Abstract
Background and Purpose
Serial nerve conduction studies (NCSs) are recommended for differentiating axonal and demyelinating Guillain-Barré syndrome (GBS), but this approach is not suitable for early diagnoses. This study was designed to identify possible NCS parameters for differentiating GBS subtypes.
Methods
We retrospectively reviewed the medical records of 70 patients with GBS who underwent NCS within 10 days of symptom onset. Patients with axonal GBS and acute inflammatory demyelinating polyneuropathy (AIDP) were selected based on clinical characteristics and serial NCSs. An antiganglioside antibody study was used to increase the diagnostic certainty.
Results
The amplitudes of median and ulnar nerve sensory nerve action potentials (SNAPs) were significantly smaller in the AIDP group than in the axonal-GBS group. Classification and regression-tree analysis revealed that the distal ulnar sensory nerve SNAP amplitude was the best predictor of axonal GBS.
Guillain-Barré syndrome (GBS) is an immune-mediated inflammatory neuropathy with various subtypes, including acute inflammatory demyelinating polyneuropathy (AIDP), acute axonal motor neuropathy, acute motor and sensory axonal neuropathy, pharyngeal-cervical-brachial variant, and Miller-Fisher syndrome. GBS and its subtypes can generally be diagnosed clinically, but differentiating these subtypes—especially between AIDP and axonal GBS—can be challenging.
Acute axonal polyneuropathy is caused by molecular mimicry of human gangliosides by Campylobacter jejuni lipo-oligosaccharides.1 In axonal GBS, autoantibodies bind to gangliosides at the nodes of Ranvier, activating the complement system and disrupting sodium-channel clusters and axoglial junctions. However, AIDP directly attacks the myelin sheath, resulting in segmental demyelination and remyelination, although the exact pathophysiology of AIDP has not been established. Differences in pathophysiology should foster efforts to differentiate the GBS subtypes accurately, aiding future clinical research.
Electrodiagnostic study is the gold standard for differentiating between axonal and myelin lesions in early-stage acute polyneuropathy. However, current electrodiagnostic criteria have some limitations in diagnosing axonal GBS.234 The axonal type of GBS is pathophysiologically characterized not only by axonal degeneration, but also by reversible conduction failure. An inability to distinguish between demyelinating conduction block, reversible conduction failure, and length-dependent compound muscle action potential (CMAP) amplitude reduction may result in patients with axonal GBS being incorrectly classified as having AIDP.12
One recent study suggested that serial nerve conduction studies (NCSs) with thorough follow-ups are required to correctly differentiate GBS subtypes.2 Antiganglioside antibody tests will facilitate a correct diagnosis. However, these approaches are not suitable for early diagnosis due to the long period required to perform repeated NCSs. In this study we assessed the diagnostic value of various NCS parameters for differentiating early-stage axonal GBS from early-stage AIDP.
We retrospectively reviewed the Korean Inflammatory Neuropathy Consortium registry database, which holds data gathered from eight nationwide institutions in South Korea. Data obtained between January 2012 and December 2014 were reviewed using the following inclusion criteria: 1) diagnosis of GBS according to the current diagnostic criteria and clinically confirmed as axonal GBS or AIDP through serial NCSs with a follow-up period of more than 6 months,356 2) first NCS performed within 10 days of symptom onset, and 3) availability of antiganglioside antibody results. We excluded patients with underlying diseases that can cause neuropathy, such as diabetes mellitus, malignancy, and chronic alcohol consumption. Patients with a previous history of a peripheral nerve disease or radiculopathy other than GBS were also excluded. A precise classification was ensured by assaying antiganglioside antibodies. Patients who displayed clinical characteristics of axonal GBS and seropositivity for ganglioside antibodies were included in the final analysis. The AIDP group included patients with seronegativity for antiganglioside antibodies. Antibodies to gangliosides were tested using an enzyme-linked immunosorbent assay, as described elsewhere,17 with the serum considered to be seropositive for antiganglioside antibodies when the titer was ≥1:400. The Institutional Review Boards at all of the participating institutions approved this study: Korea University Anam Hospital, Yonsei University Severance Hospital, Dong-A University Hospital, Chonnam National University Hospital, Inje University Haeundae Paik Hospital, Hallym University Kangdong Sacred Heart Hospital, Kangbuk Samsung Hospital, and Konkuk University Medical Center. Informed consent was obtained from all subjects in the registry database.
