Abstract
Background and Purpose
The wnt/β-catenin signaling pathway plays a critical role in embryonic development and adult-tissue homeostasis. Recent investigations implicate the importance of wnt/β-catenin signaling in normal wound healing and its sustained activation being associated with fibrogenesis. We investigated the immunolocalization and activation of wnt/β-catenin in polymyositis (PM), dermatomyositis (DM), and Duchenne muscular dystrophy (DMD).
Methods
Immunofluorescence staining and Western blot analysis of β-catenin were performed in muscle specimens from 6 PM, 8 DM, and 6 DMD subjects. The β-catenin/Tcf4 DNA-binding activity in muscle was studied using an electrophoretic mobility shift assay (EMSA), and serum wnt/β-catenin/Tcf transcriptional activity was measured using a luciferase reporter gene assay.
Results
Immunoreactivity for β-catenin was found in the cytoplasm and nuclei of muscle fibers in PM, DM, and DMD. The protein level of β-catenin was elevated, and EMSA analysis confirmed the activation of wnt/β-catenin signaling. The transcriptional activities of β-catenin/Tcf in the circulation were increased in patients with PM, DM, and DMD, especially in those with interstitial lung disease, and these transcriptional activities decreased when PM or DM patients exhibited obvious clinical improvements.
Idiopathic inflammatory myopathies (IIMs)—including polymyositis (PM), dermatomyositis (DM), and sporadic inclusion-body myositis—constitute a heterogeneous group of acquired diseases of skeletal muscle.12 Patients with IIMs manifest moderate-to-severe muscle weakness, elevation of serum creatine kinase (CK), and electromyography (EMG) features of myopathy. Muscle biopsies generally reveal necrotic and regenerating fibers, inflammatory infiltration, and up-regulation of major histocompatibility complex class-I (MHC-I). Patients with IIMs have longer clinical courses and can exhibit chronic and progressive muscle fibrosis. In addition to muscle involvement, the lung is one of the most common extra muscular targets. Interstitial lung disease (ILD), classified as interstitial pneumonia and pulmonary interstitial fibrosis, is a prevalent and often devastating manifestation of IIM.3
Duchenne muscular dystrophy (DMD) is caused by mutations in the gene that encodes the 427-kDa cytoskeletal protein dystrophin. Muscle biopsies of patients with DMD reveal necrotic and regenerating fibers, variability in muscle fiber size, increased amounts of endomysial and perimysial connective tissue, as well as scattered hypertrophic and hypercontracted fibers. In addition to the dystrophic changes, inflammatory infiltrates can be encountered when performing muscle biopsies of DMD patients.4
Wnt proteins form a family of highly conserved secreted signaling molecules that control embryonic development and adult homeostasis via the transcription co-activator β-catenin.567 When wnt signaling is absent, β-catenin can be phosphorylated by nonphosphorylated glycogen synthase kinase 3-β (GSK-3β) (active) and targeted for degradation. However, when a wnt ligand binds to its membrane receptor, GSK-3β is phosphorylated (inactive) and loses its sustained kinase activity. Thus, β-catenin cannot be phosphorylated by GSK-3β. Unphosphorylated β-catenin will accumulate in the cytoplasm and then translocate to the nucleus, where it binds to T-cell factors (Tcf, a family of transcription factors involved in mediating wnt signaling) and stimulates the transcription of target genes.8 The physiologic wnt/β-catenin signaling pathway is crucial for normal organ development, and evidence for several main roles played by wnt/β-catenin in skeletal muscle regeneration has been presented recently: wnt/β-catenin signaling 1) is necessary to induce muscle-specific gene transcription,9 2) promotes the self-renewal of skeletal-muscle satellite cells in response to skeletal muscle injury,10 and 3) is involved in switching from cell proliferation to myogenic differentiation.11 Moreover, enhanced wnt/β-catenin signaling also plays important roles in the pathogenesis of fibrotic diseases, including pulmonary fibrosis,12 renal fibrosis,13 as well as hypertrophic scars.14 Several wnt molecules appear to be up-regulated in systemic sclerosis15 and fibrotic human skin15 and muscle,16 indicating that fibroblast activation is regulated by wnt/β-catenin signaling.
