stroke patients with injury to the premotor cortex, supplementary motor cortex, inferior parietal lobe or corpus callosum. Relevant brain regions for ideational apraxia, a disorder characterized by difficulty with conceptualization and sequencing deficits, are likely comprised of the left premotor, prefrontal, middle temporal and parietal areas.29 Investigations on patients with limb-kinetic apraxia have found that brain region relevant to grasp, chiefly, the contralateral premotor cortex, is affected.46 Studies on healthy human subjects performing precision grip also complement these findings, supporting the theoretical notion that the contralateral premotor cortex, specifically, the ventral portion, is relevant in the pathophysiology of limb-kinetic apraxia.4748 In essence, the findings obtained from functional, anatomical and neurophysiological studies collectively suggest that the parieto-premotor-frontal network appears to be critical for both preparation and the execution of praxis movements (Fig. 2).49
Investigation of praxis-relevant brain areas has also been conducted using noninvasive brain stimulation. Disruption of neural activity using repetitive transcranial magnetic stimulation (rTMS) has been found to alter semantic knowledge, an essential component of praxis. In healthy human subjects, low-frequency rTMS (1 Hz for 10 minutes at 120% motor threshold) delivered to the anterior temporal lobe resulted in a category-general impairment (slowed performance for picture naming in both living and nonliving objects), whereas inferior parietal lobule stimulation induced a category-specific deficit for objects (slowed performance for nonliving objects only).50 Therefore, these study results support that brain regions are differentially involved in praxis, and are likely relevant in patients with apraxia who are selectively impaired with regard to category-specific or category-general knowledge. Such patients with sematic impairment have been identified in those with mild cognitive impairment or Alzheimer's disease.51525354
Apraxia has also been identified in movement disorders where the basal ganglia are involved such as Parkinson's disease, CBS and PSP.917555657 Even in the early stages of Parkinson's disease, difficulty with praxis has been identified, which appear to correlate with an activation shift from left parietal to left frontal areas shown on fMRI.58 The limb-kinetic apraxia found in patients with Parkinson's disease appears to be largely independent from bradykinesia or other symptoms, once again indicating that the problem appears to be one concerning dexterity.9 These findings imply that the basal ganglia also play a role in praxis, and that it is not only cortical structures that are involved in this process. CBS and PSP, both types of atypical Parkinsonism, are tauopathies, with pathological cells present mainly in the cortex in CBS and subcortically in PSP.55 In CBS, ideomotor and/or limb-kinetic apraxia have been reported, whereas ideomotor apraxia has been identified in PSP.555659 These reports support the theoretical notion that both cortical and subcortical brain regions play a role in praxis.
To date, there is no standardized treatment for apraxia that is available. Therapeutic efforts have been mostly concentrated in affected stroke patients with or without concomitant aphasia, as the relative frequency of limb apraxia has been reported to be approximately 51% in patients with left hemispheric stroke.6061 In these studies, rehabilitative treatment for apraxia was conducted three times weekly, each lasting 50 minutes and conducted over 30 sessions.60 Treatment for apraxia consisted of a behavioral training program comprised of gesture-production exercises, made up of three sections dedicated to the treatment of gesture with or without symbolic value and related or nonrelated to the use of objects.61 Patients who received treatment for apraxia were found to improve in both praxis and activities of daily living (ADL), compared to patients who received conventional treatment for aphasia. Training comprised of 24 communicative gestures was also used in patients with left hemispheric stroke with severe aphasia, resulting in substantial improvement of practiced gestures, and some improvement of unpracticed gestures.62 However, based on these reports, the sustainability of such improvement is unclear. Therefore, although rehabilitative training involving practical gestures may be useful in the treatment of patients with apraxia, training alone is likely insufficient for sustained benefit.
Noninvasive brain stimulation may be a useful technique to use in combination with rehabilitative training to tackle this difficult disorder. A variety of stimulation methods have been employed for investigational and therapeutic purposes in the field of neurology. Transcranial direct current stimulation (tDCS), single-pulse or rTMS, theta-burst stimulation (TBS) and paired associative stimulation (PAS) are some examples.63646566 Depending on different stimulation settings, these methods are presumed to work by exerting excitatory or inhibitory influences on cortical plasticity and excitability.67 For example, rTMS can be delivered in either high (≥5 Hz) frequency or low (0.2–1 Hz) frequency, the former of which is considered to work in an excitatory mode and the latter, inhibitory. TBS is also a magnetic stimulation method that has recently gained much interest, as it involves a shorter stimulation period while maintaining equal efficacy.65 tDCS also influences plasticity and cortical excitability, via delivery of a low-level continuous electric current.64 Anodal and cathodal tDCS are thought to work in excitatory and inhibitory ways, respectively. PAS is also used to study and alter cortical plasticity; long-term potentiation (LTP) and long-term depression (LTD), important physiological mechanisms underlying memory, can be achieved using this stimulation technique.6566 By altering cortical plasticity using noninvasive brain stimulation prior to the conduction of rehabilitative training, this synergistic approach may result in increased efficacy and sustainability of treatment effects.