All of the institutions followed the standard NCS protocol to exclude possible confounding factors.8 Motor NCSs of the median, ulnar, peroneal, and tibial nerves were recorded at the abductor pollicis brevis, abductor digiti minimi, extensor digitorum brevis, and abductor hallucis, respectively. A stimulus duration of 0.1 ms, a sensitivity setting of 2 mV, a sweep speed of 2 ms/division, and filtering from 5 Hz to 5 kHz were applied in all measurements. Sensory NCSs were performed on median and ulnar nerves using the orthodromic method, and on the sural nerve using the antidromic method. A sensitivity setting of 10 µV, a sweep speed of 1 ms/division, and filtering from 20 Hz to 3 kHz were used. F-waves were measured from the investigated motor nerves.
For the motor nerves, we obtained the latency, amplitude, and duration of CMAPs by stimulating at both the proximal sites (elbow for the median and ulnar nerves, popliteal fossa for the tibial nerve, and fibular head for the peroneal nerve) and distal sites (wrist for the median and ulnar nerves, and ankle for the tibial and peroneal nerves). The conduction velocity was calculated for the segment between the proximal and distal stimulation sites. The CMAP amplitude was measured from baseline to the negative peak. The CMAP duration was measured from the onset of a negative deflection from the baseline to when the last negative wave returned to the baseline. We also obtained F-wave latencies from these motor nerves. Conduction block was considered to be present in a specific nerve if the proximal-to-distal amplitude ratio was less than 0.5.3 For the median and ulnar sensory nerves, we measured the sensory nerve action potential (SNAP) amplitude at both the proximal and distal sites, in addition to measuring the distal latency. We also measured the conduction velocity at the distal regions (from wrist to finger for the median and ulnar nerves) and proximal regions (from elbow to wrist for the median and ulnar nerves). The SNAP amplitude was measured as the peak-to-peak amplitude. The conduction velocity and SNAP amplitude were measured for the sural nerve.
We compared each NCS parameter between the axonal-GBS and AIDP groups. Since results were available from only one side of a specific nerve in some patients, we chose to use the NCS data of the more-affected side when both sides had been measured.
Continuous variables were compared between groups using Student's t-tests or Mann-Whitney U tests depending on the variable distribution. Categorical variables were compared using chi-square tests or Fisher's exact tests. We assessed the optimal sensitivity and specificity of each nerve in discriminating axonal GBS from AIDP using receiver operating characteristic (ROC) curves, with cutoff values being determined. The classification and regression tree (CART) algorithm was also used to discriminate axonal-GBS patients from AIDP patients. The sensitivity was defined as the proportion of patients who were classified as axonal GBS according to the tree model among all axonal-GBS patients, and the specificity was the proportion of patients who were classified as AIDP according to the tree model among all AIDP patients. The analysis was performed using all NCS data and then repeated using only motor NCS data due to the predominant involvement of motor neurons in axonal GBS. Probability values of p<0.05 were considered statistically significant. Statistical analyses were performed using SPSS (version 20.0, SPSS Inc., Chicago, IL, USA) and R version 3.0.3 (R foundation for Statistical Computing Vienna, Austria) software.
Of 118 patients with GBS spectrum disorders, 70 patients with classic GBS were selected after excluding 31 patients with Miller-Fisher syndrome and 17 patients with the pharyngeal-cervical-brachial variant. After reviewing the antiganglioside antibody, only 27 seronegative AIDP patients and 31 seropositive patients classified as having axonal GBS were included. Among these patients, we finally analyzed 19 AIDP patients (9 men; age 57.4±15.0 years, mean±SD) and 25 axonal-GBS patients (12 men, age 58.2±11.2 years) who underwent the first NCSs within 10 days of symptom onset.
The antibody profile in the axonal-GBS group was as follows: immunoglobulin (Ig) G type GM1 antibody was found in 18 patients, GD1a in 4, GD1b in 6, GD3 in 1, GT1a in 2, GT1b in 1, GQ1b in 4, and IgM type GM1 in 3 patients.
The NCS results are presented in Table 1. The median distal CMAP latencies for all motor nerves were significantly delayed in the AIDP group than in the axonal-GBS group. Compared to the axonal-GBS group, the median conduction velocities in the AIDP group were significantly reduced in the median sensory nerve, the ulnar sensory nerve, and all motor nerves. None of the CMAP amplitudes differed between the groups. Furthermore, the proportion of patients with conduction blocks did not differ between the axonal-GBS (eight patients, 32%) and AIDP (seven patients, 37%) groups. SNAP amplitudes in the AIDP group were significantly decreased in the median and ulnar nerves.