Since β-catenin plays a key role in the process of fibrosis and muscle regeneration, it is necessary to determine whether β-catenin is activated in muscle tissue from IIMs and DMD patients, in which muscle regeneration and fibrosis are common. In the present study, we have demonstrated that wnt/β-catenin signaling was activated in muscle tissue and the circulation of patients with IIMs and DMD.
This study was approved by the ethics committee of Qilu Hospital, Shandong University. Informed consent was obtained from all subjects according to the Declaration of Helsinki. Muscle biopsy samples were obtained from 6 PM, 8 DM, and 6 DMD subjects. PM and DM were diagnosed according to the Dalakas 2003 diagnostic criteria.2 Serving as an additional diagnostic test, anti-MHC-I immunostaining was also performed in muscle specimens from DM and PM patients. The diagnosis of DMD relied primarily on clinical phenotype, high CK level, muscle biopsy findings, and immunostaining for dystrophin on muscle specimens. In addition, three nemaline myopathy (NM) patients in whom necrosis, regeneration, and fibrosis were not found in muscle biopsies were recruited as a diseased control group. To reinforce the importance of our findings, we also included six subjects as a normal-muscle-pathology control group. Although these subjects manifested various clinical weaknesses, they were diagnosed as not having common muscular disorders based on normal EMG and muscle pathology findings.
At the time of diagnosis, an open-biopsy sample was obtained from the biceps brachii under local anesthesia after obtaining written informed consent. Muscle biopsy specimens were frozen in isopentane, cooled in liquid nitrogen, and stored at -80℃ until processed. For histological examinations, serial frozen sections (8 µm thick) were stained with hematoxylin and eosin, modified Gomori trichrome, succinate dehydrogenase (SDH), cytochrome c oxidase (CCO), combined CCO/SDH, nicotinamide adenine dinucleotide-tetrazolium (NADH-TR), periodic acid Schiff (PAS), and Oil Red O (ORO).
Muscle sections (6 µm thick) were stained with anti-β-catenin antibody (Cell Signaling Technology, Danvers, MA, USA). Concentration-matched and species-specific immunoglobulins (Beyotime, China) were used as control antibodies. After labeling with Cy3-tagged secondary antibodies, slices were analyzed under a fluorescence microscope (Olympus, Japan).
Western blotting for β-catenin (Cell Signaling Technology), GSK-3β (Cell Signaling Technology) and pGSK-3β (phospho-GSK-3β ser9, Cell Signaling Technology) was performed on muscle homogenates. Equal loading was confirmed based on the GAPDH level (Beyotime, Beijing, China). Detection and quantification were performed with the Li-Cor Odyssey (Lincoln, NE, USA) imaging system and its software.
The procedures used to isolate the nuclear proteins have been described elsewhere.4 Nuclear extract (15 µg) was incubated for 30 minutes at room temperature with double-stranded oligonucleotide probe end labeled with near-infrared IRDye® 800 (Li-Cor, Lincoln, NE, USA). The DNA probes used were synthesized according to previously reported sequences.17 Samples were analyzed by 5% nondenaturing polyacrylamide gel electrophoresis, and scanned and analyzed using the Li-Cor Odyssey imaging system and its software.
Peripheral blood samples were obtained from all subjects at their first visit to our hospital. We also collected blood samples from PM and DM patients when they exhibited clinical improvement after receiving steroids or other immunosuppressant treatments. Blood samples were drawn into tubes containing inertia separation gel and coagulant, and then the serum was isolated by centrifugation (2,000 g for 10 min). The supernatant was collected and stored at -80℃ until used. HEK293 cells were cultured and transfected with M50 Super (8X) TopFlash (Addgene, Cambridge, MA, USA), which reports TCF activity in 24-well plates at 37℃. After 12 hours, the medium was changed to DMEM containing 20% serum from the subjects. Luciferase activity was measured 24 hours later using the Dual-Luciferase Reporter Assay System (Progema, San Luis Obispo, CA, USA). The activation was normalized relative to that of Renilla luciferase.