Noninvasive brain stimulation methods have been widely used for treatment of various neurological disorders, but data are scarce on the impact of brain stimulation for cognitive disorders. rTMS has been attempted for the treatment of Alzheimer's disease, with a few studies reporting improvement of cognitive function with daily sessions of high-frequency rTMS, but none with low-frequency rTMS.686970 To date, there are no reports on the effects of rTMS on apraxia. There are however, some studies using tDCS in CBS or left-brain-damaged patients with apraxia.7172 Apraxia is a striking feature in CBS, characterized by asymmetric onset of levodopa-resistant Parkinsonism, where ideomotor apraxia and limb-kinetic apraxia are commonly found. Short-term benefits of anodal tDCS (stimulation parameters: duration of 7 minutes, intensity of 0.08 mA) of the left parietal cortex in CBS patients (n=14, mean age=68.9 years) with ideomotor apraxia have been noted, as demonstrated by significant improvement in the De Renzi ideomotor apraxia test scores (Fig. 3).71 However, no significant improvement was found with anodal stimulation of the right parietal cortex in these patients.71 Bolognini et al.72 applied anodal tDCS (stimulation parameters: duration of 10 minutes, intensity of 2 mA) to the left posterior parietal cortex, the right motor cortex and sham stimulation, finding that with stimulation of the left posterior parietal cortex, the time required to perform skilled movements, as well as planning, was reduced in both left hemispheric stroke patients (n=6, mean age=72.16 years) and healthy subjects (n=6, mean age=66.7 years). They also made the observation that tDCS to the right motor cortex diminished execution times in both groups.
Apraxia is a higher-order disorder of sensorimotor integration, commonly seen in neurodegenerative diseases and stroke. Praxis requires multiple aspects of cognition and movement to be brought together; to name a few, appreciation of tools as well as the posture of one's body parts with relation to time and space, sequence of necessary actions, and planning of grasp. As a result, key components of praxis that one should test for include the following: performance using tools, performance in a given situation (such as waving hello), and pantomiming to verbal command and imitation.
Despite its apparent subtleness, apraxia has been noted to cause impairment of daily activities.973 Therefore, while apraxia may not always be immediately evident, it is important to test for as it has been found to have considerable impact on patients' quality of life.74 Advances in neurophysiological techniques allow investigators to probe specific brain areas and obtain more specific information, in contrast to the clinical setting where it is difficult to properly assess the function of each brain area when multiple regions are lesioned.
Evidence from the aforementioned functional brain imaging studies suggests that praxis requires for appropriate transformation of visual and somatosensory information into movements, such as posture and grasping. Semantic knowledge of objects is also an essential component in praxis. According to the findings of anatomical and functional neuroimaging studies, praxis-relevant brain regions include the left posterior parietal, temporal, premotor and motor cortices. Recent research has focused heavily on the left inferior parietal lobule, which appears to play a role in the storage of motor representations, as well as in semantic memory. Moreover, a left hemisphere parietal-premotor-motor network appears to be activated in praxis, indicating that the deficits found in apraxia are likely due to affected networks rather than a specific brain area.
Although further investigation is warranted, recent studies using noninvasive brain stimulation have confirmed the findings of anatomical and functional neuroimaging studies, once again demonstrating various brain regions to be involved in praxis. These investigations have confirmed, as well as built upon the previously established classical observations and theories of the dorsal-to-ventral stream of information underlying praxis. Future exploration using different methods of noninvasive brain stimulation are necessary to investigate whether praxis-relevant brain areas can be modulated, and possibly serve as potential targets for treatment. Furthermore, complementing the potential therapeutic effects of noninvasive brain stimulation with rehabilitative training comprised of practical gestures may be a synergistic and useful approach for the treatment of apraxia. Most importantly, with increased recognition of apraxia, proper assessment using available scales, and better ways to treat this disorder, we may be able to lessen the amount of disability these patients suffer from by helping them to ultimately gain functional independence.
Figures and Tables
Fig. 1
Reproduction of Liepmann's schema of the motor engram. Adapted from Roby-Brami et al. Philos Trans R Soc Lond B Biol Sci 2012;367:144-160, with permission of Royal Society Publishing.2 1: limb-kinetic apraxia, 2: ideomotor apraxia, 3: ideational apraxia, Co.: precentral gyrus, Cp: postcentral gyrus, F. inf.: frontal lobe, inferior, F. med.: frontal lobe, middle, F. sup.: frontal lobe, superior, G.sm.: supramarginal gyrus, O.m.: occipital lobe, medial, O.s.: occipital lobe, superior.
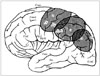
Fig. 2
Spatial plots for simple movement (A) and tool use movement (B). The beginning of the Bereitschaftspotentials (BPs), or movement-related cortical potentials, are seen to occur in bilateral sensorimotor areas in simple movement, while it is seen to begin in the left parietal area in tool pantomime. Adapted from Wheaton et al. Clinical Neurophysiology 2005;116:1382-1390, with permission of Springer.49
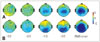
Fig. 3
Study design of tDCS in patients with corticobasal syndrome. The study was conducted in a randomized, double-blind fashion. All patients were randomly subjected to three types of stimulation over two sessions. A: tDCS of the left parietal cortex, right parietal cortex and placebo tDCS. B: The De Renzi ideomotor apraxia test was conducted to assess limb apraxia prior to and following each stimulation session. Adapted from Bianchi et al. European Journal of Neurology 2015;22: 1317-1322, with permission of Wiley.71 tDCS: transcranial direct current stimulation, PARC: parietal cortex.
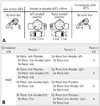
Acknowledgements
This work was supported by the National Research Foundation of the Republic of Korea (Grant #: 2017R1C1B5018).
References
2. Roby-Brami A, Hermsdörfer J, Roy AC, Jacobs S. A neuropsychological perspective on the link between language and praxis in modern humans. Philos Trans R Soc Lond B Biol Sci. 2012; 367:144–160.