Using parameters that differed significantly between the two groups, we calculated the sensitivity and the specificity with a specific cutoff point (Table 2). Distal SNAP amplitudes of the median and ulnar nerves were the strongest discriminators between the two groups. Considering only motor NCS parameters, the distal latency of the peroneal CMAP was the best predictor of axonal GBS.
According to the CART analysis, the distal ulnar SNAP amplitude was selected as the best predictor for discriminating axonal GBS from AIDP (Fig. 1A). The tree started from a root node (node 0) containing both axonal-GBS and AIDP patients. This node was then split based on the distal ulnar SNAP amplitude. Subjects with ulnar sensory nerve SNAP amplitudes of ≤7.5 mV were grouped into node 1, which comprised 15 AIDP patients (88%) and 2 axonal-GBS patients (12%). The remaining subjects were grouped into node 2, comprising of 4 AIDP patients (15%) and 23 axonal-GBS patients (85%). The sensitivity and specificity of this model for predicting axonal GBS were 92% and 79%, respectively.
When we considered only the motor nerves, the distal latency in the peroneal motor nerve and the duration in the proximal tibial motor nerve were the best predictors of axonal GBS (Fig. 1B). Node 0 was split based on the CMAP distal latency obtained from the peroneal nerve, creating node 1 (≤7.935 ms) and node 2 (>7.935 ms). The subjects in node 1 were split into nodes 3 and 4 based on the proximal CMAP duration obtained from the tibial nerve using a cutoff value of 7.125 ms. Patients with a distal latency in the peroneal motor nerve of longer than 7.935 ms were more likely to be diagnosed as AIDP. Among the patients with a distal latency in the peroneal motor nerve of shorter than 7.935 ms, all of them whose proximal duration in the tibial nerve was less than 7.125 ms had axonal GBS. The overall sensitivity and specificity of this model were 96% and 63%, respectively.
We investigated early NCS findings in GBS patients in order to discriminate patients with axonal GBS and seropositivity for antiganglioside antibodies from patients with AIDP. Early NCS parameters that could be used to differentiate these two groups were the distal latency, conduction velocity, and the amplitude of various motor and sensory nerve potentials, especially upper limb distal SNAP amplitudes and lower limb CMAP latencies or durations.
Axonal GBS is associated with Campylobacter jejuni infection,910 which mimics gangliosides in humans, subsequently resulting in the production of autoantibodies to the gangliosides.11 Since gangliosides stabilize paranodal junctions and ion-channel clusters in myelinated nerve fibers,12 the antiganglioside antibodies can cause axonal GBS.13 The pathogenesis of GBS associated with antiganglioside antibodies appears to involve complement activation, which could be utilized as a target for therapy.114 Since prompt identification of this subtype of GBS leads to early treatment, it is important to differentiate this subtype from AIDP, which is not usually associated with antiganglioside antibodies. However, the current diagnostic criteria for GBS are not particularly useful for differentiating axonal GBS from AIDP in the early stage since some patients with axonal GBS have transient conduction blocks.21516
The present results are similar to previous suggestions that patients with antiganglioside antibodies have shorter distal latencies and faster conduction velocities in motor nerves, as well as larger amplitudes and faster conduction velocities in sensory nerves.17 There are many reports of a single electro-physiological study being considered inadequate for classifying disease subtypes.21517 Although it was suggested recently that a single electrophysiological study may be used to diagnose GBS subtypes correctly,18 information about antiganglioside antibodies was not reported. Overall, our results support the usefulness of early NCS findings in addition to seropositivity for antiganglioside antibodies in the diagnosis of axonal GBS.