The detailed clinical and pathological features of the subjects were summarized in Table 1. The IIMs group comprised 6 PM and 8 DM patients. All patients with DM showed the typical skin lesions. Chest CT scans showed pulmonary interstitial fibrosis in 2 PM and 3 DM patients, and acute interstitial pneumonia in 2 PM and 2 DM patients. All patients with IIMs presented elevated plasma CK levels, ranging from 10 to 40 times the normal upper limit. EMG showed myogenic changes in 12 patients. All patients received immunosuppressive therapy, to which they responded well during the clinical follow-up. Histochemical staining in PM patients revealed variation in fiber size (n=6), necrotic and basophilic regenerating fibers (n=6), and an increased amount of interstitial connective tissue (n=6). Inflammatory infiltrates were evident, as indicated by nonnecrotic muscle fibers infiltrated by mononuclear cells (n=4), focal lymphocytic infiltrates (n=6), and typical perivascular mononuclear infiltrates (n=2). MHC-I immunostaining revealed the focal presence of MHC-I along the sarcolemma of nonnecrotic fibers (n=6). Muscle biopsy samples obtained from DM patients displayed necrotic and basophilic regenerating fibers (n=8), perifascicular atrophy (n=8), and the proliferation of interstitial connective tissue (n=8). Inflammation was predominantly perivascular (n=7) or in the interfascicular septa (n=5). MHC-I immunostaining exhibited intense, diffused sarcolemmal positivity in almost all fibers, especially in fibers with perifascicular atrophy. Six patients with DMD exhibited high CK levels (more than 50 times the normal upper limit) in serum. Muscle biopsies revealed obvious necrotic and regenerating fibers (n=6), increased amounts of interstitial connective tissue (n=6), and scattered inflammatory cell infiltrates (n=1). Immunostaining showed dystrophin deficiency in all subjects. Staining with NADH-TR, SDH, CCO, combined CCO/SDH, PAS, and ORO in muscle specimens from IIMs and DMD patients produced normal or nonspecific findings. Three patients with NM presented with generalized mild muscle weakness since childhood. The CK level in these patients was within the normal range. Muscle biopsy samples showed typical rod bodies and mild variation of fiber size. No necrosis and regenerating fibers or muscle fibrosis was found.
The role of wnt/β-catenin signaling in IIMs and DMD patients was examined by investigating β-catenin subcellular localization in muscle fibers. Staining for β-catenin and 4',6-diamidino-2-phenylindole (DAPI) in normal-muscle-pathology control specimens showed that β-catenin was expressed normally in the membrane of almost all muscle fibers and also in about 22% of nuclei (Fig. 1Aa-c). No cytoplasmic positivity was observed. However, not only increased β-catenin membranal intensity but also cytoplasmic accumulation and nucleus translocation were found in muscle fibers from patients with IIMs and DMD. In PM patients (Fig. 1Ad-f), nearly all regenerating fibers displayed cytoplasmic and nuclear β-catenin positivity. Moreover, most of the necrotic fibers were also positive for β-catenin, with only a few at the late stage of necrosis displaying no β-catenin expression. Nevertheless, about 40% of intact muscle fibers exhibited the nuclear localization of β-catenin, which was twice that in control specimens. The intense membrane, cytoplasm, and nuclear β-catenin positivity in DM patients was particularly evident in areas with perifascicular atrophy (Fig. 1Ag-i). A semiquantitative analysis showed that on average 74% of intact, necrotic, and regenerating fibers exhibited nuclei positive for β-catenin. The exact number of intact fibers with β-catenin-nuclei positivity was difficult to assess due to the severe and extensive muscular damage in most of our DM patients. In patients with DMD, β-catenin immunoreactivity showed a similar pattern to that observed in PM, being localized in the cytoplasm and nuclei of necrotic and regenerating fibers. One-quarter of intact muscle fibers in DMD patients exhibited nuclear positivity (Fig. 1Aj-l). As a diseased control, we also performed β-catenin immunostaining in muscle specimens from NM patients. However, staining for β-catenin and DAPI in these specimens only showed muscle membrane and 27% nuclei positivity (Fig. 1Am-o) which is very similar to the staining pattern in muscle specimens from the normal-muscle-pathology control group.