3. Geschwind N. Disconnexion syndromes in animals and man. II. Brain. 1965; 88:585–644.
4. Geschwind N. Disconnexion syndromes in animals and man. I. Brain. 1965; 88:237–294.
6. Buxbaum LJ, Kyle K, Grossman M, Coslett HB. Left inferior parietal representations for skilled hand-object interactions: evidence from stroke and corticobasal degeneration. Cortex. 2007; 43:411–423.


9. Foki T, Vanbellingen T, Lungu C, Pirker W, Bohlhalter S, Nyffeler T, et al. Limb-kinetic apraxia affects activities of daily living in Parkinson's disease: a multi-center study. Eur J Neurol. 2016; 23:1301–1307.


10. Mendoza JE, Apostolos GT, Humphreys JD, Hanna-Pladdy B, O'Bryant SE. Coin rotation task (CRT): a new test of motor dexterity. Arch Clin Neuropsychol. 2009; 24:287–292.


11. Gálvez-Jiménez N, Tuite PJ. Uncommon causes of movement disorders. Cambridge: Cambridge University Press;2011.
12. Tyrrell PJ. Apraxia of gait or higher level gait disorders: review and description of two cases of progressive gait disturbance due to frontal lobe degeneration. J R Soc Med. 1994; 87:454–456.
14. Donkervoort M, Dekker J, van den Ende F, Stehmann-Saris JC, Deelman BG. Prevalence of apraxia among patients with a first left hemisphere stroke in rehabilitation centres and nursing homes. Clin Rehabil. 2000; 14:130–136.