In GBS, abnormalities cannot be detected in electrodiagnostic studies until 2 weeks after symptom onset,19 which might hinder early treatment. This has prompted many attempts to diagnose AIDP earlier using electrodiagnostic studies.202122 A recent study proposed new diagnostic criteria for early AIDP (≤7 days from onset) that had a sensitivity of 81% and a specificity of 67%.21 That study found that the most common findings of early AIDP were abnormalities in the H-reflexes (97%), motor conduction velocity (78%), and distal latency in motor nerves (78%).21 Another important early finding was a sural sparing pattern.23 In line with these previous results, our study also found decreased conduction velocities and prolonged distal latencies of motor nerves in AIDP patients. Furthermore, the sural sensory nerves were not affected in either axonal-GBS or AIDP patients. We used ROC curves to identify which NCS parameters are the most useful in differentiating AIDP from axonal GBS, which revealed that the areas under the ROC curves were larger for the SNAP amplitude and conduction velocity in both median and ulnar nerves, and for the CMAP distal latency and duration in the peroneal nerve. Consistent with the literature,1624 conduction blocks could not be used to differentiate axonal GBS from AIDP. A particularly notable finding was that the results for the median and ulnar sensory nerves were the most powerful for differentiating axonal GBS from AIDP. This is consistent with a previous study showing that axonal GBS with antiganglioside antibodies rarely involves sensory nerves, and that the presence of abnormal sensory conduction is more likely to be indicative of AIDP.25 CART analysis showed that distal ulnar SNAP amplitude was the most powerful discriminating parameter. When we performed the analysis using only motor parameters, the distal peroneal CMAP latency and the proximal tibial CMAP duration were the most promising for discriminating between axonal GBS and AIDP. However, these two motor NCS parameters require careful interpretation due to the high likelihood of technical error.
Our study was limited by the smallness of the sample, which hindered the ability to draw definitive conclusions. However, we classified GBS based on the antibody status as well as clinical features and serial NCS findings, which ensured subject homogeneity. Further prospective studies involving various subtypes of antiganglioside antibodies should be performed to confirm our findings and to develop more reliable electrodiagnostic criteria for early-stage axonal GBS.
In conclusion, conduction block is one of the key findings used to differentiate demyelinating lesions from axonal lesions, but it was found to not be useful in differentiating AIDP from axonal GBS. However, we found that the distal SNAP amplitude in the median and ulnar nerves was powerful in discriminating between axonal GBS and AIDP. Although a single electrodiagnostic study cannot completely differentiate axonal GBS from AIDP in early-stage disease, careful interpretation of NCS parameters might be helpful in their differentiation.
Figures and Tables
Fig. 1
Classification and regression tree (CART) diagram for discriminating acute axonal Guillain-Barré syndrome (GBS) from acute inflammatory demyelinating polyneuropathy (AIDP) using a set of independent variables. A: CART diagram involving a set of independent variables related to both motor and sensory nerves. According to this model, patients in nodes 1 and 2 were classified as AIDP and axonal-GBS patients, respectively. B: CART diagram involving variables for motor nerves only. According to this model, patients in nodes 3 and 4 were classified as axonal GBS, and those in node 2 were classified as AIDP patients. PerMDL: distal latency in peroneal motor nerve, TibMPDur: duration in proximal tibial motor nerve, UlnSDAm: distal amplitude in ulnar sensory nerve.
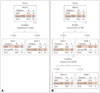
Table 1
Comparison of nerve conduction study parameters between axonal GBS and acute inflammatory demyelinating polyneuropathy
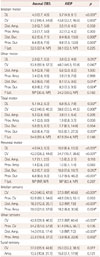
The data in parentheses represent interquartile ranges.
*p<0.05.
AIDP: acute inflammatory demyelinating polyneuropathy, Amp.: amplitude, AXONAL GBS: acute axonal Guillain-Barré syndrome, CV: conduction velocity, Dist.: distal, DL: distal latency, Dur.: duration, F Lat.: F-wave latency, NP: not provoked, Prox.: proximal.
Table 2
Sensitivity and specificity of nerve conduction study parameters determined by receiver operating characteristics curves
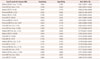
Acknowledgements
This work was supported by a National Research Foundation of Korea (NRF) grant funded by the Korean Government (MSIP; no. NRF-2015R1 A5A7037674) and the Technology Innovation Program (or the Industrial Strategic Technology Development Program) (10049743, "Establishing a medical device development open platform, as a hub for accelerating close firm-hospital communication") funded by the Ministry of Trade, Industry and Energy (MI, Korea).
References
1. Kim JK, Bae JS, Kim DS, Kusunoki S, Kim JE, Kim JS, et al. Prevalence of anti-ganglioside antibodies and their clinical correlates with guillain-barré syndrome in Korea: a nationwide multicenter study. J Clin Neurol. 2014; 10:94–100.