Western blotting was next performed to detect the protein level of β-catenin in skeletal muscle. The results showed the β-catenin level was significantly elevated in most patients with IIMs and DMD, except in patients #PM2, #PM4, and #DM2. Relative to control samples, there were mean increases of 293% (p=0.007), 304% (p=0.001), and 223% (p=0.004) in PM, DM, and DMD patients, respectively (Fig. 1B and C). Moreover, like the results for β-catenin immunofluorescence, the protein level of β-catenin in muscle samples from NM patients was consistently low. Since β-catenin accumulation has been demonstrated in IIMs and DMD patients, we next decided to investigate if GSK-3β was phosphorylated in these subjects. The obtained data indicated that, compared to normal-muscle-pathology control subjects, GSK-3β was highly phosphorylated (Fig. 2) in muscle samples with β-catenin overexpression, while nonphosphorylated GSK-3β remained unchanged (Fig. 1B and D). A semiquantitative analysis showed that the pGSK-3β/GSK-3β ratios increased to 180% (p=0.006), 393% (p<0.001), and 371% (p=0.008) in PM, DM, and DMD patients, respectively. Together these results indicate that GSK-3β phosphorylation stabilizes β-catenin in the cytoplasm and nucleus of muscle fibers from IIMs and DMD patients.
The DNA-binding activity of β-catenin/Tcf4 in skeletal muscle was also assayed using an electrophoretic mobility shift assay (EMSA). Consistent with the results of β-catenin quantification, the DNA-binding activities of β-catenin/Tcf4 were absent or low in controls as well as NM patients (p=0.194) and variably increased in most IIMs and DMD patients (Fig. 3, Table 1). A semiquantitative analysis showed there were mean increases of 269% (p=0.001), 361% (p<0.001), and 294% (p=0.001) in the β-catenin/Tcf4 DNA-binding activities in PM, DM, and DMD patients, respectively, relative to the normal controls.
Luciferase reporter gene assays were next performed to detect whether β-catenin/Tcf4 transcriptional activity was also activated in the circulation of our patients. The results showed that the β-catenin/Tcf transcriptional activities in PM, DM, and DMD patients were 2.91-fold (p=0.001), 3.71-fold (p<0.001), and 2.36-fold (p<0.001) higher, respectively, than those in control samples (Fig. 4A and B). Furthermore, the β-catenin/Tcf transcriptional activities in patients with ILD (Patients #PM1–#PM4 and #DM1–#DM5) were moderately increased compared to IIMs patients with normal pulmonary radiological findings (Fig. 4A). A quantitative analysis showed that the activity in IIMs patients with ILD was 1.54-fold (p=0.01) higher than that in patients without ILD (Fig. 4C). However, as expected, the wnt/β-catenin transcriptional activities in the circulation of NM patients were as low as those in normal-muscle-pathology controls (p=0.329).
We next tested whether β-catenin/Tcf4 transcriptional activities in the circulation were correlated with clinical improvement. Treatment with glucocorticoids or other immunosuppressants started immediately in our IIMs group once the diagnosis of PM or DM had been established. After 6–12 months of follow-up, all subjects exhibited dramatic clinical improvements, except for PM3, in whom relapse occurred when the dosage of corticosteroids was reduced to 20 mg per day. As expected, the serum β-catenin/Tcf activities decreased—ranging from 12.4% to 63.2% (Fig. 4D)—in patients exhibiting clinical improvements. This result indicates that β-catenin/Tcf4 transcriptional activities in the circulation were correlated with the clinical improvements.