16. Hanna-Pladdy B, Daniels SK, Fieselman MA, Thompson K, Vasterling JJ, Heilman KM, et al. Praxis lateralization: errors in right and left hemisphere stroke. Cortex. 2001; 37:219–230.


17. Burrell JR, Hornberger M, Vucic S, Kiernan MC, Hodges JR. Apraxia and motor dysfunction in corticobasal syndrome. PLoS One. 2014; 9:e92944.


19. De Renzi E, Motti F, Nichelli P. Imitating gestures. A quantitative approach to ideomotor apraxia. Arch Neurol. 1980; 37:6–10.
20. Vanbellingen T, Kersten B, Van Hemelrijk B, Van de Winckel A, Bertschi M, Müri R, et al. Comprehensive assessment of gesture production: a new test of upper limb apraxia (TULIA). Eur J Neurol. 2010; 17:59–66.


21. Roy EA, Square-Storer P, Hogg S, Adams S. Analysis of task demands in apraxia. Int J Neurosci. 1991; 56:177–186.


22. Foundas AL, Macauley BL, Raymer AM, Maher LM, Rothi LJ, Heilman KM. Ideomotor apraxia in Alzheimer disease and left hemisphere stroke: limb transitive and intransitive movements. Neuropsychiatry Neuropsychol Behav Neurol. 1999; 12:161–166.
23. Buxbaum LJ, Johnson-Frey SH, Bartlett-Williams M. Deficient internal models for planning hand-object interactions in apraxia. Neuropsychologia. 2005; 43:917–929.


24. Buxbaum LJ, Shapiro AD, Coslett HB. Critical brain regions for toolrelated and imitative actions: a componential analysis. Brain. 2014; 137:1971–1985.


25. Baumard J, Lesourd M, Jarry C, Merck C, Etcharry-Bouyx F, Chauviré V, et al. Tool use disorders in neurodegenerative diseases: roles of semantic memory and technical reasoning. Cortex. 2016; 82:119–132.


26. Jarry C, Osiurak F, Besnard J, Baumard J, Lesourd M, Croisile B, et al. Tool use in left brain damage and Alzheimer's disease: what about function and manipulation knowledge? J Neuropsychol. 2016; 10:154–159.


27. Lesourd M, Le Gall D, Baumard J, Croisile B, Jarry C, Osiurak F. Apraxia and Alzheimer's disease: review and perspectives. Neuropsychol Rev. 2013; 23:234–256.


28. Ochipa C, Rothi LJ, Heilman KM. Conceptual apraxia in Alzheimer's disease. Brain. 1992; 115:1061–1071.


29. Tranel D, Kemmerer D, Adolphs R, Damasio H, Damasio AR. Neural correlates of conceptual knowledge for actions. Cogn Neuropsychol. 2003; 20:409–432.


30. Choi SH, Na DL, Kang E, Lee KM, Lee SW, Na DG. Functional magnetic resonance imaging during pantomiming tool-use gestures. Exp Brain Res. 2001; 139:311–317.


31. Beauchamp MS, Lee KE, Haxby JV, Martin A. Parallel visual motion processing streams for manipulable objects and human movements. Neuron. 2002; 34:149–159.


32. Chao LL, Haxby JV, Martin A. Attribute-based neural substrates in temporal cortex for perceiving and knowing about objects. Nat Neurosci. 1999; 2:913–919.


33. Frey SH. What puts the how in where? Tool use and the divided visual streams hypothesis. Cortex. 2007; 43:368–375.


34. Ebisch SJ, Babiloni C, Del Gratta C, Ferretti A, Perrucci MG, Caulo M, et al. Human neural systems for conceptual knowledge of proper object use: a functional magnetic resonance imaging study. Cereb Cortex. 2007; 17:2744–2751.