2. Uncini A, Manzoli C, Notturno F, Capasso M. Pitfalls in electrodiagnosis of Guillain-Barré syndrome subtypes. J Neurol Neurosurg Psychiatry. 2010; 81:1157–1163.
3. Hadden RD, Cornblath DR, Hughes RA, Zielasek J, Hartung HP, Toyka KV, et al. Plasma Exchange/Sandoglobulin Guillain-Barré Syndrome Trial Group. Electrophysiological classification of Guillain-Barré syndrome: clinical associations and outcome. Ann Neurol. 1998; 44:780–788.


4. Ho TW, Mishu B, Li CY, Gao CY, Cornblath DR, Griffin JW, et al. Guillain-Barré syndrome in northern China. Relationship to Campylobacter jejuni infection and anti-glycolipid antibodies. Brain. 1995; 118(Pt 3):597–605.


5. Asbury AK, Cornblath DR. Assessment of current diagnostic criteria for Guillain-Barré syndrome. Ann Neurol. 1990; 27:Suppl. S21–S24.


7. Kusunoki S, Chiba A, Kon K, Ando S, Arisawa K, Tate A, et al. N-acetylgalactosaminyl GD1a is a target molecule for serum antibody in Guillain-Barré syndrome. Ann Neurol. 1994; 35:570–576.


8. Koo YS, Cho CS, Kim BJ. Pitfalls in using electrophysiological studies to diagnose neuromuscular disorders. J Clin Neurol. 2012; 8:1–14.


9. Ogawara K, Kuwabara S, Mori M, Hattori T, Koga M, Yuki N. Axonal Guillain-Barré syndrome: relation to anti-ganglioside antibodies and Campylobacter jejuni infection in Japan. Ann Neurol. 2000; 48:624–631.


10. Drenthen J, Yuki N, Meulstee J, Maathuis EM, van Doorn PA, Visser GH, et al. Guillain-Barré syndrome subtypes related to Campylobacter infection. J Neurol Neurosurg Psychiatry. 2011; 82:300–305.
11. Neisser A, Schwerer B, Bernheimer H, Moran AP. Ganglioside-induced antiganglioside antibodies from a neuropathy patient crossreact with lipopolysaccharides of Campylobacter jejuni associated with Guillain-Barré syndrome. J Neuroimmunol. 2000; 102:85–88.


12. Susuki K, Baba H, Tohyama K, Kanai K, Kuwabara S, Hirata K, et al. Gangliosides contribute to stability of paranodal junctions and ion channel clusters in myelinated nerve fibers. Glia. 2007; 55:746–757.


13. Uncini A, Susuki K, Yuki N. Nodo-paranodopathy: beyond the demyelinating and axonal classification in anti-ganglioside antibody-mediated neuropathies. Clin Neurophysiol. 2013; 124:1928–1934.


14. Willison HJ. The immunobiology of Guillain-Barré syndromes. J Peripher Nerv Syst. 2005; 10:94–112.
15. Hiraga A, Kuwabara S, Ogawara K, Misawa S, Kanesaka T, Koga M, et al. Patterns and serial changes in electrodiagnostic abnormalities of axonal Guillain-Barré syndrome. Neurology. 2005; 64:856–860.


16. Kokubun N, Nishibayashi M, Uncini A, Odaka M, Hirata K, Yuki N. Conduction block in acute motor axonal neuropathy. Brain. 2010; 133:2897–2908.


17. Sekiguchi Y, Uncini A, Yuki N, Misawa S, Notturno F, Nasu S, et al. Antiganglioside antibodies are associated with axonal Guillain-Barré syndrome: a Japanese-Italian collaborative study. J Neurol Neurosurg Psychiatry. 2012; 83:23–28.


18. Rajabally YA, Durand MC, Mitchell J, Orlikowski D, Nicolas G. Electrophysiological diagnosis of Guillain-Barré syndrome subtype: could a single study suffice? J Neurol Neurosurg Psychiatry. 2015; 86:115–119.


19. Albers JW, Kelly JJ Jr. Acquired inflammatory demyelinating polyneuropathies: clinical and electrodiagnostic features. Muscle Nerve. 1989; 12:435–451.


20. Gordon PH, Wilbourn AJ. Early electrodiagnostic findings in Guillain-Barré syndrome. Arch Neurol. 2001; 58:913–917.


21. Chanson JB, Echaniz-Laguna A. Early electrodiagnostic abnormalities in acute inflammatory demyelinating polyneuropathy: a retrospective study of 58 patients. Clin Neurophysiol. 2014; 125:1900–1905.


22. Albertí MA, Alentorn A, Martínez-Yelamos S, Martínez-Matos JA, Povedano M, Montero J, et al. Very early electrodiagnostic findings in Guillain-Barré syndrome. J Peripher Nerv Syst. 2011; 16:136–142.


23. Derksen A, Ritter C, Athar P, Kieseier BC, Mancias P, Hartung HP, et al. Sural sparing pattern discriminates Guillain-Barré syndrome from its mimics. Muscle Nerve. 2014; 50:780–784.