Our study is the first demonstration of the activation of wnt/β-catenin signaling in IIMs and DMD patients. In PM and DMD patients, β-catenin-positive signals were evident not only within the cytoplasm but also within nuclei of necrotic and regenerating fibers. In DM patients, fibers in perifascicular atrophy areas exhibited intense membranal, cytoplasmic, and nuclear β-catenin positivity, due to more necrotic and regenerating fibers being present in this compartment. Combining the immunocytochemistry, Western blotting, EMSA, and luciferase reporter gene assay results, our study suggests that stabilized β-catenin—at least partly through GSK-3β phosphorylation—enters the nucleus and forms a complex with Tcf, which is able to bind to specific sequences in the promoter region of target genes.
The similarity in the localization of β-catenin—that is, strong membranal, cytoplasmic, and nuclear β-catenin positivity in necrotic and regenerating fibers of PM, DM, and DMD patients—suggests that wnt/β-catenin signaling can be activated similarly in these three diseases, even though their underlying pathophysiologic mechanisms are different. It is known that wnt/β-catenin target genes are diverse and usually cell-and context-specific. This means that it is necessary to identify the different downstream target genes of the wnt/β-catenin signaling pathway in these different muscular diseases. However, it is still possible that activation of wnt/β-catenin is a phenomenon secondary to muscle damage regardless of its cause. In this regard, a common downstream molecular event may occur in these different diseases. In addition, our results showed there was no obvious difference in the protein level of β-catenin, the DNA-binding activity of β-catenin/Tcf4, or the serum wnt/β-catenin transcriptional activities between NM patients and normal-muscle-pathology control subjects. Very little muscle necrosis, regeneration, or fibrosis was found in NM muscle specimens, which further indicates that the activation of wnt/β-catenin signaling may be a phenomenon secondary to muscle damage.
The activation of wnt/β-catenin signaling in regenerating muscle fibers of IIMs and DMD patients has not been reported previously. However, several main roles played by wnt/β-catenin in skeletal muscle regeneration have been presented recently: wnt/β-catenin signaling 1) is necessary to induce muscle-specific gene transcription,9 2) promotes self-renewal of skeletal-muscle satellite cells in response to skeletal muscle injury,10 and 3) is involved in switching from cell proliferation to myogenic differentiation.11 According to these findings, the β-catenin activation in the regenerating fibers of our IIMs and DMD patients suggests that wnt/β-catenin signaling participates in the process of muscle regeneration or muscle satellite-cell differentiation.
In the current study, we also found that necrotic fibers in IIMs and DMD patients were positive for β-catenin, similar to previous results for nuclear factor kappa-B (NF-κB) activation in IIMs and DMD patients.4 It has been demonstrated that necrotic fibers displayed strong expression of tumor necrosis factor-alpha (TNF-α).18 Interestingly, a recent study demonstrated that TNF-α increased MHC-I expression, along with enhancing β-catenin, hypoxia-inducible factor 1-alpha, and NF-κB activities.19 Based on the results of these studies, it is postulated that TNF-α in necrotic fibers can up-regulate β-catenin expression. Moreover, it is known that necrotic fibers exhibit excessive oxidative stress,420 and oxidative stress could activate wnt/β-catenin signaling.21 Thus, we cannot exclude that oxidative stress also plays roles in β-catenin activation in necrotic fibers. Furthermore, researches using cell models of facioscapulohumeral muscular dystrophy22 and oculopharyngeal muscular dystrophy23 have also demonstrated that activation of the wnt/β-catenin pathway was able to prevent muscular death or apoptosis. Thus, it can be inferred that up-regulation of β-catenin in necrosis fibers may be a protective mechanism against excessive death or apoptosis.