35. Canessa N, Borgo F, Cappa SF, Perani D, Falini A, Buccino G, et al. The different neural correlates of action and functional knowledge in semantic memory: an FMRI study. Cereb Cortex. 2008; 18:740–751.


36. Vingerhoets G, Acke F, Vandemaele P, Achten E. Tool responsive regions in the posterior parietal cortex: effect of differences in motor goal and target object during imagined transitive movements. Neuroimage. 2009; 47:1832–1843.


37. Watson CE, Buxbaum LJ. A distributed network critical for selecting among tool-directed actions. Cortex. 2015; 65:65–82.


38. Ogawa K, Imai F. Hand-independent representation of tool-use pantomimes in the left anterior intraparietal cortex. Exp Brain Res. 2016; 234:3677–3687.


39. Assmus A, Marshall JC, Ritzl A, Noth J, Zilles K, Fink GR. Left inferior parietal cortex integrates time and space during collision judgments. Neuroimage. 2003; 20:Suppl 1. S82–S88.


40. Bohlhalter S, Hattori N, Wheaton L, Fridman E, Shamim EA, Garraux G, et al. Gesture subtype-dependent left lateralization of praxis planning: an event-related fMRI study. Cereb Cortex. 2009; 19:1256–1262.


41. Fridman EA, Immisch I, Hanakawa T, Bohlhalter S, Waldvogel D, Kansaku K, et al. The role of the dorsal stream for gesture production. Neuroimage. 2006; 29:417–428.


42. Ramayya AG, Glasser MF, Rilling JK. A DTI investigation of neural substrates supporting tool use. Cereb Cortex. 2010; 20:507–516.


43. Caspers S, Eickhoff SB, Rick T, von Kapri A, Kuhlen T, Huang R, et al. Probabilistic fibre tract analysis of cytoarchitectonically defined human inferior parietal lobule areas reveals similarities to macaques. Neuroimage. 2011; 58:362–380.


44. Wheaton LA, Yakota S, Hallett M. Posterior parietal negativity preceding self-paced praxis movements. Exp Brain Res. 2005; 163:535–539.


45. Wheaton LA, Shibasaki H, Hallett M. Temporal activation pattern of parietal and premotor areas related to praxis movements. Clin Neurophysiol. 2005; 116:1201–1212.


47. Davare M, Andres M, Cosnard G, Thonnard JL, Olivier E. Dissociating the role of ventral and dorsal premotor cortex in precision grasping. J Neurosci. 2006; 26:2260–2268.


48. Davare M, Lemon R, Olivier E. Selective modulation of interactions between ventral premotor cortex and primary motor cortex during precision grasping in humans. J Physiol. 2008; 586:2735–2742.


49. Wheaton LA, Nolte G, Bohlhalter S, Fridman E, Hallett M. Synchronization of parietal and premotor areas during preparation and execution of praxis hand movements. Clin Neurophysiol. 2005; 116:1382–1390.


50. Pobric G, Jefferies E, Lambon Ralph MA. Category-specific versus category-general semantic impairment induced by transcranial magnetic stimulation. Curr Biol. 2010; 20:964–968.


51. Patterson K, Nestor PJ, Rogers TT. Where do you know what you know? The representation of semantic knowledge in the human brain. Nat Rev Neurosci. 2007; 8:976–987.


52. Lambon Ralph MA, Sage K, Jones RW, Mayberry EJ. Coherent concepts are computed in the anterior temporal lobes. Proc Natl Acad Sci U S A. 2010; 107:2717–2722.


53. Adlam AL, Bozeat S, Arnold R, Watson P, Hodges JR. Semantic knowledge in mild cognitive impairment and mild Alzheimer's disease. Cortex. 2006; 42:675–684.


54. Bozeat S, Lambon Ralph MA, Patterson K, Garrard P, Hodges JR. Non-verbal semantic impairment in semantic dementia. Neuropsychologia. 2000; 38:1207–1215.


55. Soliveri , Piacentini S, Girotti F. Limb apraxia in corticobasal degeneration and progressive supranuclear palsy. Neurology. 2005; 64:448–453.