Wnt/β-catenin signaling also plays important roles in the pathogenesis of fibrotic diseases. Chilosi et al.12 reported aberrant wnt/β-catenin pathway activation in epithelial cells and fibroblasts in idiopathic pulmonary fibrosis, and Beyer et al.15 identified β-catenin as a key participant in fibroblast activation and tissue fibrosis in systemic sclerosis. Similar findings have also been obtained in renal fibrosis.13 More recently, it was shown that wnt/β-catenin increased muscular fibrosis during aging by promoting muscle stem-cell conversion.16 The accumulation of β-catenin in fibroblasts contributes to cardiac and skeletal-muscle fibrosis in X-linked Emery-Dreifuss muscular dystrophy.24 Moreover, wnt/β-catenin activation in mdx mice stimulated the stromal cell in muscles to produce collagen.25 The patients in the current study exhibited extensive fibrotic pathology in muscle biopsies and higher activities of wnt/β-catenin in muscle tissue and the circulation. Based on the correlation between wnt/β-catenin and the fibrotic process established in previous studies, we consider that activation of wnt/β-catenin signaling plays at least a partial role in the pathogenesis of muscle fibrosis in IIMs and DMD patients, even though β-catenin expression was not detected in fibroblasts or muscle stem cells in the muscle specimens from our patients. Furthermore, the serum wnt/β-catenin activities were higher in IIM patients with ILD than in patients with normal pulmonary radiological findings. It is speculated that wnt/β-catenin activation is one of the mechanisms of ILD in IIMs patients.
Immunosuppressants are effective drugs for both pulmonary fibrosis and IIMs. When the patients in the current study exhibited clinical improvement after receiving steroids or other treatment with other immunosuppressants, the wnt/β-catenin activities in the circulation were significantly decreased. This result is likely to be due to mitigation of muscle damage, but we cannot exclude the possibility that immunosuppressants directly affect wnt/β-catenin inhibition. Further investigations are needed to clarify this.
A major limitation of our study is that we did not determine the exact role that wnt/β-catenin played in PM, DM, and DMD. Thus, further investigations are needed to uncover the upstream wnt ligands (e.g., wnt1, wnt2, wnt4, or wnt6) as well as the downstream β-catenin target genes (e.g., Axin2, VEGF, or FGF9) in these different diseases to determine whether wnt/β-catenin exerts the same or different roles in the pathogenesis of PM, DM, and DMD. Second, although up-regulation of serum wnt/β-catenin transcriptional activities was much more prominent in IIMs patients with ILD than in those with normal pulmonary radiological findings, we did not establish the cause-and-effect relationship between wnt/β-catenin activation and ILDs. New testing methods should be developed for the in situ analysis of wnt/β-catenin signaling in the lung tissue of patients or disease models. A third potential limitation of this study is the relative smallness of the sample. While 6 PM and 8 DM patients were enrolled in this study, only 4 PM and 5 DM patients presented pulmonary complications. Thus, the importance of our findings could be reinforced by investigating more IIM patients with ILD. In addition, although the subjects in the normal-muscle-pathology control group were not diagnosed as having common muscular disorders, due to the presence of normal EMG and muscle pathology findings, they were not considered as normal subjects due to their clinical weaknesses. Therefore, to further highlight our conclusions, future studies need to compare wnt/β-catenin activation between diseased patients (IIMs and DMD) and normal subjects without clinical weaknesses and abnormal EMG and muscle pathology findings.
In conclusion, we have identified that wnt/β-catenin is activated in muscle tissue and the circulation of IIMs and DMD patients. We speculate that such activation can play a role in modulating muscle regeneration and pulmonary fibrosis. Although the precise molecular mechanisms leading to abnormal activation of wnt/β-catenin signaling in these diseases could not be defined in the current study, the results have demonstrated that wnt/β-catenin transcriptional activity—especially the activity in the circulation—can be used as a marker to indicate the pathological conditions of IIMs and DMD patients. Further studies are required to illuminate the specific roles that wnt/β-catenin signaling plays in IIMs and DMD patients, with the aim of developing improved therapeutic interventions.
Figures and Tables
Fig. 1
Immunofluorescence staining of β-catenin in skeletal muscle of control subjects (Aa–c) and PM (Ad–f), DM (Ag–i), DMD (Aj–l), and NM (Am–o) patients. Western blot results and semiquantitative analysis of β-catenin and GSK-3β in skeletal muscle of PM, DM, DMD, and NM patients and controls (B–D) (data represent mean±SD values from three separate experiments. *p≤0.01). DM: dermatomyositis, DMD: duchenne muscular dystrophy, GSK-3β: glycogen synthase kinase 3-β, NM: nemaline myopathy, PM: polymyositis.
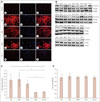
Fig. 2
Western blot results (A) and semiquantitative analysis (B) of GSK-3β phosphorylation in skeletal muscle of PM, DM, DMD patients and normal controls (data represent mean±SD values from three separate experiments. *p≤0.01 between PM patients and controls, *p≤0.01). DM: dermatomyositis, DMD: duchenne muscular dystrophy, GSK-3β: glycogen synthase kinase 3-β.
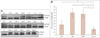
Fig. 3
Results of EMSA for nuclear extracts from skeletal muscle of PM, DM, DMD, NM patients and normal controls (data represent mean±SD values from three separate experiments. *p≤0.01). DM: dermatomyositis, DMD: duchenne muscular dystrophy, EMSA: electrophoretic mobility shift assay, NM: nemaline myopathy, PM: polymyositis.
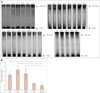
Fig. 4
Relative luciferase activities of the wnt/β-catenin/Tcf pathway reporter constructs 8xTopFlash in HEK293 cells treated with DMEM containing 20% serum from PM, DM, DMD, and NM patients and normal controls (A and B). C: Comparison of relative serum β-catenin/Tcf4 transcriptional activities in PM and DM patients with or without ILD. D: Comparison of relative serum β-catenin/Tcf4 transcriptional activities in PM and DM patients before and after treatment (data represent mean±SD values from three separate experiments. *p≤0.01). DM: dermatomyositis, DMEM: Dulbecco's modified eagle medium, DMD: duchenne muscular dystrophy, PM: polymyositis.
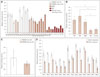
Table 1
Clinical characteristics, pathological profiles and EMSA results of IIMs, DMD, NM, and normal controls
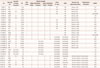
*Integrated intensity of β-catenin/Tcf4 binding activity on EMSA.
AIP: acute interstitial pneumonia, CK: creatine kinase (IU/L), DM: dermatomyositis, DMD: duchenne muscular dystrophy, EMG: electromyography, EMSA: electrophoretic mobility shift assay, F: female, M: male, MP: myopathic pattern, NM: nemaline myopathy, NP: neurogenic pattern, PIF: pulmonary interstitial fibrosis, PM: polymyositis.
References
3. Kalluri M, Oddis CV. Pulmonary manifestations of the idiopathic inflammatory myopathies. Clin Chest Med. 2010; 31:501–512.


4. Monici MC, Aguennouz M, Mazzeo A, Messina C, Vita G. Activation of nuclear factor-kappaB in inflammatory myopathies and Duchenne muscular dystrophy. Neurology. 2003; 60:993–997.


6. MacDonald BT, Tamai K, He X. Wnt/beta-catenin signaling: components, mechanisms, and diseases. Dev Cell. 2009; 17:9–26.