56. Soliveri P, Piacentini S, Paridi D, Testa D, Carella F, Girotti F. Distalproximal differences in limb apraxia in corticobasal degeneration but not progressive supranuclear palsy. Neurol Sci. 2003; 24:213–214.


57. Foki T, Pirker W, Geißler A, Haubenberger D, Hilbert M, Hoellinger I, et al. Finger dexterity deficits in Parkinson's disease and somatosensory cortical dysfunction. Parkinsonism Relat Disord. 2015; 21:259–265.


58. Matt E, Foki T, Fischmeister F, Pirker W, Haubenberger D, Rath J, et al. Early dysfunctions of fronto-parietal praxis networks in Parkinson's disease. Brain Imaging Behav. 2017; 11:512–525.


59. Denes G, Mantovan MC, Gallana A, Cappelletti JY. Limb-kinetic apraxia. Mov Disord. 1998; 13:468–476.


60. Smania N, Aglioti SM, Girardi F, Tinazzi M, Fiaschi A, Cosentino A, et al. Rehabilitation of limb apraxia improves daily life activities in patients with stroke. Neurology. 2006; 67:2050–2052.


61. Smania N, Girardi F, Domenicali C, Lora E, Aglioti S. The rehabilitation of limb apraxia: a study in left-brain-damaged patients. Arch Phys Med Rehabil. 2000; 81:379–388.


62. Daumüller M, Goldenberg G. Therapy to improve gestural expression in aphasia: a controlled clinical trial. Clin Rehabil. 2010; 24:55–65.


63. Lefaucheur JP, André-Obadia N, Antal A, Ayache SS, Baeken C, Benninger DH, et al. Evidence-based guidelines on the therapeutic use of repetitive transcranial magnetic stimulation (rTMS). Clin Neurophysiol. 2014; 125:2150–2206.


64. Lefaucheur JP, Antal A, Ayache SS, Benninger DH, Brunelin J, Cogiamanian F, et al. Evidence-based guidelines on the therapeutic use of transcranial direct current stimulation (tDCS). Clin Neurophysiol. 2017; 128:56–92.


65. Wischnewski M, Schutter DJ. Efficacy and time course of theta burst stimulation in healthy humans. Brain Stimul. 2015; 8:685–692.


66. Wischnewski M, Schutter DJ. Efficacy and time course of paired associative stimulation in cortical plasticity: implications for neuropsychiatry. Clin Neurophysiol. 2016; 127:732–739.


68. Nguyen JP, Suarez A, Kemoun G, Meignier M, Le Saout E, Damier P, et al. Repetitive transcranial magnetic stimulation combined with cognitive training for the treatment of Alzheimer's disease. Neurophysiol Clin. 2017; 47:47–53.


69. Zhao J, Li Z, Cong Y, Zhang J, Tan M, Zhang H, et al. Repetitive transcranial magnetic stimulation improves cognitive function of Alzheimer's disease patients. Oncotarget. 2017; 8:33864–33871.


70. Lee J, Choi BH, Oh E, Sohn EH, Lee AY. Treatment of Alzheimer's disease with repetitive transcranial magnetic stimulation combined with cognitive training: a prospective, randomized, double-blind, placebo-controlled study. J Clin Neurol. 2016; 12:57–64.


71. Bianchi M, Cosseddu M, Cotelli M, Manenti R, Brambilla M, Rizzetti MC, et al. Left parietal cortex transcranial direct current stimulation enhances gesture processing in corticobasal syndrome. Eur J Neurol. 2015; 22:1317–1322.


72. Bolognini N, Convento S, Banco E, Mattioli F, Tesio L, Vallar G, et al. Improving ideomotor limb apraxia by electrical stimulation of the left posterior parietal cortex. Brain. 2015; 138:428–439.


73. Bieńkiewicz MM, Brandi ML, Goldenberg G, Hughes CM, Hermsdörfer J. The tool in the brain: apraxia in ADL. Behavioral and neurological correlates of apraxia in daily living. Front Psychol. 2014; 5:353.
74. Hanna-Pladdy B, Heilman KM, Foundas AL. Ecological implications of ideomotor apraxia: evidence from physical activities of daily living. Neurology. 2003; 60:487–490.