7. Clevers H. Wnt/beta-catenin signaling in development and disease. Cell. 2006; 127:469–480.
8. Lim JC, Kania KD, Wijesuriya H, Chawla S, Sethi JK, Pulaski L, et al. Activation of beta-catenin signalling by GSK-3 inhibition increases p-glycoprotein expression in brain endothelial cells. J Neurochem. 2008; 106:1855–1865.
9. Petropoulos H, Skerjanc IS. Beta-catenin is essential and sufficient for skeletal myogenesis in P19 cells. J Biol Chem. 2002; 277:15393–15399.


10. Fujimaki S, Hidaka R, Asashima M, Takemasa T, Kuwabara T. Wnt protein-mediated satellite cell conversion in adult and aged mice following voluntary wheel running. J Biol Chem. 2014; 289:7399–7412.


11. Tanaka S, Terada K, Nohno T. Canonical Wnt signaling is involved in switching from cell proliferation to myogenic differentiation of mouse myoblast cells. J Mol Signal. 2011; 6:12.


12. Chilosi M, Poletti V, Zamò A, Lestani M, Montagna L, Piccoli P, et al. Aberrant Wnt/beta-catenin pathway activation in idiopathic pulmonary fibrosis. Am J Pathol. 2003; 162:1495–1502.


13. He W, Dai C, Li Y, Zeng G, Monga SP, Liu Y. Wnt/beta-catenin signaling promotes renal interstitial fibrosis. J Am Soc Nephrol. 2009; 20:765–776.
14. Sato M. Upregulation of the Wnt/beta-catenin pathway induced by transforming growth factor-beta in hypertrophic scars and keloids. Acta Derm Venereol. 2006; 86:300–307.


15. Beyer C, Schramm A, Akhmetshina A, Dees C, Kireva T, Gelse K, et al. β-catenin is a central mediator of pro-fibrotic Wnt signaling in systemic sclerosis. Ann Rheum Dis. 2012; 71:761–767.


16. Brack AS, Conboy MJ, Roy S, Lee M, Kuo CJ, Keller C, et al. Increased Wnt signaling during aging alters muscle stem cell fate and increases fibrosis. Science. 2007; 317:807–810.


17. Lévy L, Wei Y, Labalette C, Wu Y, Renard CA, Buendia MA, et al. Acetylation of beta-catenin by p300 regulates beta-catenin-Tcf4 interaction. Mol Cell Biol. 2004; 24:3404–3414.
18. Tews DS. Tumour necrosis factor-mediated cell death pathways do not contribute to muscle fibre death in dystrophinopathies. Acta Neuropathol. 2005; 109:217–225.


19. Ghosh S, Paul A, Sen E. Tumor necrosis factor α-induced hypoxiainducible factor 1α-β-catenin axis regulates major histocompatibility complex class I gene activation through chromatin remodeling. Mol Cell Biol. 2013; 33:2718–2731.


20. Yang CC, Askanas V, Engel WK, Alvarez RB. Immunolocalization of transcription factor NF-kappaB in inclusion-body myositis muscle and at normal human neuromuscular junctions. Neurosci Lett. 1998; 254:77–80.


21. Liu YT, Shang D, Akatsuka S, Ohara H, Dutta KK, Mizushima K, et al. Chronic oxidative stress causes amplification and overexpression of ptprz1 protein tyrosine phosphatase to activate beta-catenin pathway. Am J Pathol. 2007; 171:1978–1988.


22. Banerji CR, Knopp P, Moyle LA, Severini S, Orrell RW, Teschendorff AE, et al. β-Catenin is central to DUX4-driven network rewiring in facioscapulohumeral muscular dystrophy. J R Soc Interface. 2015; 12:20140797.
23. Abu-Baker A, Laganiere J, Gaudet R, Rochefort D, Brais B, Neri C, et al. Lithium chloride attenuates cell death in oculopharyngeal muscular dystrophy by perturbing Wnt/β-catenin pathway. Cell Death Dis. 2013; 4:e821.

